The risk of infection in corticosteroid-treated patients is related to the dose and duration of therapy (13,14). Those treated with more than 10 to 20 mg/day of prednisone for more than 3 to 4 weeks are at risk for infectious complications. Corticosteroids place the host at risk for fungal, viral, protozoal, and intracellular bacterial infections. Common pathogens to be considered in corticosteroid-treated patients presenting with a suspected infectious complication include Pneumocystis jirovecii, Listeria monocytogenes, Legionella, and Nocardia species. Bolus treatments with corticosteroids (e.g., for graft-vs.-host or autoimmune disease) may convert colonization (e.g., Aspergillus) to invasive infection.
The Solid Organ Transplant Patient
With improvements in surgical techniques and immunosuppressive therapy, a growing number of people are living with solid organ transplants. Intensified immunosuppression has decreased the incidence of graft rejection, while infectious complications are an important cause of morbidity and mortality. Interestingly, both a recent case-controlled and retrospective cohort study suggest that in hospital mortality in abdominal organ transplant patients with bacteremia and/or sepsis is lower than in the general population (15,16). Further confirmation of these results as well as additional research to understand the mechanism of this finding are needed.
Although all transplant recipients are at increased risk of infection compared to the general population, the risk of infection in an individual recipient is determined largely by the degree of exposure to potential pathogens and the overall or “net state of immunosuppression” (see Table 90.1) (17). These patients are differentiated from other immunocompromised hosts by the technical aspects (complex surgery) and the need for lifelong immune suppression to maintain graft function.
In an individual, the net state of immunosuppression is determined by the immunosuppressive agents selected as well as the dose, duration, and sequence of use. In addition, factors such as underlying immune deficiencies, metabolic derangements, the presence of foreign bodies (e.g., surgical drains, CVCs) or fluid collections, and infection with immune-modulating viruses such as cytomegalovirus (CMV), Epstein–Barr virus (EBV) or human immunodeficiency virus (HIV) contribute to the risk of infection (17,18).
TABLE 90.2 Infections Associated with Specific Immune Defects | ||
![]() |
With standardized immunosuppressive regimens, specific infections vary in a predictable pattern depending on the time elapsed since transplantation (Fig. 90.1) (17). This is a reflection of the changing risk factors over time including surgery/hospitalization, immune suppression, acute and chronic rejection, emergence of latent infections, and exposures to novel community infections. The pattern of infection changes with the immunosuppressive regimen–for example, use of pulse dose steroids or T-cell depletion for graft rejection—intercurrent viral infections, neutropenia, or significant epidemiologic exposures, such as travel or food. The timeline remains a useful starting point, although it has been altered by the introduction of newer immunosuppressive agents (e.g., sirolimus) and patterns of use including reduced use of corticosteroids and increased use of antibody-based induction therapies. Routine antimicrobial prophylaxis, improved molecular assays, antimicrobial resistance, transplantation in HIV- and hepatitis C virus (HCV)-infected individuals have also impacted the timeline (18). Figure 90.1 demonstrates three overlapping periods of risk for infection after transplantation, each most often associated with unique groups of pathogens.
- The perioperative period to approximately 4 weeks after transplantation, reflecting surgical and technical complications. Most infections are related to the surgery, similar to those occurring in the complex general surgical population, such as pneumonia, surgical site, urinary tract, and CVC-associated infections caused by typical bacterial and fungal pathogens such as Candida. Nosocomial pathogens including methicillin-resistant Staphylococcus aureus (MRSA), vancomycin-resistant enterococcus (VRE), fluconazole-resistant Candida species, Clostridium difficile, P. aeruginosa, and carbapenem-resistant Enterobacteriaceae are increasingly common. Uncommonly, infections may be transmitted from a bacteremic or fungemic donor with potentially serious complications, including seeding of the vascular suture line. The use of prophylactic antibiotics in the recipient, directed by donor culture results, may allow organs from infected donors to be safely used without compromising transplant outcomes (19–21).
- The risk for infections in the period from 1 to 6 to 12 months after transplantation are driven largely by the rapidity of tapering of immunosuppression, the use of antilymphocyte “induction” therapy, the use of antiviral (anti-CMV) and anti-Pneumocystis prophylaxis, and the reactivation of latent viral infections. Opportunistic infections include viral (CMV, varicella zoster virus [VZV], EBV, HCV), bacterial (Nocardia, Listeria, and tuberculosis), fungal (Aspergillus, Pneumocystis, and Cryptococcus), or parasitic (Toxoplasma and Strongyloides) infections.
