Chapter 5 Inhaled Anesthetics
Physical properties
4. Why are vaporizers required for the inhaled administration of volatile anesthetics?
5. Describe how a vaporizer for volatile anesthetics works.
6. What are the characteristics of desflurane that preclude its delivery in the conventional variable-bypass vaporizer?
7. What considerations must be taken into account when administering inhaled anesthetics at high altitude?
8. What are two potentially toxic compounds that can be produced as a result of the degradation or metabolism of volatile anesthetics?
9. What is a potentially toxic compound that can be produced as a result of the interaction between sevoflurane and the carbon dioxide absorbent? What factors may increase this risk?
10. What is the potential risk of human exposure to compound A? How can this risk be minimized?
11. What is a potentially toxic compound that can be produced as a result of the interaction between desflurane and the carbon dioxide absorbent? What factors may increase this risk?
12. What is the potential risk of carbon monoxide production from the carbon dioxide absorbent?
Relative potency of inhaled anesthetics
13. How are relative inhaled anesthetic potencies compared?
14. What are MAC values for isoflurane, sevoflurane, desflurane, and nitrous oxide in a 30- to 55-year-old?
15. What concentration of anesthetic is sufficient to provide amnesia in volunteers? How does this value relate to surgical patients?
16. What factors increase MAC?
Pharmacokinetics of inhaled anesthetics
18. Describe the process by which induction of anesthesia is achieved by an inhaled anesthetic.
19. What six factors determine the alveolar partial pressure of anesthetic?
20. Describe a strategy that allows maintenance of stable anesthetic partial pressure in the brain after the induction of anesthesia.
21. How does a shunt affect the induction of an inhalation anesthetic?
22. How is anesthetic solubility expressed?
23. How does anesthetic solubility influence speed of induction?
24. What is the “second gas effect”?
25. How does nitrous oxide affect the enzyme methionine synthase? How might this relationship affect patients receiving nitrous oxide?
26. How does nitrous oxide affect closed air-filled spaces in the body? What is the clinical relevance of this?
27. What are some differences between the induction of inhaled anesthesia and recovery from anesthesia?
28. How are volatile anesthetics metabolized?
Circulatory effects
30. Why might an individual patient’s responses vary in the circulatory effects of equipotent doses of a given inhaled volatile anesthetic?
31. How do inhaled volatile anesthetics affect arterial blood pressure? What is the mechanism by which this effect occurs?
32. How does the substitution of nitrous oxide for an equipotent portion of volatile anesthetic affect arterial blood pressure at a given anesthetic dose?
33. How do inhaled volatile anesthetics affect heart rate? What is the mechanism by which this occurs?
34. How do inhaled volatile anesthetics affect cardiac output?
35. How do inhaled volatile anesthetics affect myocardial rhythm?
36. How do inhaled volatile anesthetics affect myocardial conduction?
37. How do inhaled volatile anesthetics affect coronary artery blood flow? What is coronary artery steal syndrome? What is its clinical relevance?
Effects on ventilation
38. How is the rate of breathing affected by inhaled volatile anesthetics?
39. How is the tidal volume affected by inhaled volatile anesthetics?
40. How is the minute ventilation affected by inhaled volatile anesthetics? How is the overall pattern of ventilation affected by inhaled volatile anesthetics?
41. How is the ventilatory drive affected by inhaled volatile anesthetics?
42. How does the addition of nitrous oxide to a volatile anesthetic affect the ventilatory drive and the resultant PaCO2?
43. How do inhaled volatile anesthetics affect hypoxic pulmonary vasoconstriction?
44. How do inhaled volatile anesthetics affect bronchial tone?
45. How do inhaled anesthetics differ in their capacity to cause airway irritation? How do these differences affect their use in various clinical situations?
