FIGURE 36.1 Typical drainage tray. A: Layout and contents of the Safe-T-Centesis catheter drainage tray. The kit includes patient prep supplies, a 6-French temporary pigtail drainage catheter, and a fluid collection system. B: Safe-T-Centesis device. At the juncture of the syringe and the drainage catheter is an indicator (arrow) that signals the operator when the needle tip enters a free cavity with a color change and an audible click. C: Pigtail catheter unrestrained in the shape it will assume in free cavity.

FIGURE 36.2 A 74-year-old patient on Coumadin developed a focal lower extremity area of swelling, erythema, and progressive pain adjacent to the knee following trauma. A: Ultrasound (US) examination demonstrates a 5.5-mL hypoechoic superficial fluid collection (asterisk). B: Hyperechoic needle (arrows) seen entering the fluid collection. C: Postevacuation US demonstration near complete drainage of the fluid collection (asterisk). The fluid was sterile and consistent with liquefied hematoma.
Postplacement Catheter Care
The catheter is connected to either a gravity drain or a suction device such as a Jackson–Pratt bulb. Output should be recorded by the nursing staff, and the catheter should be irrigated three times a day with sterile saline to maintain patency. The catheter needs to be evaluated daily to confirm patency, evaluate output, evaluate the skin entry site, and to monitor patient clinical status including temperature trend and white count.
End points to determine the time for catheter removal are as follows: decrease in the catheter output to less than 20 mL/day, normalization of temperature and white count, and overall clinical improvement of the patient. When these end points are achieved, the patient is usually reimaged and the tube withdrawn if the collection is no longer present. However, if after 2 to 3 days these end points are not achieved, repeat imaging should also be performed to determine the need for further intervention. Reported success rates for percutaneous abscess drainage are 90% to 100% (1–3) with a recurrence rate of 8% to 20%, most commonly related to early catheter removal (4).
Special Considerations
Abscess–Fistula Complex
This is a type of enteric abscess that should be suspected when high fluid output, greater than 100 mL/day, persists. In this scenario, follow-up evaluation may include injection of iodinated nonionic contrast under fluoroscopic guidance. Results of this study will help determine the need for catheter placement directly into the fistula or surgical intervention. This subgroup often requires 3 to 6 weeks of drainage in association with medical management and nutritional support.

FIGURE 36.3 Patient with diverticular disease and mesenteric abscess. A: Close proximity of bowel loops (arrows) to the 4-mL fluid collection (asterisk). B: Required CT guidance for drain placement (arrow).
Solid Organ Abscess Drainage
Hepatic, splenic, and renal abscesses can be drained percutaneously, although this sometimes requires an intercostal approach. The lowest possible intercostal level should be used if this approach is necessary to decrease the risk of pneumothorax or the potential spread of infection into the thorax, resulting in empyema. Also, due to the higher risk of bleeding with access into these organs, it is necessary to select the shortest pathway possible through normal tissue, to use a blunt needle system when traversing normal tissue, and to use smaller drainage catheters, usually 8 French. Simple needle aspiration of these collections is preferred over catheter drainage, especially when the collections are small (Fig. 36.4).
Pancreatic Collections
Drainage of pancreatic fluid collections often requires CT guidance due to the frequently associated ileus with subsequent overlying bowel loops. Therefore excellent opacification of bowel loops with oral or enteral tube administration of contrast is necessary. Pancreatic abscesses usually contain highly viscous material, which necessitates use of larger-bore catheters—greater than 16 French; pancreatic pseudocysts usually require only an 8- to 10-French drainage catheter. Additionally, not all pseudocysts require drainage, as most spontaneously resolve. Factors influencing the decision to drain the collection include suspected infection, pain, or obstruction of adjacent bowel or bile duct. Successful pseudocyst drainage can be as high as 90%. However, the results of pancreatic abscess drainage are more variable, with reports of a success rate between 32% and 80%. This wide range is possibly due to difficulty in differentiation of infected pancreatic necrosis, which is difficult to treat, from a focal abscess (5).
Empyema
Drainage of an empyema can frequently be performed at the bedside under US guidance, with the patient’s position dependent on his or her ability to assist and the exact location of the collection; a sitting position is often preferred. The collection is localized, entry site marked, site prepped, local anesthesia obtained, and dermatotomy performed followed by deep blunt dissection. A 14- to 20-French catheter is then inserted, often with the trocar technique. The empyema is aspirated and the catheter connected to Pleur-Evac water seal pleural drainage system. The overall success rate for percutaneous drainage of empyema is 77% (Fig. 36.5) (6).
Thick fluid collections may require the use of intrapleural thrombolytic injection such as tissue plasminogen activator (t-PA), to disrupt fibrin septae. This can be accomplished with an infusion of rt-PA solution containing 2 to 6 mg of rt-PA in 50 to 250 mL of normal saline. This is infused daily for 1 to 3 days. There does not appear to be any significant systemic effect associated with this intralesional infusion of the thrombolytic medication, and there is an approximate 94% clinical improvement rate (7).
Diagnostic and Therapeutic Thoracentesis
Image-guided thoracentesis can easily be performed at the bedside with US. This is most frequently performed with the patient sitting on the side of the bed facing away from the operator. In some cases, due to severity of the patient’s condition, this procedure may need to be performed with the patient supine. This can significantly increase the difficulty of the procedure, especially with smaller collections. This situation can be improved by placing the patient on a cardiopulmonary resuscitation (CPR) backboard to slightly elevate him or her off the bed, and by raising the head of bed as tolerated to drain more of the fluid into a focal dependent position near the diaphragm. Sedation is usually not required as sufficient pain control can be obtained with local anesthesia. Of note, CT may be required in some cases due to the small size and/or location of the fluid.