- The period beyond 6 to 12 months after transplantation reflect community-acquired exposures and some unusual pathogens based on the level of maintenance immunosuppression. Commonly, these include viral respiratory infections, pneumococcal pneumonia, and gastroenteritis. Herpes simplex, CMV, and shingles infections may emerge. Intense exposure to an opportunistic pathogen may result in disease; transplant recipients should be counseled to avoid high-risk exposures such as unpasteurized milk products (22). Recipients requiring augmentation of immunosuppression for management of graft rejection, as well as those with chronic or recurrent CMV or HCV infections, remain at risk for other opportunistic infections, particularly P. jirovecii, invasive fungal infection, and EBV-associated, posttransplant lymphoproliferative disease (PTLD) (19–25).

FIGURE 90.1 The timeline of infection after transplantation. MRSA, methicillin-resistant Staphylococcus aureus; VRE, vancomycin-resistant enterococcus; HSV, herpes simplex virus; CMV, cytomegalovirus; HBV, hepatitis B virus; EBV, Epstein–Barr virus; TB, tuberculosis; PJP, Pneumocystic jirovecii; UTI, urinary tract infection. (Adapted from Fishman JA, Rubin RH. Infection in organ-transplant recipients. N Engl J Med. 1998;338[24]:1741.)
Hematopoietic Stem Cell Transplant Recipients
A growing number of patients undergo allogeneic and autologous hematopoietic stem cell transplantation (HSCT) procedures for both malignant and nonmalignant conditions (26). Despite advances, severe infectious complications are common, with up to 40% of HSCT recipients requiring intensive care unit (ICU) admission, and 60% of these needing mechanical ventilation, which is associated with a high mortality rate (27,28). Although traditionally poor, the outcomes of HSCT recipients admitted to the ICU are improving with advances in infection prevention, diagnosis and management, and ICU care (29); the combination of allogeneic transplantation, mechanical ventilation, and vasopressor use is predictive of mortality (30).
Advances in stem cell source and nonmyeloablative conditioning have resulted in shorter periods of neutropenia and less severe mucositis and hepatic venoocclusive disease; the risk of early bacterial infections is decreased while the risks of late viral, fungal, and bacterial infections persist (31,32). In part this reflects increased use of positively selected CD34+ progenitor cells for transplant which results in significant T-lymphocyte and monocyte depletion of the graft and therefore increases the risk of opportunistic infection while reducing malignant cells and reducing graft-versus-host disease (GVHD) (32). Reduced-intensity or nonmyeloablative-conditioning regimens have been developed in attempt to extend these therapies to older and more medically complex patients (34) and although the period of neutropenia is shorter, and potent antitumor effects result from these transplants, patients remain at high risk for GVHD necessitating significant immunosuppression for GVHD prophylaxis (31,33–35). As a result of pretransplant chemotherapy, with or without total-body irradiation, both humoral- and cell-mediated immunity are diminished. Natural host barrier defenses are also impaired by mucositis and the use of vascular access catheters.
The timeline of infectious complications in the HSCT recipient is generally divided into three phases.
- The pre-engraftment phase—the period from conditioning therapy to engraftment when patients are neutropenic. Prolonged neutropenia carries the risk for bacterial and fungal infections. Similar to other neutropenic hosts, there has been a shift from predominantly gram-negative to gram-positive—coagulase-negative Staphylococci, Streptococci, Enterococci—bacterial infections with the use of fluoroquinolone prophylaxis, to which the streptococci are generally resistant (4). Many centers use azole prophylaxis to reduce the incidence of candidemia; however, azole-resistant fungemia remains common (36,37). Herpes simplex virus may also reactivate during this phase but can be prevented with prophylactic acyclovir in seropositive HSCT recipients.
- The second phase, from engraftment to day 100 or for the duration of treatment for acute GVHD, is characterized by deficits in cellular immunity. The most important pathogens in this period are viral, particularly CMV and adenovirus, and invasive mold infections. The most common manifestations of CMV disease in HSCT recipients are pneumonia and gastrointestinal disease (38). Despite treatment with intravenous ganciclovir and CMV-hyperimmune globulin or intravenous immunoglobulin, the mortality from CMV pneumonitis remains high at 50% or greater (38). The use of ganciclovir or valganciclovir for prophylaxis or preemptive therapy has resulted in a decreased risk of CMV infection during this period, with most infections now occurring after discontinuation (39). Adenovirus may produce severe hepatitis.
