Lung transplantation is a high-risk procedure that requires a highly trained cardiothoracic anesthesiologist and a considerable degree of vigilance. In the 50 years since the first lung transplantation, improvements in immunosuppression, preservation solutions, and surgical techniques and technologies have led to increased survival rates. The development of the extracorporeal circulatory membrane oxygenation allowed for bridge to transplantation and for donor organ recovery from primary graft dysfunction post transplantation [1]. In addition, changes in the criteria for lung allocation will cause the anesthesiologist to encounter older recipients with comorbidities that would have been disqualifying for transplantation a decade ago [2].
Introduction
The first lung transplantation was performed in 1963 by Dr. Hardy in Mississippi. The recipient was a patient with lung cancer and survived 18 days post transplantation . In Toronto, Dr. Cooper performed the first successful long-term single lung transplantation in 1983 in a patient with pulmonary fibrosis. The recipient was alive and well 26 months post transplantation .
The recipient’s lung pathology determines whether one or two donor lungs are needed. Patients with COPD/emphysema or pulmonary fibrosis may receive a single or a double lung transplantation. Bilateral lung transplantation is required in candidates with suppurative lung disease (cystic fibrosis and bronchiectasis) or pulmonary hypertension (PHN) . If severe uncorrectable heart disease is combined with the lung condition, heart–lung transplantation is necessary.
Previous studies have supported that bilateral lung transplantation provides longer survival than single lung transplantation . Neurohr et al. reported that in patients with idiopathic pulmonary fibrosis (IPF), single lung transplantation provides acceptable survival rates, but selected patients benefit significantly when receiving a double lung transplantation . They suggested further investigation for possible confounders, such as PHN . Schaffer et al. concluded that since the application of the lung allocation score, IPF patients achieved better organ survival when they received a double lung transplantation . Interestingly, in patients with COPD, there was no difference in the 5-year survival rate between single and double lung transplantation .
Although there are many case reports in the literature with great results despite the prolonged ischemic graft time, even more than 11 h, a multicenter analysis by Thabut et al. demonstrated a correlation between the graft ischemic time and (a) the early gas exchange and (b) the long-term survival . In addition, there was an increased risk of death when the time exceeded 6 h .
Common complications of lung transplantation include primary graft dysfunction (primarily related to ischemia-reperfusion injury), acute rejection, airway complications (stenosis, dehiscence), infections, and bronchiolitis obliterans (a fibroproliferative disorder that leads to chronic allograft dysfunction) .
In addition, as recipients presenting for lung transplantation are older and with more comorbidities than previous years, their overall treatment becomes more challenging .
Preoperative evaluation
Performing a thorough history and physical examination is essential because the patients’ status often changes from the time of their latest evaluation. Worsening of exercise tolerance and increased oxygen requirements indicate the progression of the disease. Pulmonary function tests (PFTs) demonstrate the severity of the patients’ conditions, but the latest available PFT results at the time of the transplant may not reflect the current stage of the disease.
One can indirectly obtain information about the patients’ conditions by reviewing the recent laboratory results. Increased bicarbonate may imply carbon dioxide retention (contraction alkalosis secondary to diuretics must be excluded). High hemoglobin levels may suggest hypoxemia or dehydration (electrolyte and uric acid levels may be helpful in differentiating). High INR may reflect liver dysfunction related to right heart failure. Low albumin levels may be a prognostic indicator and should be considered when dosing highly albumin-bound medications.
The airway history and examination will determine the intubation method. History of previous difficult intubation and/or a difficult airway upon evaluation may suggest the need for additional instruments, such as a fiber optic bronchoscope and video laryngoscope. All equipment needed for intubation should be present and checked for proper function before the patients enter the operating room. These patients may decompensate quickly, and no time should be wasted for this purpose. The NPO status will determine whether rapid sequence induction will be performed.
The electrocardiogram may show right bundle brunch block and increased P wave, findings that represent dilated/hypertrophic right cardiac cavities. The echocardiogram provides considerable information about the patients’ pulmonary arterial pressures, right and left heart function, presence of patent foramen ovale, valvulopathies, and the presence of pericardial effusion. These data may determine whether cardiopulmonary bypass (CPB) or extracorporeal circulatory membrane oxygenation (ECMO) will be initiated sooner than later in the case. The right heart catheterization confirms the pulmonary pressure information. Not everyone requires a left heart catheterization. This depends on the patients’ risk factors for cardiac disease and the institution’s protocol.