Other organ system effects
46. How does nitrous oxide affect cerebral blood flow and intracranial pressure?
47. How do inhaled volatile anesthetics affect cerebral blood flow and intracranial pressure?
48. How do inhaled volatile anesthetics affect cerebral metabolic oxygen requirements?
49. How do inhaled volatile anesthetics affect evoked potentials?
50. What electroencephalographic (EEG) changes occur with increasing concentration of inhaled volatile anesthetics?
51. How do inhaled volatile anesthetics affect neuromuscular function?
52. Which inhaled anesthetics have the potential to trigger malignant hyperthermia?
Answers*
Mechanism of action
1. Characteristics of the anesthetic state include immobility, amnesia, analgesia, and skeletal muscle relaxation. (81)
2. Characteristics of the anesthetic state that are achieved by inhaled volatile anesthetics include immobility, amnesia, and skeletal muscle relaxation. Analgesia is difficult to define in an amnestic, immobile patient, but surrogate measures of perception of painful stimuli (i.e., increases in heart rate or blood pressure at the time of incision or intubation) suggest that inhaled anesthetics do not possess analgesic characteristics at concentrations typically used in clinical practice. (81)
3. Nitrous oxide contributes to immobility, but is not reliable in doing so when administered alone. It has amnestic effects at higher concentration (although these are difficult to assure), and in contrast to potent inhaled anesthetics, does not contribute to skeletal muscle relaxation. (81)
Physical properties
4. Volatile anesthetics exist as liquids at room temperature and at atmospheric pressure. The inhaled delivery of these anesthetics requires that the anesthetics be vaporized. Vaporizers allow not only the vaporization of liquid anesthetics, but they also reliably and accurately deliver the specified concentration of anesthetic to the common gas outlet and ultimately to the patient. Nitrous oxide exists as a gas at room temperature and therefore does not require a vaporizer for inhaled delivery to a patient. (81)
5. Conventional volatile anesthetic vaporizers are classified as agent-specific, variable-bypass, flow-over, temperature-compensated, out-of-circuit vaporizers. After passing through the flowmeters, gases mix in the common manifold, then enter the vaporizers. Once in the vaporizer there are different streams of flow that the gases can take. The gases may be diverted by a temperature-compensating bypass valve to the bypass chamber, or they may enter the vaporizing chamber.
