where Hb = hemoglobin concentration, SaO2 = % hemoglobin saturation with oxygen, and PaO2 = partial pressure of dissolved oxygen.
Global oxygen delivery (DO2) to the body depends on this arterial oxygen content (CaO2) as well as cardiac output (CO) :
Global oxygen consumption (VO2) is the total oxygen consumption by all of the body’s organs and tissues. Normal oxygen consumption is ~3 ml/kg/min O2. The amount of oxygen that is returned to the cardiopulmonary circulation from the venous side is termed the mixed venous oxygen saturation (SvO2). The oxygen extraction ratio (O2ER) is defined as oxygen consumption divided by oxygen delivery :
Under normal conditions, the body extracts approximately 30–35 % of the delivered oxygen and the rest is returned to the heart as the mixed venous oxygen. Thus, normal mixed venous oxygen saturation is 65–70 %.


The body is capable of increasing oxygen extraction for brief periods during exercise or stress up to a maximum O2ER of about 70 %. Any further or prolonged increase in oxygen consumption (or decrease in oxygen delivery) will result in cellular hypoxia, anaerobic metabolism, and the production of lactic acid.
Recall from the above arterial oxygen content equation that hemoglobin concentration (Hb) and hemoglobin saturation (SaO2) influence the oxygen content. The relationship between partial pressure of oxygen in the blood and hemoglobin saturation is defined by the oxyhemoglobin dissociation curve (Fig. 29.1). The position of this curve is affected by pH, temperature, PaCO2, and 2,3-diphosphoglycerate (2,3-DPG). Shifting of the curve to the left or right will alter the ability of hemoglobin to bind oxygen. As the curve shifts to the right, hemoglobin has less affinity for oxygen, and thus more oxygen will be released to the tissues. As the curve shifts to the left, hemoglobin binds oxygen more tightly and releases less to the tissues. During periods of stress (such as metabolic acidosis), the curve is shifted to the right to allow more oxygen to be delivered to the tissues.


Figure 29.1
Oxyhemoglobin dissociation curve . This curve defines the relationship between partial pressure of oxygen in the blood and the percent of hemoglobin that is saturated with oxygen. The position of this curve is influenced by factors such as H+ concentration, PaCO2, temperature, and 2-3-diphosphoglycerate (DPG) (Image Courtesy J. Ehrenfeld)
Markers of Oxygen Balance and Tissue Perfusion
Lactate
When the body’s oxygen balance is such that oxygen demand exceeds oxygen supply, cells become hypoxic and convert to anaerobic metabolism. Lactic acid (lactate) is a by-product of anaerobic metabolism and can be measured in the blood. Elevated lactate levels are associated with tissue hypoperfusion and poor oxygenation. Although other factors can affect lactate levels, the presence of elevated lactate can therefore be used as an indirect marker of poor tissue perfusion and shock.
Central Venous and Mixed Venous Blood Oxygen Saturation
When a central venous catheter is in place, blood can be drawn from the superior vena cava (distal port) and sent to the lab for measurement of central venous blood oxygen saturation. Central venous blood oxygen saturation (ScvO2) correlates well with mixed venous blood oxygen saturation (SvO2) in most circumstances, and can be used to reflect tissue oxygenation. Normal ScvO2 is approximately 70 % (compared to normal SvO2 of approximately 65 %). Lower than normal ScvO2 or SvO2 is an indication of poor tissue oxygenation and the need for improved oxygen delivery and perfusion. The advantage of using ScvO2 is that it does not require a pulmonary artery catheter (versus SvO2 which is drawn from the pulmonary artery).
Hemodynamic Monitoring (Also See Chap. 11)
Goals
The goals of hemodynamic monitoring in the critically ill patient are to optimize perfusion and oxygen delivery to tissues, ensure rapid detection of changes in clinical status, and monitor for response to treatment. Although noninvasive monitors (such as a blood pressure cuff) are associated with less risks and complications, it is often necessary to use invasive monitoring techniques to achieve these goals.
Invasive Arterial Blood Pressure Monitoring
A common cause of admission to the intensive care unit is hypotension, which may be due to any number of etiologies (see “Shock” section below). Blood pressure monitoring with a noninvasive cuff may be adequate, but if the blood pressure is significantly low, it may be undetectable or inaccurate by a cuff. In addition to being the most accurate form of blood pressure monitoring, arterial cannulation allows continuous beat-to-beat monitoring. It also serves as a site for obtaining lab measurements of oxygenation, ventilation, and acid–base status. The most common sites for arterial cannulation are radial or femoral arteries, but other arteries may be used if necessary (see Chap. 15, IV, Arterial & Central Line and Gastric Tube Placement Techniques).