FIGURE 36.4 CT-guided drainage of infrahepatic abscess. A: CT scan through the fluid collection (asterisk) with localizer grid applied on the skin over the target. B: Following determination of pathway, entry site marking, sterile skin prep and drape, and local anesthesia the access needle is advanced under CT guidance into the fluid collection. Repeat CT is performed to evaluate needle path and depth (arrow). C: Postdrainage obtained at the same level as A shows loop of drainage catheter (short arrow) in markedly decompressed abscess cavity. (Courtesy of Lauren Alexander, MD, Gainesville, FL.)
Diagnostic thoracentesis is often used to differentiate a transudate from an exudate, and in the case of an exudate, to evaluate for malignancy or infection. This diagnostic procedure can be performed with a 20- to 22-gauge needle. US is used to localize the collection, and the site of entry is marked (Fig. 36.6). This area is prepped and local anesthesia obtained. The needle is then advanced close to the superior rib margin at the selected intercostal space to avoid the neurovascular bundle that courses along the inferior margin of the rib. Once entered, sufficient pleural fluid is aspirated for laboratory analysis consisting of pH, Gram stain and culture, total protein, lactate dehydrogenase (LDH), glucose, and cytology. At completion of the procedure, the needle is removed and an air-occlusive dressing applied.
Therapeutic thoracentesis is usually requested due to dyspnea or in association with catheter placement for a chemical pleurodesis. A posterolateral approach is used to decrease patient discomfort when lying down and potentially pressing on the tube rather than a direct posterior approach, which is used in standard diagnostic thoracentesis. When a catheter will be necessary only during the short drainage procedure, we use the 6-French catheter that comes in the Safe-T-Centesis kit, with its three-way stopcock and internal valves to decrease the risk of pneumothorax. The fluid is then removed with syringe aspiration or by connection to a vacuum bottle that can be changed when full. Chest pain can occur with rapid fluid removal; however, this usually resolves with temporary cessation of the drainage and with subsequent resumption of the drainage procedure at a slower rate. Coughing often occurs toward the end of the procedure as the pleural surfaces become reapposed. When longer-term access is required, a 10- to 14-French tube is placed by either trocar or Seldinger technique and connected to Pleur-Evac water seal drainage system. The overall incidence of pneumothorax with US-guided procedures approximates 3%.
If a pneumothorax occurs in association with thoracic intervention, or secondary to underlying lung disease, a chest tube/pleural catheter may be required. The indications for tube placement include size of pneumothorax greater than 25%, pain, shortness of breath, or progressive enlargement on serial radiography. These tubes are easily placed in the second or third intercostal space anteriorly. Our preference is to place an 8-French catheter under fluoroscopic guidance using the Seldinger technique to avoid injury to the adjacent lung. We gain access with a 4-French micropuncture system (Angio Dynamics, Queensbury, NY) followed by insertion of a 0.035 guidewire over which the chest tube is advanced. This is secured in place with nonabsorbable suture and covered with petroleum jelly gauze to decrease the risk of air leak. Most of the pneumothorax is aspirated using a 60-mL syringe with the aid of a three-way stopcock. Then the tube is connected to a Pleur-Evac drainage system as is done with surgically placed chest tubes. The pleural catheter remains in place until there is no significant residual pneumothorax and there is no evidence of air leak when the tube is connected to water seal (Fig. 36.7).

FIGURE 36.5 A 19-year-old patient with sepsis and cough. A: Axial CT at the level of the midchest demonstrates an 8.5-cm fluid collection in the right posterior hemithorax (asterisk) with compression atelectasis of the adjacent lung. Direct percutaneous access is blocked due to inferior margin of the scapula, overlying ribs, and aerated lung. B: Patient was placed in a left-side-down decubitus position. Change in position provides direct posterior access to the fluid collection. A localizer grid has been placed to mark site of access. C: Access gained with micropuncture needle system and then a 14-French drain placed over a wire. Fluid aspirated with syringe and then drain was secured in place and connected to suction bulb. D: Postdrain placement CT. (Courtesy of Lauren Alexander, MD, Gainesville, FL.)
Abdominal Paracentesis
US or CT guidance can be used to drain diffuse or loculated fluid collections in the abdomen. In most cases, US is sufficient and can be performed at the bedside. The site of maximal fluid collection is localized without evidence of intervening loops of bowel, and this area is marked and prepped. The procedure can usually be performed with local anesthesia without sedation. The preference at our institution is to use the 6-French Safe-T-Centesis catheter kit. This drainage procedure can be performed for diagnostic and/or therapeutic purposes. When performed as a therapeutic procedure, the catheter and tubing can be connected to either a three-way stopcock and drainage bag or to a 1-L vacuum bottle system. Removal of volumes greater than 5 L can lead to volume redistribution and may require IV albumin infusion (Fig. 36.8).
Suprapubic Drain Placement
The patients in whom placement of a suprapubic cystostomy tube are performed are those with bladder neck obstruction from prostatic hypertrophy or prostate cancer, and with a neurogenic bladder. However, there is an increasing population of ICU patients following pelvic trauma who may also require this procedure.

