Chapter Outline
Fluid Delivery Systems for Resuscitation 218
Infusion Devices 218
Background 218
IV Catheters 220
Rapid Infusion Catheters 220
Intraosseous Needles 222
IV Tubing Sets 224
Air Embolism 226
Filtration of Blood Products 228
Fluid Warmers 229
Background: Intraoperative Hypothermia 229
Overview of Fluid Warming Systems 230
Simple Fluid Warmers 230
Resuscitation Fluid Warmers 231
Cell Salvage Systems 231
Overview 231
Contraindications to Cell Salvage 233
Equipment for Cell Salvage 233
Fluid Delivery Systems for Resuscitation
Infusion Devices
Background
Determinants of Fluid Flow Rate
Numerous factors determine the flow rate of IV fluids, including characteristics of the fluid itself, characteristics of the IV circuit that are extrinsic to the patient, and patient-driven determinants of the driving pressure for fluid flow ( Figure 16–1 ).

Characteristics of the infusate that affect flow rate include its viscosity and temperature, its potential to form air locks or overwhelm closed air filters (with air derived either from the fluid source or from spontaneous bubble formation as a cold solution spontaneously warms within the IV circuit), or its potential to occlude the administration set with particulate matter (such as clot, emulsifiers, or precipitates resulting from incompatible infusates) ( Table 16–1 ).
Mechanism of Occlusion | Examples |
---|---|
Clot formation | Calcium-containing solutions (lactated Ringer’s, Hetastarch) with blood products |
Precipitate formation | Thiopental with vecuronium/pancuronium; heparin, amiodarone, and numerous antibiotics with a variety of anesthesia drugs |
Bicarbonate with calcium/many local anesthetics | |
Clogged filter | Any of the above; air; emulsifiers such as those in propofol, intralipid |
Features of the IV circuit that affect fluid flow include the inner diameter of the IV catheter, tubing, injection ports and Luer- locks, the length of each circuit component (particularly the narrowest components), the distensibility of the tubing and its propensity to kink, whether the fluid delivery system is pressurized, and the vertical distance between the fluid source and the target vein. When multiple fluid sources funnel into the same IV catheter, the carrier flow rate, the relative pressurization of each fluid source, and the competency of any one-way valves at the convergence of two fluid streams can also profoundly affect the forward flow rate for each infusate. Lastly, fluid flow rate also reflects patient-specific factors such as the back-pressure in the cannulated vein (intrinsic or extrinsic [i.e., from a noninvasive blood pressure cuff]) and the pressure gradient between the vein containing the IV cannula and the right atrium.
Determinants of Drug Delivery
Drug delivery through an IV administration set is a function of the concentration of the stock solution of drug given as an infusion through a piggyback into a carrier infusion, and the flow rates of both infusions ( Figure 16–2 ). Another important factor is the size of the dead space volume (V) that lies between the convergence of the carrier and drug infusions and the tip of the IV cannula. To avoid fluctuations in drug delivery, the carrier flow rate within IV tubing should be kept constant and the dead space of the infusion system should be minimized. Avoid using concentrated solutions of potent drugs to minimize the risk of complications if carrier flow changes abruptly.

Experimental and mathematical models demonstrate that it takes 1 to 3 time constants for the drug concentration volume (V) throughout the dead space to reach a new steady state after a change in carrier or drug flow rate. The time required for a new drug infusion to reach the patient at the desired concentration also varies significantly, depending on the dead space volume of the side port that must be primed.
Characterizing Fluid Flow Within the IV Circuit
The impact of length and inner diameter on fluid flow rates (through both catheters and IV cannulas) has long been appreciated. Historically, fluid flow through IV catheters and tubing was considered laminar (and therefore has been described by the Poiseuille formula) ( Figure 16–3 , A ). However, the Poiseuille formula does not fully describe pressure loss for fluid flow through IV cannulas and IV tubing. Laminar flow through an IV catheter is prevented by the short length and abrupt decrements in tubing diameter (i.e., where the IV administration set meets the catheter), which create eddies.