Complications associated with arterial cannulation and precautions to decrease the incidence of complications are listed in Table 29.1.
Table 29.1
Complications associated with arterial cannulation
Complication | Precautions to decrease risk |
---|---|
Hematoma | Avoid multiple needle punctures/attempts Apply pressure if artery punctured |
Bleeding | Caution in coagulopathic patients Apply pressure to bleeding site |
Thrombosis | Avoid multiple needle sticks Use continuous flush system Avoid prolonged catheterization |
Vasospasm | Avoid multiple or traumatic punctures/attempts at cannulation |
Air embolism | Caution when flushing catheter |
Nerve damage | Avoid sites in close proximity to nerve |
Infection | Use sterile technique Avoid prolonged catheterization |
Intra-arterial drug injection | Keep venous and arterial lines well-organized, separated, and clearly labeled |
Ischemia | Avoid traumatized sites Avoid prolonged catheterization Place pulse oximeter on ipsilateral side to verify perfusion |
Volume status can be assessed by evaluating the arterial pressure height during controlled mechanical ventilation . Positive pressure ventilation will lead to significant systolic variation (>10 mmHg) of the blood pressure in patients who are hypovolemic (Fig. 29.2).


Figure 29.2
Arterial blood pressure tracing showing systolic pressure variation with three consecutive incremental breaths (10, 20, 30 cm H2O) during changes in mechanical ventilation. The line of best fit connects the three respective lowest systolic blood pressures (Used with permission. From Functional Hemodynamic Monitoring by Michael R. Pinsky, Didier Payen. Springer, 2004)
Cardiac Output
Recall that global oxygen delivery (DO2) to the tissues is dependent on the oxygen content of blood (CaO2) as well as cardiac output (CO) . Cardiac output is equal to the product of heart rate (HR) and stroke volume (SV):
The variables that affect stroke volume include preload, afterload, and contractility. Preload is an estimate of left ventricular volume at the end of diastole. The Frank-Starling curve shows the relationship between preload and stroke volume (Fig. 29.3). In general, increases in preload lead to greater stroke volume. However, a point on the Frank-Starling curve is eventually reached where further increases in preload do not increase stroke volume and may instead lead to decreased stroke volume (as in congestive heart failure). Because it is difficult to measure ventricular volume, ventricular pressure is commonly used to estimate volume and thus preload. Use of a central venous catheter enables monitoring of right atrial pressure or central venous pressure (CVP), which is an estimate of right ventricular preload. In a patient without significant pulmonary hypertension or valvular disease, it can be assumed that right ventricular preload correlates with left ventricular preload because the same blood volume that enters the right heart will traverse to enter the left heart. By way of this assumption, CVP is often used as an estimate of left ventricular preload.



Figure 29.3
Frank-Starling curve . This curve shows the relationship between preload (end-diastolic volume) and stroke volume. Increases in preload lead to increased stroke volume until a point is reached where further increases in end-diastolic volume lead to congestive heart failure (point B to point A) (Used with permission. From Pediatric Critical Care Medicine: Basic Science and Clinical Evidence. Derek S. Wheeler, Hector R. Wong, and Thomas P. Shanley, editors. Springer, 2007)
Afterload refers to the myocardial wall tension that is required to overcome the opposing resistance to blood ejection. Right ventricular afterload is clinically represented by the pulmonary vascular resistance (PVR) and left ventricular afterload is clinically represented by the systemic vascular resistance (SVR). SVR may be calculated from the following equation when cardiac output measurements are obtained:
where MAP = mean arterial pressure.
![$$ \mathrm{S}\mathrm{V}\mathrm{R} = \left[\left(\mathrm{MAP} - \mathrm{C}\mathrm{V}\mathrm{P}\right)/\mathrm{C}\mathrm{O}\right] \times 80 $$](/wp-content/uploads/2016/09/A161280_2_En_29_Chapter_Eque.gif)
Contractility refers to the ability of the myocardium to contract and eject blood from the ventricle. Contractility depends on preload and afterload so these variables should be optimized first in order to improve contractility. Contractility can be directly measured with the use of echocardiography to estimate ejection fraction. However, once preload and afterload are optimized, contractility is often indirectly represented by cardiac output. If cardiac output remains low despite improvements in preload and afterload, the use of inotropic pharmacologic agents may be initiated to improve contractility.