FIGURE 36.6 Ultrasound image showing typical appearance for thoracentesis guidance. The central target (pleural effusion) is identified by an asterisk, lung margin by arrowheads, and drainage catheter pathway with an arrow.
The procedure is performed under local anesthesia and with conscious sedation, usually with both US and fluoroscopic guidance, although in some cases this may be performed at the bedside with US guidance alone. The bladder must be distended to displace loops of small bowel out of the pelvis. This can be accomplished by the normal filling of the bladder, in the case of outflow obstruction, by filling of the bladder through an indwelling urinary bladder catheter (IUBC), or by insertion of a 20-gauge needle into the bladder and subsequent filling of the bladder with normal saline. After preparation of the site, if direct access with a needle is used, US guidance is used to localize the bladder and avoid adjacent loops of small bowel. Once distended, a point 2 to 3 cm above the pubic symphysis is localized in a paramedian location. An 18-gauge needle is advanced under US guidance, again to avoid any loops of small bowel. The position is confirmed by return of urine, or if saline has been used to distend the bladder, iodinated contrast material can be injected. On confirmation of position, a 0.035-in stiff guidewire is coiled in the bladder and the tract serially dilated. The degree of dilatation is dependent on type of catheter to be used. For short-term drainage, a soft, 10- to 12-French locking loop catheter can be used. However, for longer-term use, a 16- to 20-French Foley catheter should be used, which can be inserted through a peel-away sheath. Either the locking loop catheter or the peel-away sheath is advanced over the indwelling stiff guidewire under fluoroscopic guidance after sufficient tract dilatation.

FIGURE 36.7 A 70-year-old female with progressive pneumothorax following placement of transvenous cardiac pacing device. A: Arrows indicate the partially collapsed left lung following pacing device placement. B: Fluoroscopic image obtained at the time of 8-French chest tube insertion. The tube was placed slightly lower than the usual apical position to avoid potential damage to the pacing device or wires (arrow head). C: Following chest tube insertion, the pneumothorax was evacuated with syringe aspiration of the air. Follow-up chest radiograph demonstrates near-completed resolution. The chest tube was connected to Pleur-Evac drainage system.

FIGURE 36.8 Abdominal paracentesis. A: Initial position shows a segment of bowel blocking access to the perihepatic fluid. B: Slight change in transducer position yields a safe pathway for drainage. B, segment of bowel; L, liver; asterisk, fluid.
Catheter maintenance primarily centers around risk of infection. Some authors recommend use of long-term trimethoprim/sulfamethoxazole. Additionally, the catheter should be exchanged every 2 to 3 months or whenever it becomes clogged with debris. The overall success rate for the procedure approaches 100% (8).
CHOLECYSTOSTOMY
Surgical cholecystostomy was established as a definite technique for decompression of the gallbladder by Sims in 1878 (9). Sims demonstrated that surgical cholecystostomy with insertion of a drainage tube gave rapid relief of symptoms in patients who were otherwise too ill to undergo open cholecystectomy with gallbladder resection (9). Percutaneous access to the gallbladder was first described by Burkhardt and Muller (10) in 1921, but did not become accepted until 1980, when Shaver et al. (11) demonstrated that percutaneous drainage of the gallbladder was an effective and safe technique in patients with acute cholecystitis who were unfit for surgery (9–11). This technique consists of a minimally invasive method of removing gallbladder contents by placing a drainage catheter into the gallbladder lumen using some form of image guidance (9,12,13).
Aside from safe decompression of the gallbladder, percutaneous access to the gallbladder lumen allows additional interventional procedures to be carried out on the gallbladder and biliary tree. Some of these procedures include dissolution of gallstones using methyl-tert-butyl ether (MTBE), gallbladder ablation, percutaneous cholecystolithotomy, and drainage of the biliary tree. However, due to the advent of laparoscopic techniques in the early 1980s, these additional interventional procedures are now rarely performed.
Although surgical or laparoscopic cholecystectomy is the preferred treatment for cholecystitis in the acute setting, cholecystectomy has a substantial mortality rate related to patient issues, including advanced age and coexisting diseases (14). Elective cholecystectomy in a nonacute setting carries a mortality as low as 0.7% to 2%. However, cholecystectomy in the acute patient may have a mortality rate as high as 14% to 19% (14). Percutaneous cholecystostomy can be performed in the acute setting with low mortality rates as a temporizing measure. When the patient has improved and is better able to tolerate an operative intervention, cholecystectomy can be performed electively. In addition, in patients with acalculous cholecystitis, percutaneous cholecystostomy will decompress the gallbladder and may eliminate the need for later cholecystectomy (9).
Acalculous cholecystitis typically occurs in patients admitted for shock, major surgery, and thermal injury, as well as in patients receiving total parenteral nutrition (TPN). It is believed to be caused by inflammation and ischemia of the gallbladder, not related to the presence of stones, but rather to intravesicular hemorrhage or inspissated bile causing obstruction, leading to inflammation and infection (14,15). The diagnosis of acalculous cholecystitis can be elusive, as US and scintigraphy are diagnostically unreliable (9). Percutaneous cholecystostomy has been advocated as a therapeutic trial in these patients provided other sources of sepsis have been excluded (16). Other indications for percutaneous cholecystostomy include hydrops, empyema of the gallbladder, and complications of cholecystitis including perforation and pericholecystic abscess (Fig. 36.9A) (17).
Percutaneous cholecystostomy is usually performed in the angiography suite using US and fluoroscopy. CT scan is another modality that may be of benefit in certain patients (Fig. 36.9B). The procedure may also be performed at the patient’s bedside using only US (Fig. 36.9C). Although conscious sedation is usually administered, occasionally, if the patient is unable to tolerate conscious sedation, the procedure can be safely performed using only local anesthesia (Figs. 36.10 and 36.11).