In reality, flow through IV tubing and catheters has both laminar and turbulent characteristics. Accordingly, the prevailing model for flow through IV catheters and infusion systems incorporates both types of flow by using a quadratic equation ( Figure 16–3 , B ) to describe the relationship between radius and flow, rather than Poiseuille’s quadratic relationship. For IV catheters, the laminar component of pressure loss (which has a linear pressure-flow relationship) is consistent with what the Poiseuille formula would predict, while the turbulent component reflects pressure loss due to flow disturbances at the inlet, and where the catheter changes shape. For IV tubing, there is an additional component of turbulent-flow pressure loss that is proportional to tubing length. Recent research indicates that while radius is the most powerful determinant of flow rate through an IV catheter, the effect of changing radius on flow rates through 14- to 20-gauge IV catheters is less than commonly believed.
Regulations from the International Organization for Standardization require the disclosure of maximal IV flow rates through all catheters. These quoted rates describe the flow through a perfectly straight cannula into an open receptacle, from an IV fluid source located 100 cm above the receptacle. While these idealized conditions approximate neither conditions in the operating room nor the maximum flow rates obtained clinically, the quoted rates are helpful for making comparisons among various IV catheters.
IV Catheters
Overview
IV cannulation devices consist of a tapered catheter threaded over a hollow-bore needle. Most are latex-free, radio-opaque, and nonpyrogenic. While no venipuncture system can eliminate the risk of unintended needlesticks, self-retracting safety systems eliminate the risk of needlesticks after a needle is withdrawn from its catheter.
Because of its comparatively shorter length (and lower resistance), a large-bore, well-functioning peripheral IV is considered superior to a central venous catheter for large volume fluid resuscitation. For example, as highlighted in Table 16–2 , manufacturer-quoted maximum flow rates are significantly lower for central venous catheters, as compared with peripheral IV catheters with the same inner diameter. This difference is fivefold when comparing the flow through a 16-gauge peripheral IV (approximately 330 mL/min) to that through the 16-gauge proximal port of a triple lumen central line (63 mL/min) ( Table 16–2 ).
Gauge | Inner Diameter (mm) | Length | Manufacturer-Quoted Flow (mL/min) |
---|---|---|---|
|
| 50 mm |
|
|
| 50 mm | 210 |
|
| 45 mm | 100 |
|
| 32 mm |
|
| 0.9 mm | 25 mm |
|
| 0.7 mm |
|
|
7F Triple-lumen arrow central catheter for use only with 8.5 or 9F percutaneous sheath introducer (PSI) | |||
16 (proximal) | 140 mm | 76 | |
18 (medial) | 35 | ||
18 (distal) | 36 | ||
7F Triple-lumen arrow central catheter for use only with MAC two-lumen central venous access device | |||
16 (proximal) | 63 | ||
18 (medial) | 26 | ||
18 (distal) | 25 |
Other Features of IV Cannulation Systems
Other important design features include the conformation of the orifice on the needle tip and the distance between this orifice and the catheter tip (see Figure 16–5). A flash of blood appears (whose location may vary, see Figure 16–4 ) when the tip of the needle orifice enters the vessel lumen; the needle must then be advanced further to project the catheter tip into the vessel lumen before the catheter can be threaded. Because unfamiliarity with these product-specific spatial relationships can predispose one to transect or tear a vein during attempted cannulation, it is useful to become facile with a variety of IV cannulation systems.

Other product-specific features also affect the cannulation process ( Figure 16–5 ). These include the needle penetration force required (which can range from 50 to 150 g) during cannulation (which is largely determined by the needle bevel), the type of hub used to advance the catheter, and the degree to which the catheter tapers (which affects catheter penetration force, a force that typically exceeds needle penetration force by onefold to twofold). Material memory can cause severe kinks to persist, even after extrinsic forces are removed.

Rapid Infusion Catheters
Rationale
The rapid infusion catheter is designed to replace an in situ 20-gauge (or larger) peripheral IV catheter, to facilitate large volume rapid infusions ( Figure 16–6 ). RIC lines allow for more rapid infusion rates as compared to central and conventional peripheral catheters, owing to their larger diameter and shorter length. Relative to central lines, RIC lines can be easier or faster to place (and even have a role in pediatrics), although central access can be easier to obtain and more reliable when peripheral veins are small or friable. Because of the dire consequences of intra-arterial infusion of air, pharmacologic agents or fluids (especially under pressure), it is critical to confirm that the RIC is intravenous before using it.

