Indications and Options for Mechanical Ventilation
KEY POINTS
1 Prime indications for initiating mechanical ventilation include inadequate alveolar ventilation, inadequate arterial oxygenation, excessive respiratory workload, and acute heart failure with labored breathing.
2 For machine-aided breathing cycles, the physician must determine the minimum frequency of the machine’s inspiratory cycle, the pressure or tidal volume to administer, the inspired oxygen fraction, the triggering sensitivity and the levels of certain boundary conditions (e.g., end-expiratory pressure and alarm limits).
3 Positive-pressure inflation can be achieved with machines that control either of the two determinants of ventilating power—pressure or flow—and terminate inspiration according to pressure, flow, volume, or time criteria. Both pressure and flow cannot be fixed simultaneously because once either is set, the other becomes a dependent variable influenced by the interaction of the inflation impedance with the controlled variable.
4 The fundamental difference between pressuretargeted and volume-targeted ventilation is implicit in their names. Strictly pressuretargeted modes regulate pressure at the expense of letting flow and tidal volume vary; volume-targeted modes guarantee flow and/or tidal volume but let airway pressure vary.
5 Standard modes of positive-pressure ventilation include assist/control ventilation, SIMV, and PSV. The first two can be applied using either flow-controlled or pressurecontrolled machine cycles.
6 Potentially useful ventilatory options include pressure regulated volume control, automatic tube compensation, airway pressure release ventilation, and Bi-Phasic Airway pressure. Promising newer modes include adaptive support ventilation, proportional assist ventilation, and neurally adjusted ventilatory assist. Each of these innovations is intended to combine desirable features of pressure preset and flowcontrolled, volume-targeted ventilation.
7 High frequency ventilation may be useful for those patients who have a bronchopleural fistula, a specialized need for ventilatory support or refractory hypoxemia with high risk for ventilator-induced lung injury. Deep sedation is generally required.
8 Certain adjuncts to mechanical ventilation, including permissive hypercapnia and prone positioning, are now widely applied in clinical practice. The value of other methods, such as inhaled nitric oxide, tracheal gas insufflation, partial liquid ventilation, and extrapulmonary gas exchange, is yet unproved.
9 Noninvasive ventilation seems to be particularly helpful when initiated early in the course of rapidly reversible diseases that respond to modest airway pressures. Good examples are exacerbated COPD and congestive heart failure. It is less often successful for patients whose condition has already deteriorated, for patients who are comatose or noncooperative, and for patients who either cannot be attended closely or are hemodynamically unstable.
▪ INDICATIONS FOR MECHANICAL VENTILATION
Although often made concurrently, the decisions to institute mechanical support should be made independently of those made to perform tracheal intubation or to use positive end-expiratory pressure (PEEP) or continuous posture airway pressure (CPAP). This is especially true considering the recently improved noninvasive (nasal and mask) options and interfaces for supporting ventilation. As the ventilator assumes the work of breathing (WOB), important changes occur in
pleural pressure, ventilation distribution, and cardiac output (Fig. 7-1). Mechanical assistance may be needed because oxygenation cannot be achieved with an acceptable FiO2 without manipulating PEEP, mean airway pressure, and pattern of ventilation or because spontaneous ventilation places excessive demands on ventilatory muscles or on a compromised cardiovascular system (Table 7-1).
pleural pressure, ventilation distribution, and cardiac output (Fig. 7-1). Mechanical assistance may be needed because oxygenation cannot be achieved with an acceptable FiO2 without manipulating PEEP, mean airway pressure, and pattern of ventilation or because spontaneous ventilation places excessive demands on ventilatory muscles or on a compromised cardiovascular system (Table 7-1).
Inadequate Alveolar Ventilation
Apnea and deteriorating ventilation despite other therapeutic measures are absolute indications for mechanical breathing assistance. Usually in such cases there are signs of respiratory distress or advancing obtundation, and serial blood gas measurements show a falling pH and stable or rising PaCO2. Although few physicians withhold mechanical assistance when the pH trends steadily downward and there are signs of physiologic intolerance, there is less agreement regarding the absolute values of PaCO2 and pH that warrant such intervention; these clearly vary with the specific clinical setting. In fact, after intubation has been accomplished, pH and PaCO2 may be allowed to drift deliberately far outside the normal range to avoid the high ventilating pressures and tidal volumes that tend to induce lung damage. This strategy—permissive hypercapnia—is now considered integral to a lungprotective ventilatory approach for the acute management of severe asthma and adult acute respiratory distress syndrome (ARDS). Acute hypercapnia has well-known and potentially adverse physiologic consequences. Nonetheless, recent experimental work in varied models of clinical problems—notably, ischemia/reperfusion and ventilator-induced lung injury—clearly indicate that certain forms of cellular injury are actually attenuated by hypercapnia. Whether it is hypercapnia itself or the associated change in hydrogen ion concentration is still a subject of active investigation (ARDS; see Chapter 24).