FIGURE 36.9 A: HIDA scan performed on a patient with acute cholecystitis. Note nonvisualization of the gallbladder. B: CT scan reveals thickened gallbladder wall and fluid surrounding the gallbladder (arrow). C: Sonographic image obtained during a percutaneous cholecystostomy. Note the transhepatic approach and tip of the needle within the lumen of the gallbladder.

FIGURE 36.10 Contrast injection through the cholecystostomy tube. Note the irregular filling defects consistent with sludge or pus in the gallbladder.

FIGURE 36.11 CT scan after successful placement of percutaneous cholecystostomy. Note the catheter in the gallbladder (arrow). The gallbladder has been decompressed.
The two techniques used are the Seldinger needle and trocar techniques. The Seldinger needle technique involves, after proper preparation, placement of a fine needle, usually 22 gauge, into the lumen of the gallbladder, followed by placement of a 0.018 wire through the needle, and then dilating the site over the wire to accommodate larger wires, if needed, or placement of a drainage catheter. The trocar technique involves placement of a catheter, usually 8 French, which is held on a rigid needle with sharp stylet. This system is advanced as a unit under image guidance into the lumen of the gallbladder. Each needle technique has its own merits and is usually chosen based on operator comfort.
The Seldinger technique allows for a single puncture of the gallbladder, which is then sequentially dilated to accommodate a 0.035 wire. Fascial dilators are then used to accommodate the 8-French drain. It is during the dilation that the guidewire may buckle and access may be lost. If this occurs, the gallbladder may leak into the peritoneum and decompress, making puncture more difficult. The author’s experience suggests that if a transhepatic approach is used, this generally does not pose a problem. Alternatively, the trocar technique uses a 22-gauge needle inserted into the gallbladder to be used as a guide to advance the 8-French catheter into the gallbladder. Using this system, a swift stroke of the catheter is required to puncture the gallbladder to prevent the organ from moving away and being displaced. Also, even though bile may be aspirated, the entire catheter may not be in the lumen and when the catheter is advanced, dislodgement may occur. Again, both techniques are widely used and accepted and depend on the operator’s comfort level.
The gallbladder can be approached either via the transhepatic route (see Fig. 36.9C), attempting to enter through the anatomic bare area, or using a transperitoneal approach. The bare area is formed embryologically when the mesentery that surrounds the gallbladder fuses with the undersurface of the liver. The transhepatic approach tends to minimize bile leak in the presence of ascites. This approach also minimizes gallbladder motion by puncturing near the bare area. This is of significance as it is gallbladder motion that will lead to tube dislodgement. Puncture of the gallbladder by this approach minimizes leakage of bile as the liver will act to tamponade the tract. However, puncture through the bare area has proven difficult in all cases (18). The transperitoneal approach is more direct in some cases and allows better access than the transhepatic approach if percutaneous cholecystolithotomy is planned using a large scope because of the large tract that would have to be formed through the parenchyma of the liver to accommodate the endoscopic device. The main drawback of the transperitoneal approach is when the Seldinger technique is planned, as the gallbladder mobility allows for easy buckling of the catheters due to a lack of parenchymal support, leading to misplaced or dislodged catheters. This problem is largely eliminated if the trocar system is used in combination with the transperitoneal approach.
Cholecystostomy has been well studied and has a 100% technical success rate (see Fig. 36.11) (11,13,14,16,19). Procedure-related mortality rates are well documented in the range of 0% to 2%, well below the published rate of complications for surgical cholecystostomy in the acute setting (14,20–22). Procedure-related complications include bleeding, vagal response, and biliary peritonitis related to bile leak. Clinical improvement has been shown to occur in 1 to 2 days, and response rates of 87% have been shown at 3 days (9,23). Browning et al. (24) reported that patients with pericholecystic fluid or gallstones were the most likely to have a positive response to the procedure. Ninety-two percent of patients with gallstones showed clinical improvement after percutaneous cholecystostomy. In the ICU, where acute acalculous cholecystitis is most likely to occur, it has been shown that in patients with unexplained sepsis, percutaneous cholecystostomy has shown dramatic clinical improvement, defined as decreased white blood cell count, normalization of body temperature, and reduction in the use of vasopressors, in up to 59% of patients (9,16,19).
HEPATOBILIARY DRAINAGE
Although Burkhardt and Muller first described the technique of percutaneous cholangiography in 1921 (25), it was not until 1966 that percutaneous transhepatic biliary drainage was described by Seldinger (26), using a simple sheathed needle technique. In 1974, Molnar and Stockum (27) described percutaneous biliary drainage using a transhepatic catheter. Subsequent to this description, numerous technical advances were made in the area of guidewires and catheters, which allowed an increased ability to negotiate areas of stricture and stenosis within the biliary tree using a percutaneous approach (25). At present, there is a wide array of devices and instrumentation that allow for increased percutaneous biliary interventions, including management of biliary strictures, biliary leaks, biliary fistulae, stone extraction, biopsies, and radiation therapy.
Whereas percutaneous biliary interventions gained popularity in the 1970s and 1980s, the development of endoscopic retrograde cholangiopancreatography (ERCP) has largely replaced the percutaneous approach for many disease states. However, the technique of choice will mainly depend on the skills available by physicians at the local institution (28). Additionally, there are instances where a percutaneous approach is preferred over an ERCP, mainly instances where ERCP fails, or ERCP is not an option due to prior intestinal surgery that does not allow the endoscope to reach the biliary tree (Figs. 36.12 and 36.13).