Preoperative evaluation
Performing a thorough history and physical examination is essential because the patients’ status often changes from the time of their latest evaluation. Worsening of exercise tolerance and increased oxygen requirements indicate the progression of the disease. Pulmonary function tests (PFTs) demonstrate the severity of the patients’ conditions, but the latest available PFT results at the time of the transplant may not reflect the current stage of the disease.
One can indirectly obtain information about the patients’ conditions by reviewing the recent laboratory results. Increased bicarbonate may imply carbon dioxide retention (contraction alkalosis secondary to diuretics must be excluded). High hemoglobin levels may suggest hypoxemia or dehydration (electrolyte and uric acid levels may be helpful in differentiating). High INR may reflect liver dysfunction related to right heart failure. Low albumin levels may be a prognostic indicator and should be considered when dosing highly albumin-bound medications.
The airway history and examination will determine the intubation method. History of previous difficult intubation and/or a difficult airway upon evaluation may suggest the need for additional instruments, such as a fiber optic bronchoscope and video laryngoscope. All equipment needed for intubation should be present and checked for proper function before the patients enter the operating room. These patients may decompensate quickly, and no time should be wasted for this purpose. The NPO status will determine whether rapid sequence induction will be performed.
The electrocardiogram may show right bundle brunch block and increased P wave, findings that represent dilated/hypertrophic right cardiac cavities. The echocardiogram provides considerable information about the patients’ pulmonary arterial pressures, right and left heart function, presence of patent foramen ovale, valvulopathies, and the presence of pericardial effusion. These data may determine whether cardiopulmonary bypass (CPB) or extracorporeal circulatory membrane oxygenation (ECMO) will be initiated sooner than later in the case. The right heart catheterization confirms the pulmonary pressure information. Not everyone requires a left heart catheterization. This depends on the patients’ risk factors for cardiac disease and the institution’s protocol.
Preparation
To minimize ischemic graft time, it is imperative that all equipment and medications required for the procedure are readily available before entering the operating room. Placement of an arterial line before acceptance of the organ remains at the discretion of the anesthesiologist as early placement may avoid delays.
It is important to verify that all antibiotics and immunosuppressive medications have been ordered and are available to be given according to the protocol. Some of these have to be given orally in the preoperative area.
Often the organ acceptance time changes, and the patients remain in the preoperative area for longer than initially planned. It is essential to continue their routine bronchodilators and other key home medications necessary to support their baseline pulmonary status. Some patients may require additional oxygen support because of their higher anxiety levels.
Preoperative sedatives or narcotics should be administered cautiously. Minimal suppression of the respiratory system in end-stage lung disease patients may be detrimental. On the contrary, anxiety may worsen breathing and increase the pulmonary pressures . Administration of such medications should be in small dosages and under strict supervision.
A thoracic epidural catheter may be placed preoperatively. These patients should be carefully selected and considered to be at low need for extracorporeal life support (ECLS) during the surgery. Unplanned conversion to mechanical support, a bloody tap, and time delays, which lead to increased organ ischemic time, are only some of the concerns with placing neuraxial catheters . Many are reluctant to perform this procedure, as the risk of a belatedly diagnosed epidural hematoma is a devastating complication .
Nerve cryoablation has been discussed by some but it needs to be further explored. Pain control procedures may be performed, if necessary, post-operatively in the ICU when the patients are awake, under more controlled conditions.
Monitors
Although there are no consensus guidelines regarding intraoperative hemodynamic monitoring, most centers routinely use arterial line, pulmonary arterial catheter (PAC), and transesophageal echocardiography (TEE) , in addition to all standard monitors. The arterial line should be present before induction as close monitoring of the blood pressure and immediate intervention are imperative. In addition to the radial arterial line, some centers place a femoral arterial line after intubation. Application of external defibrillator pads varies according to the situation. Utilization of cerebral oximetry and technologies to assess the depth of anesthesia differs between institutions.
A PAC is inserted usually after intubation. In patients with severe PHN and compromised right heart function, some may prefer to place the PAC pre induction if the patients can tolerate the supine position. A PAC with continuous cardiac output and continuous mixed venous monitoring may be useful.