Central Venous Pressure Monitoring
As described above, invasive CVP monitoring allows continuous measurement of right heart pressures, which can be used to reflect preload. Normal CVP during positive pressure ventilation ranges from 6 to 12 mmHg. A low CVP with hypotension and tachycardia most often corresponds to hypovolemia. Persistent hypotension following a fluid challenge and higher than normal CVP indicates cardiac congestion (as may occur with cardiac tamponade, tension pneumothorax, or myocardial ischemia).
Cannulation sites for CVP placement include subclavian, internal jugular, and femoral veins (also see Chap. 15, IV, Arterial & Central Line and Gastric Tube Placement Techniques). Complications associated with the placement of a central line are presented in Table 29.2. To reduce the number of cannulation attempts and the risk of inadvertent arterial puncture, direct visualization with ultrasound guidance should be used when possible during placement of a central line.
Table 29.2
Complications associated with central venous and pulmonary artery catheterization
Complication | Precautions to decrease risk |
---|---|
Hematoma | Avoid multiple needle punctures/attempts Apply pressure if vein or nearby artery punctured |
Bleeding/hemorrhage | Caution in coagulopathic patients Apply pressure to bleeding site |
Air or thrombotic embolism | Caution with infusions Use head-down tilt and avoid open catheter to air Avoid prolonged catheterization and use continuous flush |
Carotid artery puncture/cannulation | Use appropriate landmarks ± sonographic visualization Use small finder needle; transduce pressure to verify venous |
Pneumothorax/hemothoraxa | Use appropriate landmarks Avoid multiple needle sticks No risk with femoral vein Risk with internal jugular < risk with subclavian |
Infection/bacteremia/endocarditis | Use strict sterile techniqueb Avoid prolonged catheterization |
Nerve trauma | Use appropriate landmarks and avoid sites in close proximity to nerves |
Thoracic duct damage/chylothorax | Avoid left subclavian and internal jugular when possible |
Complete heart block | Extreme caution placing PAC in patient with LBBB |
Cardiac dysrhythmias | Use ECG monitoring while placing catheter and avoid prolonged placement of wire/catheter in atria/ventricles |
Pulmonary ischemia/infarction | Do not keep PAC continuously wedged Minimize balloon inflation time |
Pulmonary artery rupture Myocardial perforation | Do not over-wedge PAC; avoid balloon hyperinflation Always inflate balloon before advancing catheter, but never inflate balloon against significant resistance Always deflate balloon before withdrawing catheter |
Pulmonary Artery Catheter
As described above, left heart pressures may be estimated from right heart pressures in most circumstances and CVP may be used to approximate pulmonary capillary wedge pressure (PCWP). However, when left ventricular function is impaired, or significant valvular disease or pulmonary hypertension is present, the use of a pulmonary artery catheter (PAC) may be indicated for more accurate estimations of left heart pressures. Use of a PAC allows continuous monitoring of pulmonary artery pressures, intermittent monitoring of PCWP, and thermodilution for estimation of cardiac output and calculation of systemic vascular resistance. PCWP is used as the best estimation of left ventricular end-diastolic volume (preload), analogous to CVP estimation for the right ventricle.
The PAC can also be used to obtain blood samples for mixed venous oxygen saturation (SvO2) in order to evaluate oxygen balance. Risks associated with PAC placement include those associated with central line placement as well as additional risks (Table 29.2). Table 29.4 in the next section shows how the use of a PAC can help in the determination of common hemodynamic disturbances in shock.
Table 29.4
Hemodynamic disturbances in shock
Shock type | Central venous pressure or pulmonary capillary wedge pressure | Cardiac output | Systemic vascular resistance |
---|---|---|---|
Hypovolemic | Decreased | Decreased | Increased |
Cardiogenic | Increased | Decreased | Increased |
Distributive | Depends on volume status (initially decreased) | Normal or increased | Decreased |
Obstructive | Increased | Decreased | Increased |
Shock
Shock is a disorder of impaired tissue perfusion and results when oxygen delivery is inadequate to meet the demands of oxygen consumption or when tissues are unable to adequately utilize delivered oxygen. Hypotension is often present in shock, but shock can also occur without hypotension due to compensatory mechanisms that serve to augment blood pressure. Many other clinical signs of shock may be present, including altered mental status, organ dysfunction such as low urine output, cold extremities, acidosis, tachycardia, tachypnea, and any other sign of impaired perfusion. If not rapidly treated, shock can lead to irreversible tissue injury, organ failure, and death.