FIGURE 36.12 Failed endoscopic placement of common bile duct (CBD) stent due to the presence of a large mass (arrow) within the CBD. (Courtesy of James Caridi, MD.)

FIGURE 36.13 Successful percutaneous drainage using a left-sided biliary duct. Note that the drainage catheter has been advanced past the obstruction and into the small intestine. (Courtesy of James Caridi, MD.)
Prior to the initiation of the procedure, any coagulopathy should be corrected and prophylactic antibiotics administered. Preprocedure imaging includes either a CT scan, US, or MRI to look for the location of dilated bile ducts and also to evaluate the anatomy of the liver. The right upper quadrant of the abdomen in the midaxillary line is prepped, using sterile technique, for an intercostal approach. Although the right-sided approach is commonly used, many interventional radiologists prefer to access the biliary tree via a left-sided ductal approach.
After conscious sedation is administered, a 22-gauge needle is advanced into the liver using fluoroscopic guidance. Diluted contrast is injected as the needle is slowly withdrawn; alternatively, the needle can be advanced and contrast injected; this technique works well for the nondilated system. After identifying the biliary system, further contrast is injected slowly to opacify the biliary tree and identify a suitable access site in the bile ducts. Next, with the contrast-filled duct as a target, the system is entered using a 22-gauge needle with fluoroscopic guidance. Thereafter, there are two options. First, an external drain can be placed to decompress the system, with the patient being brought back to the interventional radiology (IR) suite 24 hours later; this reduces the risk of introducing infected bile into the bloodstream. The second option is to attempt internal/external drainage. Although this may save the patient one step in a staged procedure and allow for physiologic drainage of bile, there is a risk of introducing infection as previously described. It is the authors’ preference to achieve external drainage first and allow the ducts to drain overnight before attempting any lengthy intervention (Fig 36.14A,B).
The indications for percutaneous biliary drainage include palliation of an unresectable primary or secondary malignancy of the liver causing biliary obstruction, benign strictures including biliary enteric anastomosis as seen in liver transplant patients, sepsis secondary to biliary obstruction, preoperative decompression, stone removal, bile leak after laparoscopic cholecystectomy, biopsies, permanent internalization of drainage by placement of internal stent, and radiation therapy (Fig. 36.14C ,D) (29).
The only true contraindication to percutaneous biliary drainage is a bleeding diathesis. Usually this problem is overcome with the administration of blood products in the form of fresh frozen plasma, platelets, and vitamin K. Relative contraindications include the presence of sepsis, unless it is of biliary origin. The presence of ascites increases the risk of bleeding and catheter misplacement, as well as making the procedure technically more difficult; hence ascitic fluid should be drained prior to performing the procedure. The presence of multiple intrahepatic obstructions also raises the risk of introducing bacteria to a bile duct that is not drained and that can rapidly become infected.
The catheter should be anchored to the skin. This is usually performed with a suture from the skin to the tube. Approximately 2 cm of slack should be available to prevent tube dislodgement from hepatic motion with respiration. The tube should be flushed with 5 mL of saline every 6 hours for the first 48 hours the tube is in place, and as needed thereafter. The drainage from the tube should be monitored daily to identify any signs of obstruction of the catheter or evidence of bleeding. If brisk bleeding occurs, this may be the result of erosion of the tube through an adjacent hepatic blood vessel that is in continuity with one of the many side holes of the drainage catheter; this usually occurs in the setting of a tube that has migrated out of position with patient motion or inadequate tube anchorage.
The tube site should be cleaned routinely to discourage bacterial colonization. Patients with an external biliary drain in place should receive IV fluids if there is marked choleresis, as this may precipitate hepatorenal failure. If any problem is identified with the tube, IR should be contacted if the problem cannot be resolved immediately on the unit (28).
Burke et al. (30) reviewed the published success rates of the technique in the literature. Canalization is easier when the intrahepatic ducts are dilated, and success rates approach 95% for the dilated system, whereas it is 70% for the nondilated biliary tree. Internal drainage is achieved in 90% of patients with successful biliary canalization. Stone removal is successful in 90% of cases. Stents placed as palliation for malignant disease are patent 50% of the time at 6 months after placement.
Percutaneous transhepatic biliary drainage carries a significant risk, approximately 10% for all patients (30), and this risk may increase or decrease based on the patient’s overall medical condition. Patients presenting with coagulopathy, cholangitis, biliary stones, malignant obstruction, or proximal obstruction will have higher complication rates (30). Complications related to insufficient bile drainage and tube dislodgement is usually relieved with placement of a tube 10 French in size. Sepsis, reported in 2.5% of cases, may be considerable unless prophylactic antibiotics are used prior to the procedure; bleeding is reported with 2.5% of cases as well. Abscess formation or peritonitis is noted in 1.2% of patients; pleura entry is noted in 0.5% of cases. Death is reported in 1.7% of patients.
TRANSJUGULAR INTRAHEPATIC PORTOSYSTEMIC SHUNT
Portal hypertension refers to a pathologic increase in pressure in the portal vein. Although it is defined as an increase in portal venous pressure above 12 mmHg, the standard is to report the portal pressure as a gradient between the portal vein and the inferior vena cava (IVC) (31). The gradient becomes important because there are several conditions, including pregnancy and ascites that may elevate the absolute portal pressure or the pressure in the IVC.