Technique
To place a RIC, the guidewire is advanced through an in situ IV catheter (with limited force, to avoid damaging the vein), and the preexisting IV catheter is removed. Next, the skin opening is enlarged, by advancing the scalpel away from the guidewire, carefully to avoid cutting the guidewire or creating a skin bridge. The dilator is then threaded over the guidewire, grasped close to the skin incision, and advanced through the skin and subcutaneous tissues into the vein with a slight twisting motion. Finally, the dilator is removed, and the infusion catheter is inserted, connected to IV tubing, and sutured into place.
To achieve maximal flow rates with the RIC, standard-gauge stopcocks and any small-diameter or unnecessary lengths of IV tubing should be removed from the IV administration set. The maximal flow rate through a RIC doubles when IV tubing with a large inner diameter is used.
Intraosseous Needles
Rationale
Sometimes intravenous access is impossible. However all patients have long bones, and the bone marrow space is connected to the circulation. In fact, the bone marrow space, or intraosseous compartment, is considered to be connected to the central circulation and is suitable for administration of fluids for resuscitation and potent vasopressors.
Intraosseous cannulation has been described using various needles, typically as a technique of last resort. Pain on insertion, pain on injection, and concerns about osteomyelitis have limited use of the technique in the past. Recently available commercial kits and changes in technique have effectively addressed most of these concerns these obstacles.
Technique
A commercially available intraosseous access kit is illustrated in Figure 16–7 . The kit contains all of the items needed to make the technique safe and relatively painless. The skin over the selected bony prominence should be disinfected with a germicidal, persistent solution, such as chlorhexidine in alcohol (shown as part of the kit in Figure 16–7 ). Local anesthesia is provided at the skin puncture site. The appropriate length of needle is chosen; typically only one diameter is available. The needle is inserted with a powered driver, shown in Figure 16–8 . Use of a powered driver to spin the needle shortens the duration of painful stimulus, reduces the insertion force required, and adds an element of control in placement. Pain from injection is alleviated by first giving a dose of local anesthetic such as lidocaine. After lidocaine administration, the needle should be flushed under pressure, with one or more 10-mL syringes of sterile normal saline to establish flow.


Confirmation of successful insertion hinges on a constellation of “soft” findings, including a firmly seated needle, potential (but not certain) ability to aspirate blood from the needle, easy flow of fluids under pressure, and expected responses to pharmacological agents. Because the intramedullary pressure is higher than venous pressure (typically 35 to 50 mm Hg), fluids must be given under pressure to achieve high flow rates.
Intraosseous administration provides quick access to the circulation and should be considered when access is difficult. This is particularly true when difficulty obtaining intravenous access is delaying administration of resuscitation drugs. Even in elective settings, the intraosseous route may be preferable to repeated attempts at starting a peripheral IV. Common insertion sites include the distal and proximal tibia and the proximal humerus. All of these have the key feature of being palpable even in obesity, and well removed from any major nerves or blood vessels.
Contraindications include: infection at the potential insertion site, fracture of the target bone, and recent procedures involving the target bone.
IV Tubing Sets
Overview
Simple fluid administration sets typically consist of a dripper, a drip chamber, a rolling side-clamp to adjust flow, sliding clamps to occlude flow, and, typically, some kind of injection port ( Figure 16–9 , A ). Manufacturer-quoted drop size (often 15 gtt/mL for adult sets, 60 gtt/mL for pediatric sets) describes the flow rate of a standard crystalloid infusate at room temperature. The internal diameter of tubing typically ranges from approximately 0.1 to 0.3 mm. Many sets also contain an air filter (typically capable of sequestering less than 5 mL of air).