Echocardiographic monitoring allows the anesthesiologist to detect changes in right heart function and size, worsening of tricuspid regurgitation, and filling status of the left ventricle. This information becomes important in deciding if ECLS is necessary.
The presence of air in the left atrium during reperfusion may lead to air embolism-related left ventricular regional wall function deterioration that can be detected by TEE. Usually, this condition is associated with ST changes on the EKG.
Obstruction of the pulmonary vein flow (due to clot, kinking, or narrow anastomosis) may present with hypoxia. TEE becomes an important tool in differentiating this condition from other causes such as acute graft rejection or reperfusion injury. Turbulence of flow, pulmonary vein diameter of <0.5 cm, peak systolic flow velocity >1 m/s, and pulmonary vein-left atrial pressure gradient ≥10–12 mmHg support the diagnosis of pulmonary vein stenosis . A diameter less than 0.25 cm and velocity 1.6 m/s may lead to graft failure . Detection of previously unrecognized patent foramen ovale might also contribute to the development of hypoxia. TEE may be used to calculate hemodynamic parameters such as cardiac output and pulmonary pressures.
Monitoring body temperature and avoiding hypothermia are essential to prevent increases in pulmonary vascular resistance (PVR). It also has a considerable effect on the coagulation system when moderate-to-severe acidosis is present . Hypothermia has also been associated with increased bleeding, infections, and prolonged drug effects .
Arterial blood gases should be performed regularly for early detection of changes in acid–base, electrolyte, hematocrit, carbon dioxide (CO 2 ) and oxygen (O 2 ) levels and for providing appropriate adjustments. These patients have abnormal ventilation/perfusion (V/Q) and CO 2 gradients, and the end-tidal CO 2 does not accurately represent the PaCO 2 levels.
Close monitoring of fluid administration and urine output is mandatory. In general, large volumes of fluids should be avoided, as the donor lung is susceptible to edema . In addition, colloids may adversely affect early graft function .
Intraoperative management
Prior to induction
It is crucial to confirm that the patients have taken all the oral immunosuppressive medications according to the protocol. The stomach should not be suctioned before placing the TEE probe after intubation to allow absorption of oral medications. All intraoperative immunosuppressive medications and antibiotics should be administered on time. The immunosuppression and antibiotic protocols are subject to occasional change and vary in different practices.
PHN and right heart dysfunction are the main concerns when anesthetizing patients undergoing lung transplantation. PHN, right ventricular hypokinesis, and leftward shift of the interventricular septum due to the high right-sided pressures are associated with low left ventricular preload and low stroke volume.
Hypoxemia, hypercarbia, acidosis, anxiety, hypothermia, and sympathetic stimulation increase PVR and thus increase right ventricular afterload, which may lead to acute right heart decompensation, hypotension, coronary hypoperfusion, and cardiac arrest.
Utilization of 100% FiO 2 is usually necessary to maintain optimal oxygenation during the surgery. PaCO 2 30–35 mmHg and pH 7.4–7.45 (with the use of bicarbonate) have been described to be beneficial in patients with PHN , but one should consider the whole picture of the patients’ conditions as these patients present with different types of lung pathophysiology. It is important to consider the risks and benefits involved in trying to achieve this goal.
In many of these patients, it is very difficult of eliminate CO 2 , and administration of bicarbonate may further increase its level. In addition, exaggerated maneuvers to increase minute ventilation may be harmful and lead to pneumothorax or hemodynamic compromise.
Before intubation, a bronchodilator is administered in patients who are on treatment for bronchospasm. Inhalation of iloprost (nebulizer) or nitric oxide (NO) (during mask ventilation) by patients with severe PHN may attenuate pulmonary vasospasm during intubation. Iloprost may cause vasodilation and hypotension and should be used cautiously in patients who are hemodynamically compromised.
Allowing ample time for preoxygenation is important. As mentioned earlier, patients with end-stage lung disease have small respiratory reserve and tend to desaturate quickly. Often, the patients need to be in a semi-sitting or sitting position, as they may become severely dyspneic in the supine position.