FIGURE 36.14 Patient presented with biliary obstruction. A: On hospital day 1, external drainage has been achieved using a right-sided bile duct approach. Cholangiogram shows complete obstruction in the mid common bile duct (CBD) (arrow). B: On hospital day 2, internal/external drainage has been achieved by gaining access to the small intestine across the area of obstruction. C: A metallic self-expanding stent has been placed across the area of obstruction in the distal common bile duct. Note the waist in the stent (arrow) due to the surrounding mass. D: Post–stent placement and balloon angioplasty. No residual stenosis is noted, and the stent is fully expanded.
The liver is the main source of resistance to blood flow in the portal vein (32). Certain disease states, including cirrhosis, disrupt the normal architecture of the liver and lead to formation of fibrosis around the hepatic venules and sinusoids. This fibrosis reduces the diameter of the sinusoids, thus acting to increase resistance. As the pressure in the portal vein increases, a typical physiologic response is the development of portosystemic collaterals, such as varices, as an alternative pathway for blood to return to the heart. Certain patterns of varices are commonly seen, with the classic distribution in the lower esophagus and stomach. Other common patterns include mesenteric varices and connections between the spleen and kidney. Generally, varices begin to form with a portal pressure greater than 12 mmHg. Risk of variceal hemorrhage increases as the portal pressure rises to 18 mmHg.
There have been several methodologies used in treating the complications associated with chronic portal hypertension. Nicolai Eck was the first to achieve surgical portal flow diversion in 1877 (33). In 1945, Whipple et al. (34) and Blakemore and Lord (35) began using portacaval and conventional splenorenal shunts in clinical practice. Since that time, other shunt procedures have been developed, including end-to-side portacaval shunt, side-to-side portacaval shunt, distal splenorenal shunt, as well as various interposition shunts, all aimed at reducing the pressure in the portal vein and treating variceal hemorrhage (32). Surgery remained the standard until the 1980s when endoscopic techniques were popularized, including direct injection of the bleeding varix with a sclerosing agent. Additional therapies offered through use of an endoscope include banding and ligation of the bleeding varices. Other forms of medical management include administration of vasopressin or vasopressin combined with nitroglycerin using an IV route. Vasopressin and its derivatives act by constricting the vascular smooth muscle altering the body’s arterial beds including the splanchnic arteries, and thereby reducing the portal pressure by reducing the portal inflow (32). Sclerotherapy has also been performed by direct injection of sclerosing agents into the varices.
The main complication of a successful surgical shunt is the development of encephalopathy, whereas the main drawback to endoscopic and other forms of medical management is that the offending process, portal hypertension, is not addressed, which leads to high rates of rebleeding.
Transjugular intrahepatic portacaval shunting (TIPS) was first conceived of and performed in dogs in 1969 by Josef Rosch et al. (36). The first human case of percutaneous TIPS was reported in 1988 (37). It is an effective nonsurgical and nonendoscopic means to control variceal bleeding by decompressing the portal venous system (38). The technique consists of a canalization of the right internal jugular vein to access the hepatic veins. Passage of a long curved needle is then performed from a satisfactory location in the hepatic vein, usually the right hepatic vein, into the identified location in the intrahepatic portion of the portal vein or its branches (Fig. 36.15). If acceptable, balloon angioplasty of the intrahepatic tract between the hepatic vein and the portal vein is then performed and a stent, usually covered, is deployed from the portal vein to the hepatic vein through the tract to keep it patent and prevent hepatic recoil and restenosis (Fig. 36.16).

FIGURE 36.15 Patient with cirrhosis undergoing a transjugular intrahepatic, portacaval shunting (TIPS) procedure. Catheter (white arrow) is positioned from a hepatic venous approach, through the hepatic parenchyma into the portal vein (black arrow). (Courtesy of Harry K. Meisenbach, MD.)