Interstitial pneumonitis is an important clinical syndrome presenting during the postengraftment phase; etiologies include CMV, respiratory viruses, or idiopathic pneumonia syndrome (IPS, which is overdiagnosed). P. jirovecii can be eliminated as a cause of pneumonitis with TMP-SMX prophylaxis. Respiratory viral infections, such as influenza, respiratory syncytial virus (RSV), parainfluenza virus, and human metapneumovirus are commonly recognized as etiologies of pneumonia in HSCT (40). A number of noninfectious etiologies must also be considered in the differential diagnosis, including IPS and diffuse alveolar hemorrhage.
Most invasive mold infections occur during the postengraftment phase, and are associated with treatment of acute GVHD (37). Although Aspergillus continues to be the predominant pathogen, non-Aspergillus molds, including Zygomycetes, Fusarium, and Scedosporium species are important pathogens in this population (41,42). Invasive mold infections generally present as pulmonary nodules or pneumonia, but invasive fungemia with septic emboli, sinus disease, and disseminated disease including central nervous system (CNS) involvement are other common presentations.
- In the late phase, beyond day 100 following engraftment and with chronic GVHD, the incidence of infection is determined by the level of immunosuppression required for the GVHD. Beyond 100 days post transplant, there is gradual recovery of humoral and cellular immune function, but immune reconstitution is often incomplete and antimicrobial prophylaxis may simply shift risk to later periods (43). Up to 40% of HSCT recipients develop VZV infection, generally as a result of reactivation of latent infection, with most cases occurring during the first year. Late CMV disease may occur and is associated with a history of early CMV disease and GVHD (44). Late invasive mold infections may occur, particularly in those with GVHD and preceding viral (CMV) infection. About one-third of patients with chronic GVHD may develop recurrent infection with encapsulated bacteria (sinopulmonary, bacteremia) (45). The predominant pathogen is Streptococcus pneumoniae (often with antimicrobial resistance) as well as Haemophilus influenzae and S. aureus. This risk is related to a deficit in opsonizing antibody (4,36,40,44–52).
The Patient Treated with Immunomodulatory Agents
There are a growing number of immunomodulatory agents, generally targeting specific cell populations and cytokines, commonly used in clinical practice (53). As more patients are treated with these therapies and with longer-term follow-up, understanding of the risk of infection associated with these biologic compounds will be refined.
Tumor necrosis factor-α (TNF-α) antagonists are effective in the treatment of rheumatoid arthritis, active inflammatory bowel disease, psoriasis, and ankylosing spondylitis, and are amongst the best characterized in terms of infectious risk. There are currently five marketed in the United States: Infliximab (Remicade, Centocor Inc.), etanercept (Enbrel, Amgen and Wyeth Pharmaceuticals), adalimumab (Humira, Abbott), certolizumab pegol (Cimzia, UCB Inc.), and golimumab (Simponi, Janssen). Blockade of TNF-α, a proinflammatory cytokine, results in improvement in systemic inflammatory conditions; however, TNF-α, along with interferon-γ and other cytokines, is an important component in maintaining cellular immunity and, in animal studies, preventing bacterial deep tissue infections.
Tuberculosis has been associated with use of TNF-α antagonists due to cell-mediated immune deficits (54,55). Rates are three- to fourfold higher with adalimumab and infliximab compared to etanercept (56,57). In general, cases have occurred in those with risk factors for latent tuberculosis infection. As a result, tuberculosis skin testing (TST) or interferon-gamma-release assay (IGRA) and chest radiography are recommended in patients prior to the initiation of a TNF-α antagonist as well as the newer biologic agents. Regardless of TST or IGRA data, tuberculosis (and nontuberculous mycobacterial infections) should be considered in the differential diagnosis in a patient presenting with compatible symptoms and after a TNF-α antagonist. Infections with fungi (histoplasmosis, aspergillosis, coccidiomycosis, and candidiasis), bacteria (listeriosis, nocardiosis), parasites (leishmaniasis), and viruses (herpes zoster, hepatitis B and C reactivation) have also been associated with the use of TNF-α antagonists. In 2008 the FDA issued a black box warning regarding endemic mycoses and TNF-α antagonists, although the exact degree of risk appears to vary based on intensity of exposure (55).