TABLE 7-1 INDICATIONS FOR MECHANICAL VENTILATION | |||||
---|---|---|---|---|---|
|
Blood pH is generally a better indicator than PaCO2 of the need for ventilatory support. Hypercapnia per se should not prompt aggressive intervention if pH remains acceptable and the patient remains alert, especially if CO2 retention occurs slowly. Many patients require ventilatory assistance despite levels of alveolar ventilation that would be appropriate for normal resting metabolism. For example, patients with metabolic acidosis and neuromuscular weakness or airflow obstruction may lower PaCO2 to 40 mm Hg or below but not sufficiently to prevent acidemia. The physiologic consequences of altered pH are still debated and clearly depend on the underlying pathophysiology and comorbidities. However, if not quickly reversible by simpler measures, a sustained pH greater than 7.65 or less than 7.10 is often considered sufficiently dangerous in itself to require control by mechanical ventilation and sedation (with muscle relaxants, if needed). Inside these extremes, the threshold for initiating support varies with the clinical setting. For example, a lethargic patient with asthma who is struggling to breathe can maintain a normal pH until shortly before suffering a respiratory arrest, whereas an alert cooperative patient with chronically blunted respiratory drive may allow pH to fall to 7.25 or lower before recovering uneventfully in response to aggressive
bronchodilation, steroids, and oxygen. In less obvious situations, the decision to ventilate should be guided by trends in pH, arterial blood gases, mental status, dyspnea, hemodynamic stability, and response to therapy. The ongoing need for ventilatory assistance must be carefully and repeatedly assessed (see Chapter 10).
bronchodilation, steroids, and oxygen. In less obvious situations, the decision to ventilate should be guided by trends in pH, arterial blood gases, mental status, dyspnea, hemodynamic stability, and response to therapy. The ongoing need for ventilatory assistance must be carefully and repeatedly assessed (see Chapter 10).
▪ INADEQUATE OXYGENATION
Arterial oxygenation is the result of complex interactions between systemic oxygen demand, cardiovascular adequacy, and the efficiency of pulmonary oxygen exchange. Improving cardiovascular performance and minimizing O2 consumption (by reducing fever, agitation, pain, etc.) may dramatically improve the balance between delivery and consumption. Transpulmonary oxygen exchange can be aided by supplementing FiO2 by using PEEP, by changing the pattern of ventilation to increase mean airway (and consequently, mean alveolar) pressure and average lung size (see Chapter 5), or by prone positioning. In patients with edematous or injured lungs, relief of an excessive breathing workload may improve oxygenation by relaxing the expiratory muscles (improving end-expiratory lung distention) and by allowing the mixed venous O2 saturation and venous admixture to improve.
Modest fractions of inspired oxygen are administered to nonintubated patients using masks or nasal cannulas. Controlled, low-range O2 therapy is best delivered to the nonintubated patient by a well-fitting Venturi mask, which automatically adjusts to changes in inspiratory flow demand without change in delivered FiO2. Without tracheal intubation, delivery of high FiO2 can only be achieved with a snug nonrebreathing mask that is flushed with high flows of pure O2. Unfortunately, apart from the risk of O2 toxicity, masks often become displaced or must be removed intentionally for eating or expectoration. Intubation facilitates the application of PEEP and CPAP needed to avert oxygen toxicity as well as enables extraction of airway secretions.
Although positive airway pressure (noninvasive ventilation [NIV] or CPAP) can be applied to spontaneously breathing, nonintubated patients, these techniques may not be well tolerated for extended periods, especially by confused, poorly cooperative, or hemodynamically unstable patients who require high mask pressures (>15 cm H2O; see “Noninvasive Ventilation”, following). Patients who retain secretions also are poor candidates for these techniques. Moreover, with the airway unprotected, these methods should be used only with extreme caution in patients who are obtunded or comatose. Continuous positive airway pressure is best tolerated at low levels (<10 cm H2O) for less than 48 h, with sporadic breaks allowed to relieve facial pressure.
Excessive Respiratory Workload
A common reason for mechanical assistance is a lack of ventilatory power or reserve. The respiratory muscles cannot sustain tidal pressures greater than 40% to 50% of their maximal isometric pressure. Respiratory pressure requirements rise with minute ventilation and the impedance to breathing. Patients with hypermetabolism or metabolic acidosis often need ventilatory support to avoid decompensation. Impaired ventilatory drive or muscle strength diminish ventilatory capacity and reserve.