FIGURE 36.16 Post–transjugular intrahepatic, portacaval shunting (TIPS) angiogram shows a patent TIPS shunt with a covered stent (black arrow). (Courtesy of Harry K. Meisenbach, MD.)
When TIPS was first described, it was indicated for uncontrollable esophageal variceal hemorrhage; other indications included gastric or intestinal variceal hemorrhage. The indications have since expanded and now include recurrent variceal hemorrhage despite repeated endoscopic treatment, refractory ascites, hepatic hydrothorax, Budd–Chiari syndrome, and as a bridge to liver transplantation. TIPS produces profound hemodynamic effects secondary to portosystemic diversion, including decreasing flow to the liver and increasing that to the heart. There are several situations where TIPS is absolutely contraindicated. These include severe or rapidly progressive liver failure, severe encephalopathy, and congestive heart failure. Relative contraindications include biliary obstruction, hepatic malignancy, portal vein thrombosis, and polycystic liver disease; additional considerations include the patient’s coagulation status. The most frequent complication is bleeding related to perforation of the hepatic capsule by the needle during attempted canalization of the portal vein (39,40). Hemobilia can be seen if the needle perforates a bile duct in close proximity to a vascular structure. The needle may leave the capsule of the liver and puncture adjacent organs including the intestine, gallbladder, kidney, and aorta, among others (Figs. 36.17–36.19). Dilation and stenting of the tract through the liver may also produce numerous complications. If the puncture of the portal vein is extrahepatic, as it has been shown to be in 50% of patients (41), and the tract dilated, fatal hemoperitoneum may result. Encephalopathy is not uncommon post-TIPS and ranges from 12% to 34% (38).
The patient’s ability to tolerate a TIPS procedure, as well as being a predictor of early mortality, has been calculated in the past using the Child-Pugh classification and the acute physiology and chronic health evaluation II (APACHE II) scores. Today the Model for End-stage Liver Disease (MELD) score has largely replaced other calculations as the best predictor of early mortality. The MELD score is based on the patient’s INR, total bilirubin, and creatinine (Cr). The formula is as follows:

FIGURE 36.17 Attempted canalization of the portal vein during a transjugular intrahepatic, portacaval shunting (TIPS) procedure. This CO2 injection identifies canalization of the hepatic artery (black arrow).

FIGURE 36.18 Attempted canalization of the portal vein during a transjugular intrahepatic, portacaval shunting (TIPS) procedure. The tip of the cannula is in the renal pelvis (black arrow).

FIGURE 36.19 Attempted canalization of the portal vein during a transjugular intrahepatic, portacaval shunting (TIPS) procedure. The tip of the cannula is in the left hepatic duct (black arrow). Contrast is noted throughout the biliary system and into the common bile duct (white arrow).
MELD score = 10{0.957Ln(Cr) + 0.378Ln (Total bili)
+ 1.12Ln(INR) + 0.643}
The maximum value for the MELD score is 40 and a MELD score of 18 has generally been shown to be the cutoff for performing a TIPS procedure.
Follow-up care for TIPS patients includes routine US surveillance of the stent to confirm patency and exclude restenosis or thrombosis of the stent. US is usually performed within 1 to 2 weeks after placement of the TIPS; it is repeated at 3 months, 6 months, and then every 6 months and as needed for early identification of any problems with the shunt. It is much easier to maintain a working shunt than to place a new TIPS.
TIPS can be performed with extremely high rates of success, approximating 92% to 99%. Reasons for a failed TIPS procedure include portal vein thrombosis, atrophic hepatic veins, small hard livers, and massive ascites. For patients in whom TIPS was performed for hemorrhage, the procedure was successful in stopping the bleeding acutely in 91.1%, although the risk of rebleeding increases with time up to 20.7% at 2 years. The fatality rate ranges from 0.6% to 4.3%, and the published, less than 30-day mortality rates range from 4% to 36%. The 5-year mortality rate averages 31.7%, with the most common cause of death being progressive hepatic failure (38).
CENTRAL VENOUS ACCESS
Central venous access and maintenance is one of the most commonly performed procedures in IR (42). The demand for stable, secure, and dependable central venous access has increased dramatically over the past several years as medical therapies have become more complex, and patients present with multiple comorbidities. Additionally, outpatient services have increased sharply as providers have come under increased pressure to reduce hospital costs (43). Today, over 5 million central venous catheters (CVCs) are placed each year in the United States (44,45). CVCs are used in the ICU for infusion of a wide array of medications, blood products, TPN, and can be used for blood draws, hemodialysis, and plasmapheresis as well as many other reasons including contrast administration for diagnostic imaging.
Image-guided procedures allows the IR service to be more involved with vascular access, predominantly in difficult access patients (Fig. 36.20). Studies have shown that image-guided vascular access is generally superior to blind insertion, with fewer immediate complications and improved long-term function (1–3) and overall lower hospital costs in compared to surgically placed catheters in the operating room (46). Certainly, imaging is critical during any complex vascular access procedure including translumbar and revascularization techniques, but the role for image guidance is gaining importance even in routine vascular access procedures as the need for rapid and safe central venous access increases.
Peripherally Inserted Central Catheters
In peripherally inserted central catheters (PICCs), a peripheral vein in the upper extremity—usually the antecubital, basilic, or cephalic—is cannulated, most often with US guidance, and a long flexible catheter, essentially a long IV line, is advanced from this puncture site to position its tip in the superior vena cava (SVC) near the right atrium. PICCs come in various sizes and styles, either single- or double-lumen versions, and range from 3 to 7 French. They may have internal valves near the hub, or even on the tip of the catheter, which are important for patients with an allergy to heparin. These valved PICCs are able to be flushed with normal saline, as heparin is not needed to maintain patency. There may also be external clamps that occlude the catheter when it is not in use. The advantage of PICCs includes that they are well tolerated by patients, they look and function like a peripheral IV, which allows for ease of use by the nursing staff, and additionally, PICCs carry a lower procedural risk than central lines because they are placed in a peripheral vein, avoiding the vital structures in the chest. The single- and double-lumen varieties usually provide sufficient access for most patients. A new “power” PICC configuration has been developed that allows for higher pressures during high-flow contrast injections as seen in CT scans for diagnostic purposes. Many hospitals have entire PICC line teams, composed of nurses who specialize in placing these at the bedside. If the team is unsuccessful, then the PICC line can usually be placed by an IR clinician or another caregiver specializing in these procedures. Although PICCs are widely used with rapidly increasing acceptance by patients and clinicians alike, there are some associated complications with this type of catheter. One should be aware of the potential complications and long-term ramifications so that the appropriate access can be chosen for each patient.