Rituximab (Rituxan, Genentech Inc. and Biogen Idec) is a chimeric murine/human monoclonal antibody to the CD20 epitope expressed on B lymphocytes but not plasma cells. Treatment with rituximab results in rapid depletion of circulating CD20+ B cells. This agent is approved for the treatment of CD20+ B-cell lymphoma, as well as in combination with methotrexate in rheumatoid arthritis. Rituximab has also been used for the treatment of PTLD, immune thrombocytopenic purpura, autoimmune hemolytic anemia, systemic lupus erythematosus, multiple sclerosis, GVHD, and treatment of antibody-mediated graft rejection (58). Following rituximab therapy, antibody production is maintained by plasma cells. Peripheral B-cell recovery takes 3 to 12 months (59). Approximately 5% of people can develop persistent hypogammaglobulinemia after rituximab treatment, increasing infectious risk (60–62). Fatal hepatitis B reactivation (63,64) and progressive multifocal leukoencephalopathy (PML), a demyelinating disease of the CNS caused by human JC polyomavirus, have been associated with rituximab (65).
Numerous other biologic agents are currently approved or undergoing study (53). Table 90.3 summarizes selected approved agents, mechanism of action, associated infectious risks, and approved indications.
DIAGNOSIS
The signs and symptoms of infection are often muted in immunocompromised hosts with infectious syndromes (66). Minor complaints may be the only clues to localize infections. A physical examination should be completed, with particular focus on organ systems commonly involved with infectious complications including the skin, respiratory tract including sinuses, CNS, and urinary tract. Cutaneous lesions may be the earliest manifestation of disseminated infection. Examination of the skin should include the perirectal area, looking for evidence of erythema or tenderness; this is a common site of infection and source of fever notably in neutropenic patients.
In neutropenic patients, fever, defined as a single oral temperature of 38.3°C (101°F) or higher or a temperature of 38°C (100.4°F) or higher for an hour or more, may be the only indication of infection (6). About 50% of neutropenic patients with fever have a documented infection, and about 20% of those with neutrophil counts less than 100 cells/μL have bacteremia (2–7,9,10,67,68). Up to 50% of neutropenic patients with a normal chest radiograph and fever lasting 2 days, despite empiric antibiotic therapy, will have findings on chest CT suggestive of pneumonia that were not appreciated on plain radiograph (69). A daily search for subtle signs and symptoms of infection should be undertaken if unexplained fever persists.
TABLE 90.3 Selected Biologic Agents, Mechanisms of Action, Food and Drug Administration (FDA)-Approved Indications and Associated Infections | |||
![]() |
Basic investigations include a CBC with differential, serum creatinine, liver enzymes, and liver function tests in addition to cultures of blood, urine, and sputum prior to antimicrobial therapy. A chest radiograph should be performed and, if normal, a CT scan should be obtained in the patient with pulmonary symptoms. Collection of additional specimens is guided by the clinical presentation and preliminary investigations (e.g., stool cultures and examination for parasites and C. difficile toxin, blood for CMV nucleic acid testing [NAT], respiratory viral studies, viral swabs for HSV, and VZV from skin lesions).
Delays in appropriate therapy may compromise outcome necessitating an aggressive approach to making a specific microbiologic diagnosis. Based upon the clinical stability of the patient, the severity of immune deficits, and the most likely cause of infection, the physician may initiate empiric therapy while awaiting the results of investigations, or therapy may be deferred until clinical data become available. Increasingly, infections in compromised hosts are due to organisms with antimicrobial resistance patterns that make selection of empiric therapy more difficult. Compromised hosts have an increased susceptibility to community-acquired organisms (MRSA, extended-spectrum beta lactamase (ESBL) producing gram negatives and multi–drug-resistant Pneumococcus) and nosocomial pathogens (VRE, fluconazole-resistant Candida species and carbapenem-resistant Enterobacteriaceae). All microbiologic isolates require susceptibility testing in immunocompromised patients. Consultation with an infectious diseases specialist may be useful to assist in decisions regarding empiric therapy and for guidance regarding appropriate investigations, specimen collection, and transport.