6. The two characteristics of desflurane that preclude its delivery in a conventional variable-bypass vaporizer are its volatility and its potency. At 20 °C, the vapor pressure of desflurane is 669 mm Hg, whereas those of isoflurane and sevoflurane are 238 mm Hg and 157 mm Hg, respectively. In addition, the boiling point of desflurane is near room temperature. Because of its volatility, erratic and dangerously high concentrations of desflurane would be delivered if a conventional variable-bypass vaporizer were to be used. The Tec-6 heated vaporizer was developed to address this problem. Desflurane’s potency is substantially lower than that of other volatile anesthetics—roughly three times less than that of sevoflurane and almost five times less than that of isoflurane. Thus, the large number of molecules converted from liquid to gas phase would create a large cooling effect (from the heat of vaporization) and it would not be possible to compensate without externally heating the anesthetic. Thus, desflurane vaporization requires a vaporizer that is electrically heated and pressurized for these reasons. (83 and Table 8-1)
7. Although vaporizer output is conventionally expressed in volumes percent, the pharmacologically relevant measure is anesthetic partial pressure. Administration of anesthesia at high altitude will result in higher volumes percent vaporizer output when a variable bypass vaporizer is used. However, the increase in anesthetic partial pressure will be minimized by the overall decrease in ambient pressure, and the clinical effect will be very small. On the other hand, the Tec 6 vaporizer behaves differently, since it is a blender of two gases and maintains constant volumes percent output. Therefore, at high altitude, although the volumes percent output will be unaffected, the delivered partial pressure will be substantially smaller and an adjustment must be made to avoid unintentional delivery of partial pressures below those clinically needed. The anesthesiologist should select the desired anesthetic vaporizer setting that would be appropriate at sea level, and multiply by this value by the ratio of sea level divided by the local barometric pressures. (82-83)
8. Two potentially toxic compounds that can be produced as a result of the degradation or metabolism of volatile anesthetics include compound A and carbon monoxide. (83)
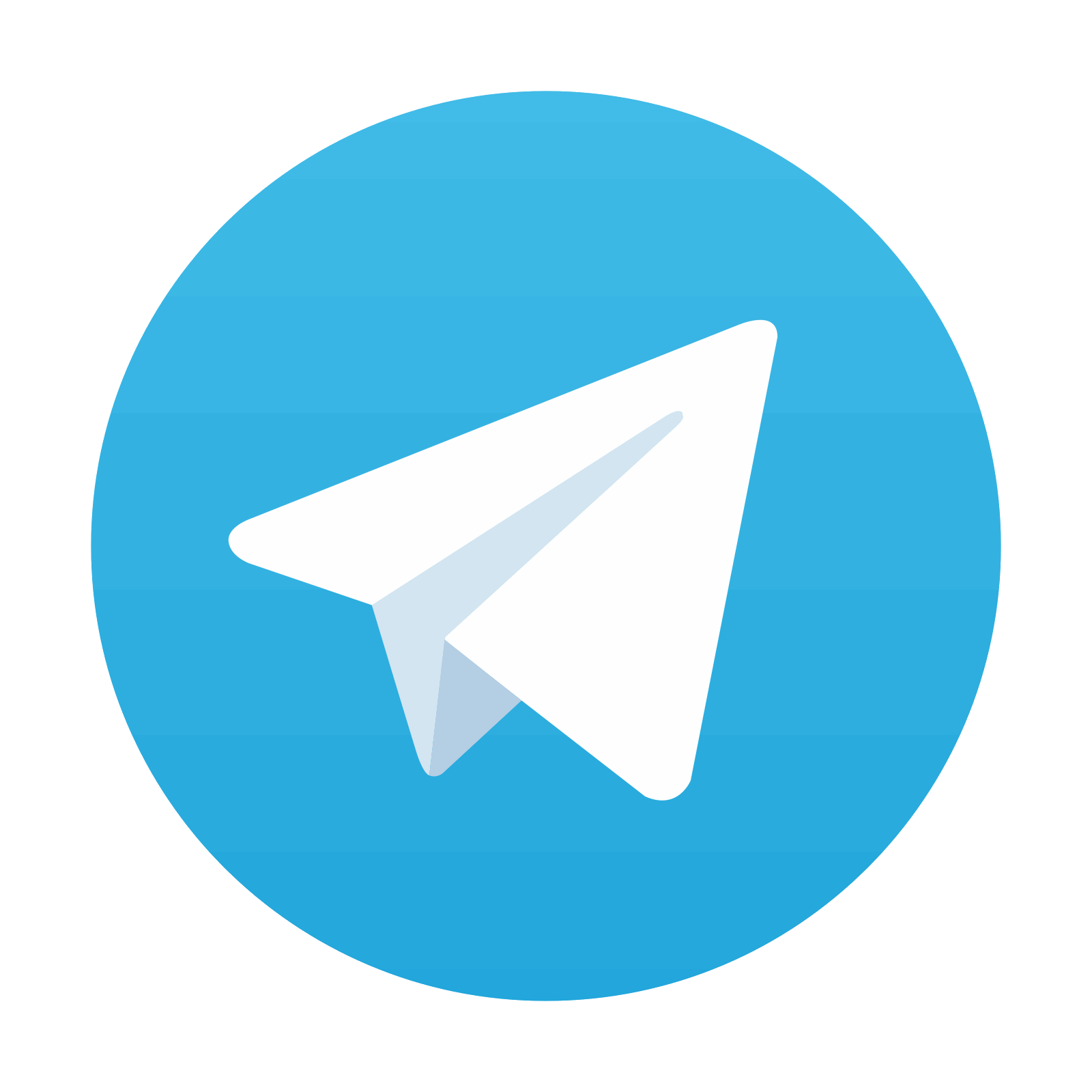
Stay updated, free articles. Join our Telegram channel

Full access? Get Clinical Tree
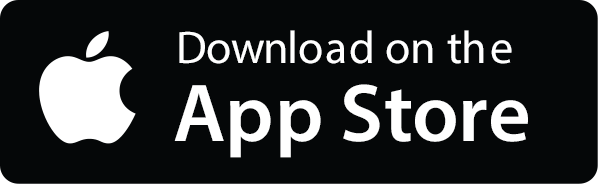
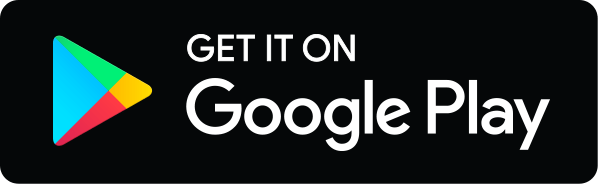