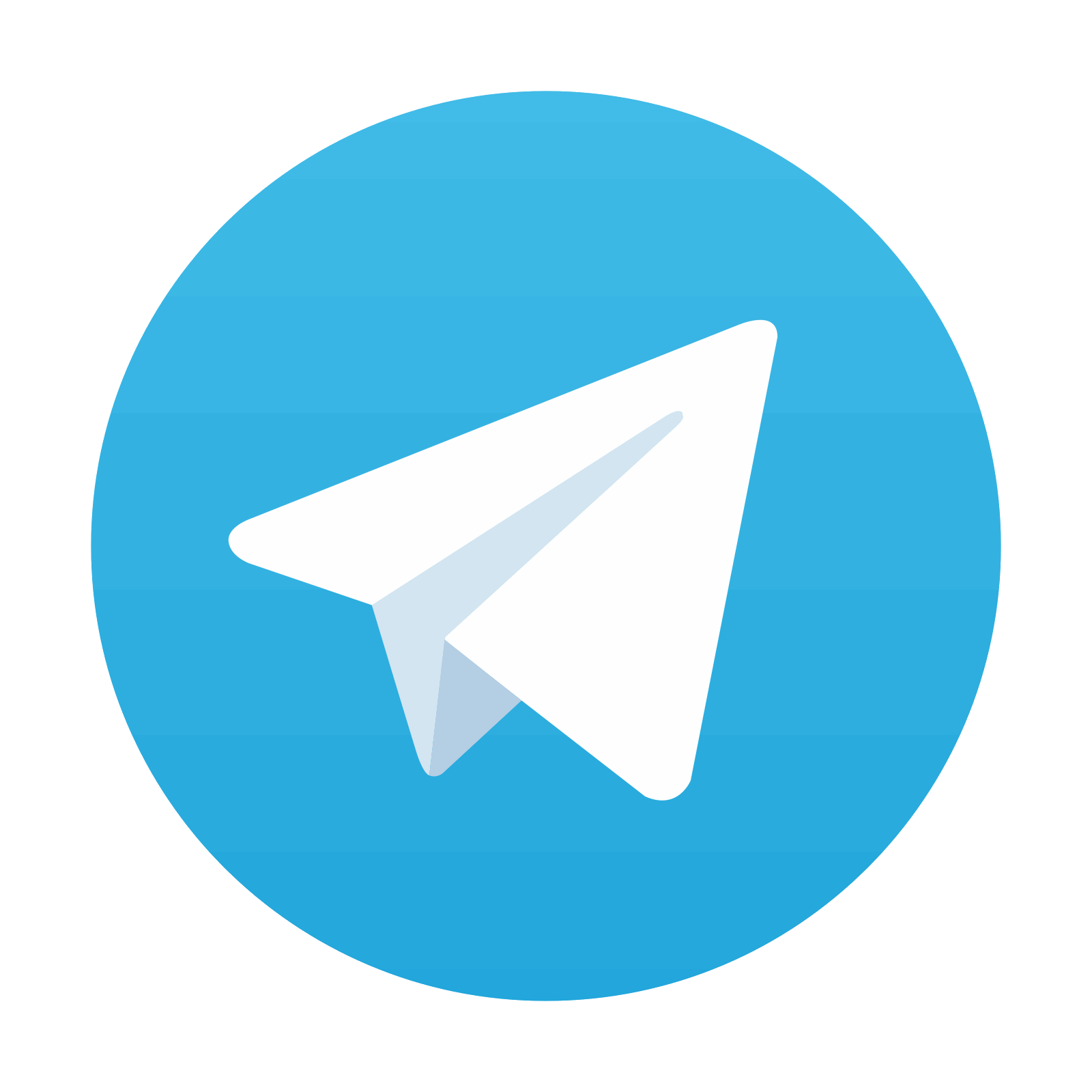
Stay updated, free articles. Join our Telegram channel

Full access? Get Clinical Tree
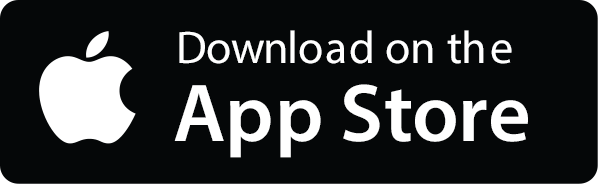
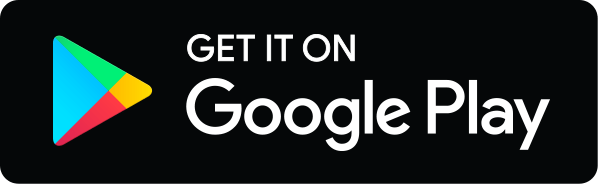