Whenever tissue or body fluids are collected, appropriate histologic and microbiologic investigations should be performed, and consultation with the pathologist and/or microbiologist is recommended to ensure appropriate testing. Diagnosis of many pathogens that cause disease in immunocompromised hosts requires special stains (e.g., modified acid-fast stain for Nocardia, silver or immunofluorescent stains for P. jirovecii) or culture media (e.g., for Mycobacteria species). In addition, given that noninfectious etiologies such as organ rejection, drug toxicity, and GVHD are often in the differential diagnosis, histology is integral to making a definitive diagnosis and invasive diagnosis should be considered early in the patient’s course. The diagnosis of virally mediated diseases such as tissue-invasive CMV (70) and EBV-associated PTLD (71) may require histology for diagnosis.
TREATMENT
The Neutropenic Patient
After appropriate microbiologic studies, empiric antimicrobial therapy is indicated in neutropenic patients at the onset of fever or, in the case of suspected infection, without fever (6). In critically ill neutropenic patients, there is no single empiric regimen appropriate for all patients (6,72–74). The selection of an initial empiric antibiotic regimen should take into consideration the general trend of increasing gram-positive infections, the local hospital epidemiology, including the susceptibility patterns of isolates from neutropenic patients, in addition to the clinical presentation, epidemiologic exposures, and prior antimicrobial use.
Options include monotherapy with (a) a third- or fourth-generation cephalosporin (e.g., ceftazidime or cefepime), (b) an antipseudomonal carbapenem such as imipenem or meropenem, or (c) piperacillin–tazobactam. Dual therapy, such as an antipseudomonal β-lactam plus an aminoglycoside or fluoroquinolone may be used or, for inpatients with recent surgery or vascular access catheters, a glycopeptide such as vancomycin can be combined with one- or two-drug therapy.
The initial empiric addition of vancomycin therapy in febrile neutropenia has not been shown to alter outcomes in patients without pulmonary infiltrates, septic shock, clinically documented infections likely due to gram-positive organisms such as CVC or skin and soft tissue infections, or documented gram-positive infections resistant to the primary empiric therapy (75). Vancomycin use has also been associated with the emergence of vancomycin-resistant enterococci; its use in febrile neutropenic patients should be limited as indicated above.
For those with an identified source of infection, usually less than half of patients, antimicrobial therapy can be tailored based on culture results. Those who defervesce on empiric antibacterial therapy should have the antimicrobials continued to complete a therapeutic course appropriate for the defined infection and until neutrophil recovery.
Controversy persists regarding the optimal timing of adding antifungal therapy. In patients who have been in the ICU for more than 5 to 7 days and have been hypotensive or otherwise critically ill, anti-Candida therapy may be added after cultures are obtained (76,77). In others who have failed to defervesce on empiric antibiotic therapy after 5 to 7 days, and in whom no source of infection is identified, there is a high risk of systemic fungal infection, and empiric antifungal therapy should be added (5–7,72). Amphotericin B is the historical gold standard for empiric therapy in this setting; however, lipid products of amphotericin B (e.g., liposomal amphotericin B [AmBisome, Astellas] and amphotericin B lipid complex [Abelcet, Elan]) have similar efficacy with less toxicity (78). In the United States, liposomal amphotericin or echinocandins (caspofungin (79)) are approved for empiric therapy of fever in neutropenia. Other agents may have efficacy such as voriconazole (80), other echinocandins (e.g., anidulafungin and micafungin), and posaconazole (81). Renal and hepatic function, potential drug interactions, cost, and suspected source of fungal infection are all considerations when choosing an initial empiric antifungal agent. In those with confirmed invasive aspergillosis, voriconazole is the drug of choice although no direct clinical trial comparison with liposomal amphotericin has been performed. Limited data suggest that combination therapy with these agents with an echinocandin may improve outcomes in highly selected patients (52,82,83).
Although the use of hematopoietic growth factors such as granulocyte colony-stimulating factor (G-CSF) increase the neutrophil count, they have not been shown to have benefit in the management of febrile neutropenia and routine use is not recommended (84,85).