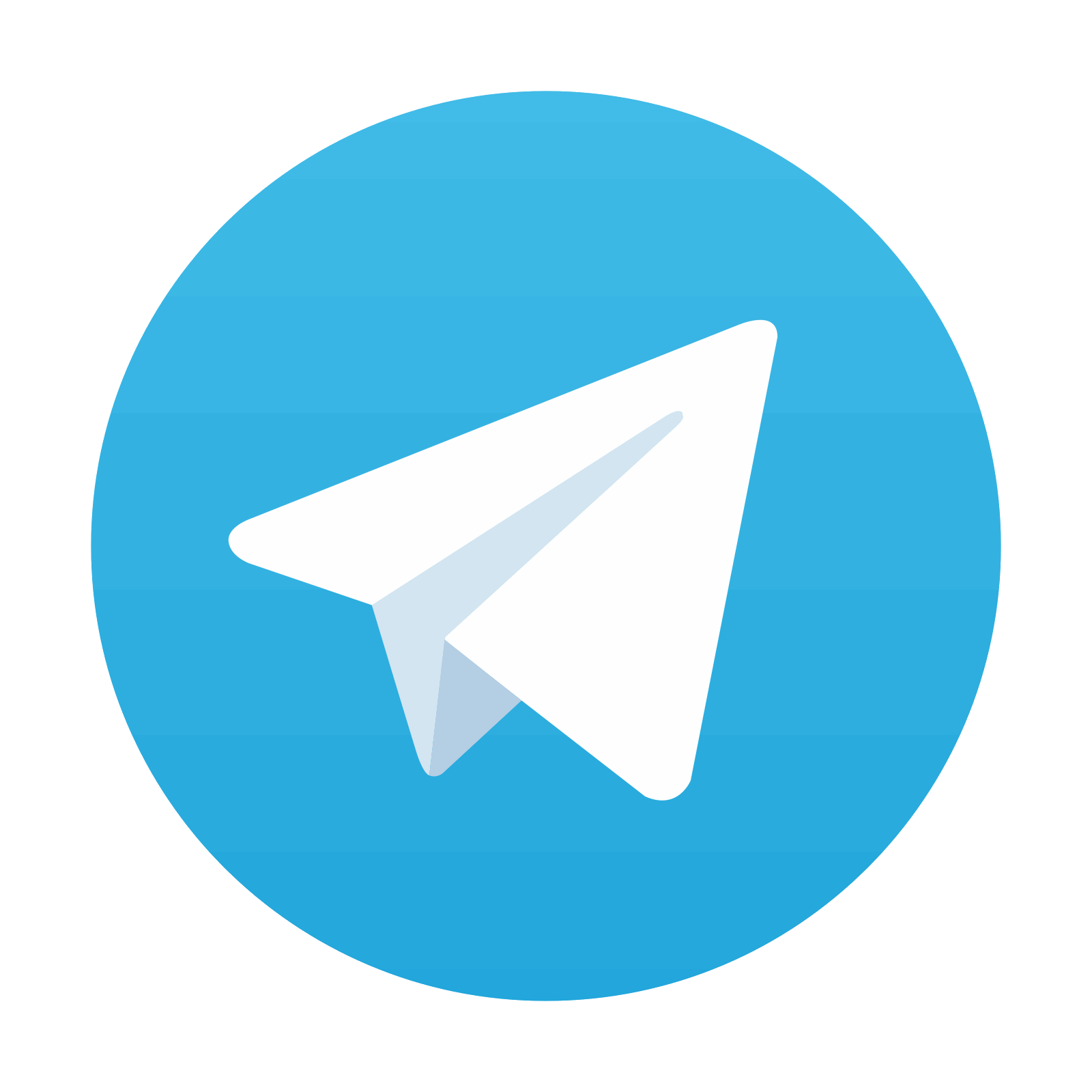
Stay updated, free articles. Join our Telegram channel

Full access? Get Clinical Tree
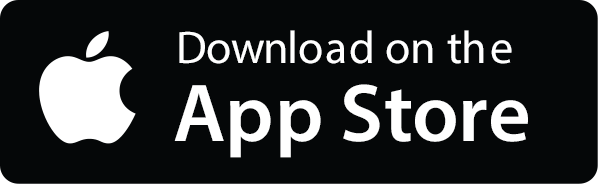
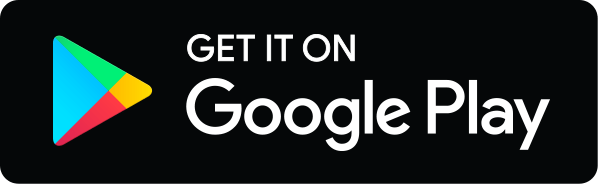