Induction of anesthesia
Usually, patients scheduled to undergo lung transplantation are nil per os (NPO) for many hours as they are notified early during the process, when an organ is offered. Performance of rapid sequence, “modified rapid sequence” (mask ventilation with cricoid pressure), or awake intubation depends on the NPO status, the airway exam, and the risk for aspiration. An anesthesiologist has to consider that drugs given in large boluses during rapid sequence induction may cause hemodynamic instability and disastrous outcomes.
Induction is a crucial part of the surgery. The surgeon and the perfusionist should be present and ready to emergently initiate ECMO or CPB in the case of hemodynamic or respiratory instability . Occasionally, the team may decide to place the patients on ECMO before anesthesia induction. Usually, this is required for patients with high noninvasive respiratory support and severe PHN. Early access of the groin vessels before induction may expedite the initiation of ECMO or CPB after induction. Patients placed on veno-venous ECMO must be hemodynamically stable, while veno-arterial ECMO provides hemodynamic and respiratory support.
Optimization of right ventricular preload, right ventricular support and efforts to prevent increases in right ventricular afterload is key to a safe anesthesia induction.
Anesthetic-related suppression of the sympathetic system, vasodilation, and myocardial depression in combination with positive pressure ventilation may cause severe hypotension and cardiac arrest . Conversely, sympathetic stimulation during intubation, light anesthesia, hypoxia, hypercapnia, and acidosis may cause increase in pulmonary pressure and acute right heart collapse.
Rhythm and the extremes of heart rate may contribute to significant changes in cardiac output. Hypotension and decreased perfusion of the right ventricle can lead to myocardial ischemia, arrhythmias, and irreversible hemodynamic instability .
Airway management
In patients with copious secretions, initial intubation is usually performed using a single lumen tube to allow the use of a large bronchoscope and thorough suctioning.
In patients with a difficult airway, a single lumen tube may be used initially (easier to place) that can then be replaced with a left-sided double lumen tube (DLT) by using a tube exchanger. This technique may prevent episodes of hypoxia and hypercarbia. The position must be confirmed using a bronchoscope. Left-sided DLTs are preferred over right-sided ones because they are easier to place .
The use of a bronchial blocker is not practical as alternating the sides of single lung ventilation is frequently needed during the surgery. DLTs permit simultaneous ventilation of the donor and recipient lung with different oxygen concentrations and ventilation settings after the new organ has been implanted. They allow efficient unilateral irrigation and suctioning, and allow visualization for evaluation of the bronchial anastomosis without interrupting ventilation of the inflated lung. According to a meta-analysis of 39 randomized controlled trials, DLTs can be correctly positioned easier and faster than bronchial blockers . These differences become more significant when taking care of patients with severe pulmonary disease and extremely limited respiratory reserve.
Surgical approach
Double lung transplantation can be performed in the supine position by performing the traditional clamshell incision with the arms secured and padded above the head or by bilateral sternal-sparing anterior thoracotomies . Single lung transplantation can be performed by posterolateral thoracotomy in the lateral decubitus position or by sternal-sparing anterior thoracotomy in the supine position. The clamshell for double lung transplantation and the anterior thoracotomy for single lung transplantation allow for rapid access to central and groin cannulation, respectively .
Anesthetics
Anesthetic agents should be titrated cautiously as they may affect the PVR, right and left ventricular afterload and contractility, and cardiac output . There is no specific anesthetic technique, and management should be tailored to the patients’ hemodynamic conditions and response to medications. Midazolam, fentanyl, sufentanil, etomidate, propofol, and ketamine have been used. Use of succinylcholine or high dose of nondepolarizer muscle relaxants depends on the urgency for airway access.
A study investigating the direct effects of intravenous anesthetics on PVR in the isolated rat lung demonstrated that ketamine (3.0 mg/kg) is a direct pulmonary vasodilator . Ketamine has been found to increase PVR in studies of spontaneously breathing adults where it was unclear whether this was secondary to the ketamine’s hypoventilation effect . Hickey et al. concluded that ketamine had minimal effect on the pulmonary vascular resistance index (PVRI) in mildly sedated intubated infants with maintained ventilation, with normal or increased baseline PVRI . Later, Williams et al. observed that ketamine did not increase PVR in children with severe PHN, spontaneously breathing with a facemask, and under sevoflurane administration . The bronchodilating effects of ketamine have been well described in a previous study .