Cardiovascular Support
Although little effort is expended by normal subjects who breathe quietly, the O2 demands of the respiratory system account for a very high percentage of total body oxygen consumption ([V with dot above]O2) during periods of physiologic stress (Fig. 7-2). Experimental animals in circulatory shock who receive mechanical ventilation survive longer than their unassisted counterparts. Moreover, patients with combined cardiorespiratory disease often fail attempts to withdraw ventilatory support for cardiac rather than respiratory reasons. Such observations demonstrate the importance of minimizing the ventilatory O2 requirement during cardiac insufficiency or ischemia. Doing so helps rebalance myocardial O2 supply with requirements and/or allows diaphragmatic blood flow to be redirected to other O2-deprived vital organs. Moreover, reducing ventilatory effort may improve afterload to the left ventricle (see Chapter 1). Therefore, the physician should intervene early to relieve an excessive breathing workload for patients with compromised cardiac function. Although it is possible to use NIV or CPAP alone for mildly to moderately affected patients, fatigue often sets in unless underlying oxygen requirements are reduced substantially, which often requires adequate sedation or higher pressures than can be provided noninvasively.
▪ OPTIONS IN MECHANICAL VENTILATION
Types of Ventilation
Negative Versus Positive Pressure
To accomplish ventilation, a pressure difference must be developed phasically across the passive lung. This difference can be generated by negative pressure developed by respiratory muscles in the pleural space, by positive pressure applied to the airway opening, or by a combination of both. Although of major historical interest, negative-pressure ventilators are seldom appropriate for the modern acute care setting and will not be discussed further. Whether using negative or positive pressure for machine-aided cycles, the physician must determine the machine’s minimum cycling rate, the duration of its inspiratory cycle, the baseline pressure (PEEP), and either the pressure to be applied or the tidal volume to administer, depending on the “mode” selected.
Positive-Pressure Ventilation
Positive-pressure inflation can be achieved with machines that control either of the two determinants of ventilating power—pressure or flow—and terminate inspiration according to pressure, flow, volume, or time limits. Waveforms for both flow and pressure cannot be controlled simultaneously, however, because pressure is developed as a function of flow and the impedance to breathing, which is determined by the uncontrolled parameters of resistance and compliance. Thus, the clinician has the choice of specifying pressure, with tidal volume as a resulting (dependent) variable, or of controlling flow, with pressure as the dependent variable. Whereas older ventilators offered only a single control variable and a single cycling criterion, positive-pressure ventilators of the latest generation enable the physician to select freely among multiple options.
Pressure-Cycled Ventilation
Although pressure-cycled (pressure-limited) ventilators generally have been supplanted by more advanced machines that offer multiple modes with different cycling criteria (time or flow), some are still used, especially in economically depressed regions or developing countries. In their simplest form, these low-cost machines allow gas to flow continuously until a set pressure limit is reached. A pressurized gas source is all that is required to operate many of these machines, making them immune to electrical failure. Small size, portability, and low cost make this now obsolete equipment acceptable for low-demand applications in transport and in respiratory therapy, where they can be used for intermittent delivery of aerosols or for deep breathing (intermittent positive pressure breathing [IPPB]).
Pressure-Preset (Pressure-Targeted) Ventilation
Modern ventilators provide pressure-preset or pressure-targeted ventilatory modes (e.g., pressure control or pressure support) as options for full or partial ventilatory assistance. After the breath is initiated, these modes quickly attain a targeted amount of pressure at the airway opening until a
specified time (pressure control) or flow (pressure support) cycling criterion is met (Fig. 7-3). Maximal pressure is controlled, but tidal volume is a complex function of applied pressure and its rate of approach to target pressure, available inspiratory time, and the impedance to breathing (compliance, inspiratory and expiratory resistance, and auto-PEEP). High flow capacity, pressure-targeted ventilation compensates well for small air leaks and is therefore quite appropriate for use with leaking or uncuffed endotracheal (ET) tubes, as in neonatal or pediatric patients. Because of its virtually “unlimited” ability to deliver flow and its decelerating flow profile, pressure-targeted ventilation also is an appropriate choice for spontaneously breathing patients with high or varying inspiratory flow demands, which usually peak early in the ventilatory cycle. The decelerating flow profiles of pressure-targeted modes also improve the distribution of ventilation in lungs with heterogeneous mechanical properties (widely varying time constants). Apart from their potential to limit the lung’s exposure to high airway pressure and risk for barotrauma, pressure-targeted modes also prove helpful for adult patients in whom the airway cannot be completely sealed (e.g., bronchopleural fistula).