FIGURE 36.20 Grayscale sonographic image of the neck demonstrates clear visualization of the left internal jugular vein (white arrow). The left internal carotid artery is seen as well (black arrow). Additional features used to differentiate the two structures are the increase in vein caliber with Valsalva maneuver compared with the typical arterial pulsation of the carotid artery.
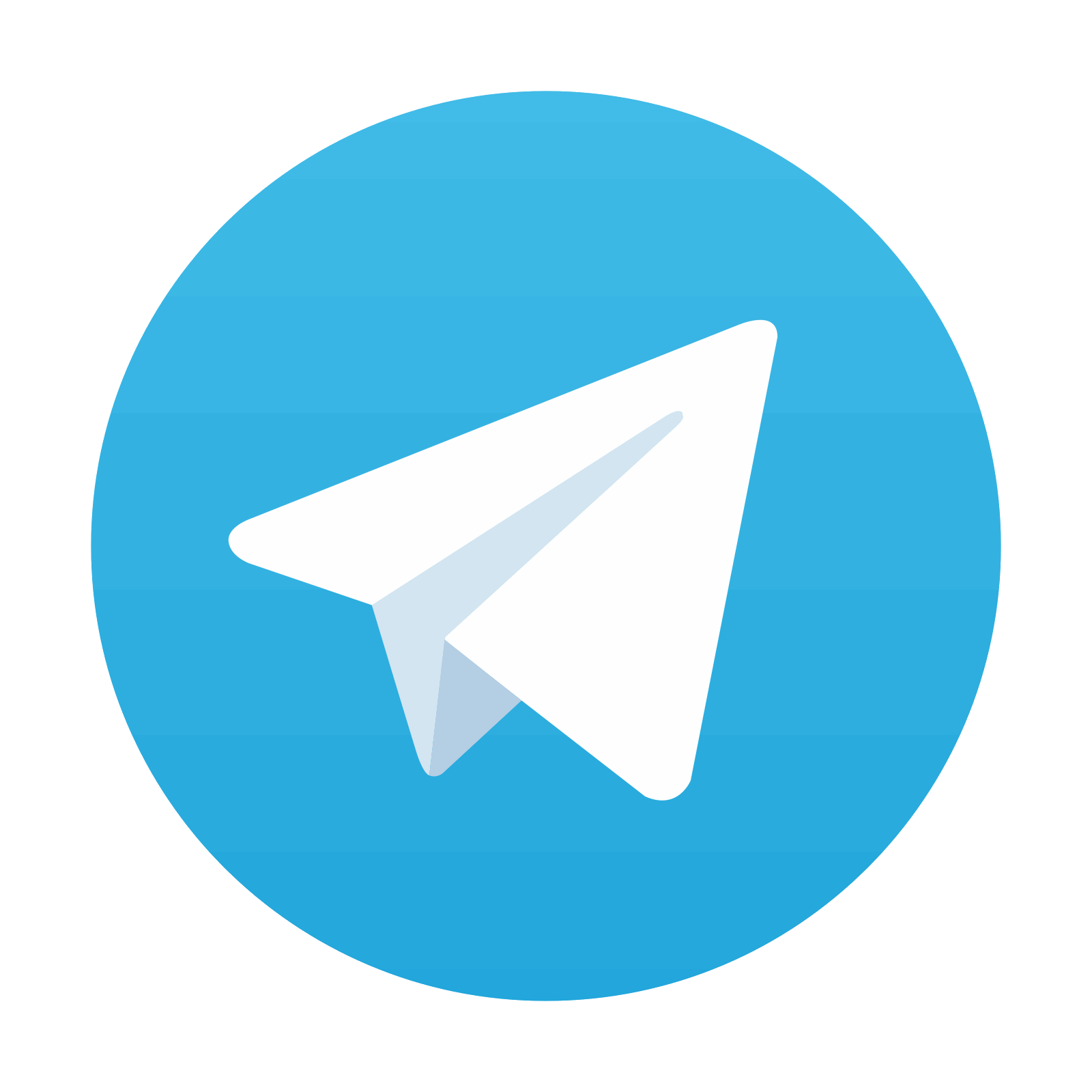
Stay updated, free articles. Join our Telegram channel

Full access? Get Clinical Tree
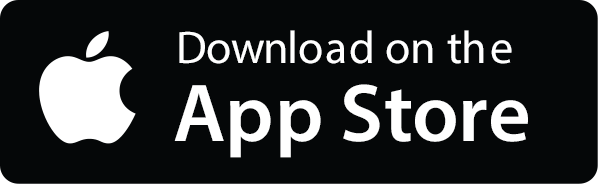
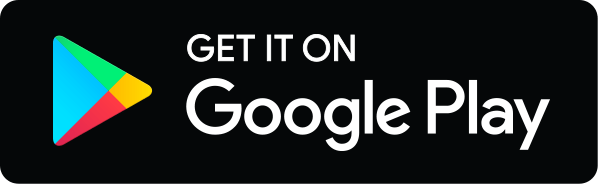