In a study by Rich et al., midazolam (0.3 and 3 mg/kg), etomidate (0.3 mg/kg), and propofol (2 mg/kg) did not affect PVR . Etomidate at much higher doses (3.0 mg/kg) increased PVR .
Propofol should be used with caution in patients with PHN as it decreases the systemic vascular resistance, PVR and myocardial contractility, thereby causing a considerable decrease not only in mean PAP but also in MAP . Etomidate seems to be preferred in cases of existing hemodynamic compromise as it is described to offer more hemodynamic stability . Although opioids blunt the sympathetic response to laryngoscopy and may prevent PVR increases during intubation, they may decrease the systemarterial pressure and cause hypotension. Therefore they should be titrated slowly.
In patients with compromised cardiac performance, midazolam induction was found to be an alternative that maintains cardiovascular stability . Although some reports have shown an association between midazolam and post-operative delirium, the association is mostly observed in patients older than the patient population on lung transplantation .
Although inhalation agents (isoflurane and sevoflurane) provide bronchodilation, they may cause vasodilation and myocardial depression and therefore should be introduced slowly.
Single lung ventilation with inspired isoflurane 1–1.2% was associated with significantly higher shunt but less right ventricular depression than that with propofol . MAC ≥1 with the newer volatile agents has been found to attenuate hypoxic pulmonary vasoconstriction (HPV) . Cho et al. derived similar results when comparing desflurane–remifentanil to propofol–remifentanil levels titrated to maintain appropriate anesthetic depth (bispectral index (BIS) 30–50) during single lung ventilation . Isoflurane and desflurane were found to cause similar changes in oxygenation and systemic and pulmonary hemodynamics during single lung ventilation .
Nitrous oxide is better avoided as it may increase PVR . During A-V ECMO, inhalation anesthetics should be supplemented with intravenous anesthetics to ensure adequate anesthesia as blood flow is diverted away from the lungs and toward the ECMO circulation.
Pharmacological hemodynamic support
Vasopressors and inotropes are used to counteract the hemodynamic effects of anesthetics and positive pressure ventilation. Alpha 1 and beta 1 receptor agonists and vasopressin should be readily available. Phenylephrine (α1), norepinephrine (mostly α1, α2, and some β1), epinephrine (α1, α2, β1, and β2), vasopressin (V1 and V2), and dobutamine (β1 and β2) can be used. Beta 1 agonists provide both inotropy and chronotropy, which are necessary to maintain a normal cardiac output. Milrinone (phosphodiesterase inhibitor) may also be used, but it may cause vasodilation and hypotension . Starting these medications early is crucial, so that they can circulate promptly and support hemodynamics during induction. Low vigilance and slow intervention may result in irreversible complications.
Vasopressin causes vasoconstriction by stimulating the V1 receptors . In addition to its agonist effect on the V1 receptor, it restores vascular tone in catecholamine-resistant shock states through three complementary mechanisms: modulation of ATP-sensitive K + channels, modulation of NO, and potentiation of the effects of adrenergic agents . In contrast to other vasoconstrictive agents, it has also been demonstrated to cause vasodilation in various vascular beds by activation of endothelial oxytocin receptors (OTRs), which in turn activate endothelial NO synthase . The dominant response (vasoconstriction or vasodilation) would depend on the model studied (animal vs. human, in vitro vs. in vivo), the dose and duration of vasopressin exposure, and the receptor density in the particular vasculature (V1 vs. OTR). In in vitro canine models, the vasodilatory effects of vasopressin on the pulmonary artery have been clearly demonstrated and appeared to be related to the NO pathway .
Human evidence is inconclusive as to whether vasopressin has lesser vasoconstrictive effects on the pulmonary vasculature than the catecholamines. However, both have been used in clinical practice.