TABLE 90.4 Treatment Modalities for Common Opportunistic Infections | |||
![]() |
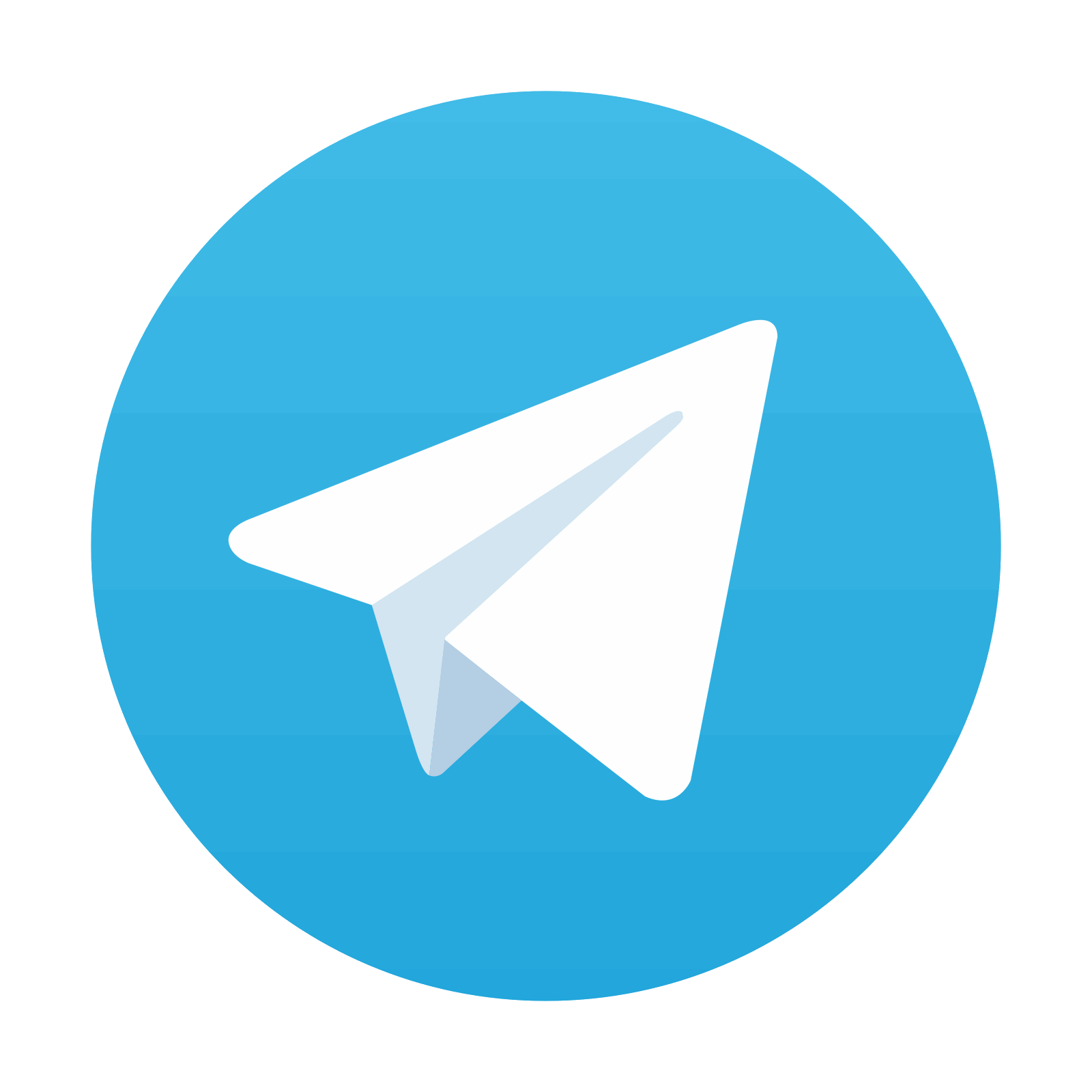
Stay updated, free articles. Join our Telegram channel

Full access? Get Clinical Tree
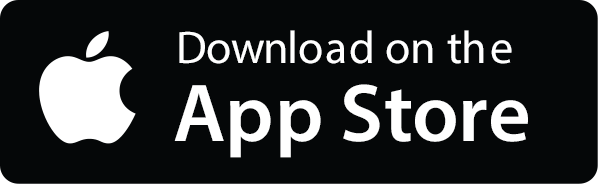
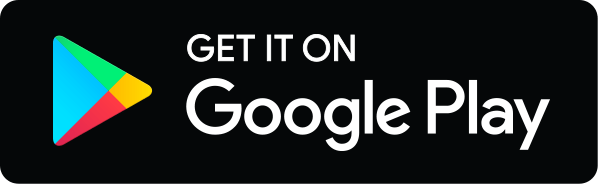