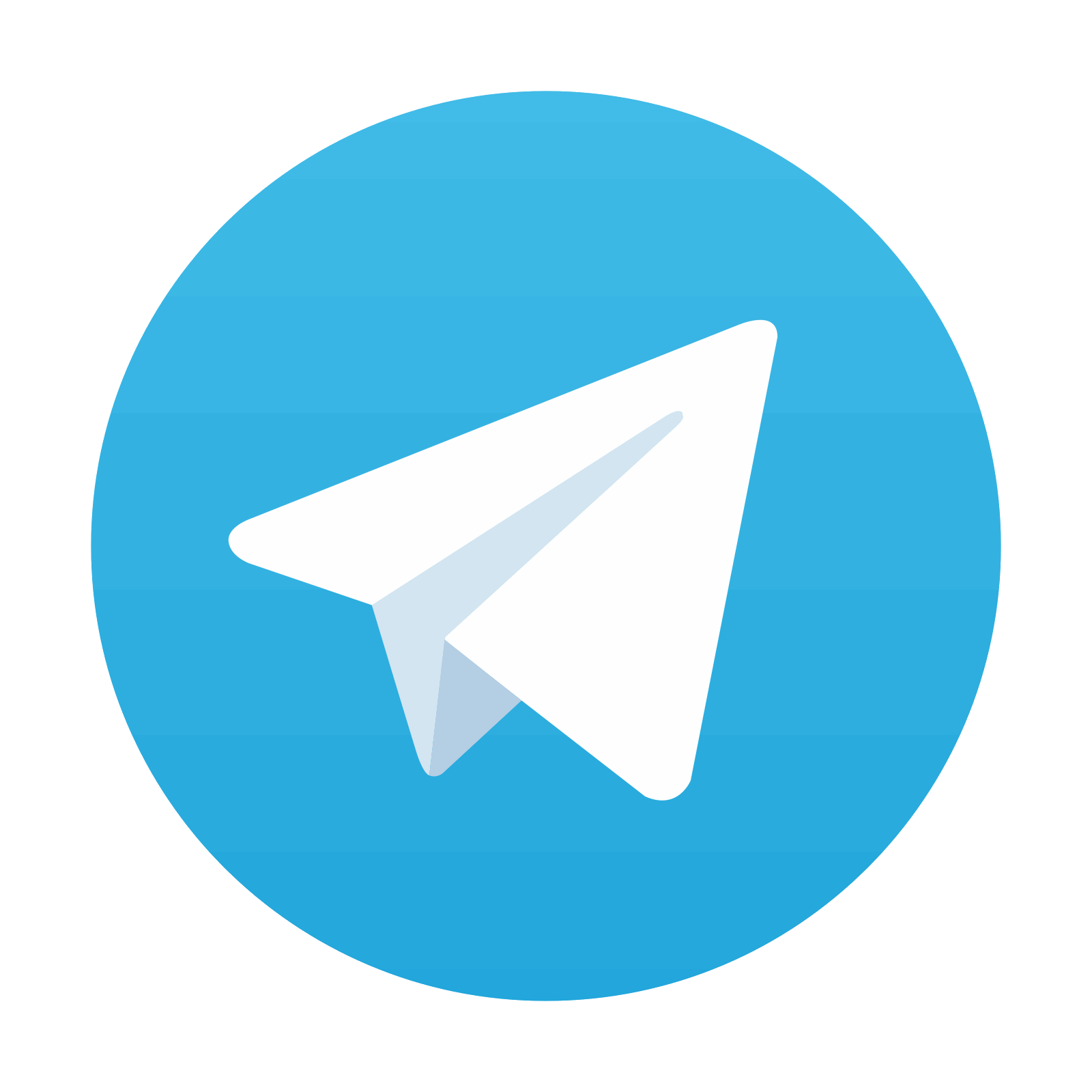
Stay updated, free articles. Join our Telegram channel

Full access? Get Clinical Tree
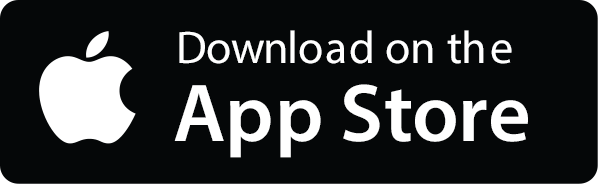
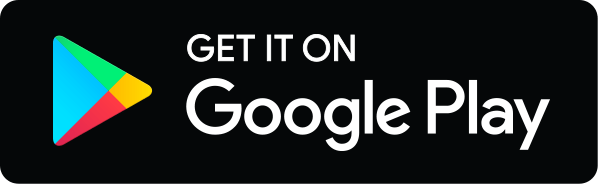