specified time (pressure control) or flow (pressure support) cycling criterion is met (Fig. 7-3). Maximal pressure is controlled, but tidal volume is a complex function of applied pressure and its rate of approach to target pressure, available inspiratory time, and the impedance to breathing (compliance, inspiratory and expiratory resistance, and auto-PEEP). High flow capacity, pressure-targeted ventilation compensates well for small air leaks and is therefore quite appropriate for use with leaking or uncuffed endotracheal (ET) tubes, as in neonatal or pediatric patients. Because of its virtually “unlimited” ability to deliver flow and its decelerating flow profile, pressure-targeted ventilation also is an appropriate choice for spontaneously breathing patients with high or varying inspiratory flow demands, which usually peak early in the ventilatory cycle. The decelerating flow profiles of pressure-targeted modes also improve the distribution of ventilation in lungs with heterogeneous mechanical properties (widely varying time constants). Apart from their potential to limit the lung’s exposure to high airway pressure and risk for barotrauma, pressure-targeted modes also prove helpful for adult patients in whom the airway cannot be completely sealed (e.g., bronchopleural fistula).
Flow-Controlled, Volume-Cycled Ventilation
For many years, flow-controlled, volume-cycled ventilation (assist-control) has been the technique of choice for supporting seriously ill adult patients. Flow can be controlled by selecting a waveform (e.g., constant or decelerating) and setting a peak flow value or by selecting a flow waveform and setting the combination of tidal volume and inspiratory time. By controlling the tidal volume and “backup” frequency, a lower limit for minute ventilation can be guaranteed. Unfortunately, there are two important trade-offs of controlling flow. First, the pressure required to ventilate with any given PEEP and tidal volume varies widely with the impedance to breathing (Fig. 7-3). Moreover, once the peak and profile are chosen, flow remains inflexible to increased (or decreased) inspiratory flow demands.
Differences between Pressure-Targeted and Volume-Targeted Ventilation
After the decision has been made to initiate mechanical ventilation, the physician must decide to use either pressure-controlled ventilation (PCV) or volume-cycled ventilation. For a well-monitored passively ventilated patient, pressure-targeted and volume-targeted modes can be used with virtually identical benefit and risk. With either method, FiO2, PEEP, and backup frequency must be selected. If pressure control (sometimes referred to as pressure assist control) is used, the targeted inspiratory pressure (above PEEP) and the inspiratory time must be selected (usually with consideration toward the desired tidal volume). Pressure support differs from pressure control in that each pressuresupported breath must be patient initiated (triggered). Furthermore, the off-cycling criterion for pressure support is flow, rather than time, so that cycle length is free to vary with patient effort.
If volume-cycled ventilation is used, the physician may select (depending on ventilator) either tidal volume and flow delivery pattern (waveform and peak flow) or flow delivery pattern and minimum minute ventilation (with tidal volume the resulting quotient of VE and backup frequency).
If volume-cycled ventilation is used, the physician may select (depending on ventilator) either tidal volume and flow delivery pattern (waveform and peak flow) or flow delivery pattern and minimum minute ventilation (with tidal volume the resulting quotient of VE and backup frequency).
TABLE 7-2 PRESSURE-CONTROLLED VERSUS VOLUME-CONTROLLED VENTILATION | ||||||||||||
---|---|---|---|---|---|---|---|---|---|---|---|---|
|
The fundamental difference between pressureand volume-targeted ventilation is implicit in their names; pressure-targeted modes guarantee pressure at the expense of letting tidal volume vary, and volume-targeted modes guarantee flow—and consequently the volume provided to the circuit in the allowed inspiratory time (tidal volume)—at the expense of letting airway pressure float. This distinction governs how they are used in clinical practice (Table 7-2).
Flow and tidal volume are important variables to monitor when pressure targeting, whereas airway pressure is of parallel importance in volume targeting. Gas stored in compressible circuit elements does not contribute to effective alveolar ventilation. For adult patients, such losses (approx. 2 to 4 mL/cm H2O of peak pressure) usually constitute a modest fraction of the tidal volume. For infants, however, compressible losses may comprise such a high fraction of the VT that effective ventilation varies markedly with peak cycling pressure. Thus, during volume-cycled ventilation, moment-by-moment changes in chest impedance caused by bronchospasm, secretions, and muscular activity can force peak airway pressure and compressible circuit volume to rise and effective tidal volume to fall. Finally, because airway pressure is controlled, pressure-targeted modes are less likely to cause barotrauma, which is a particularly serious problem for this age group.
Volume-targeted modes deliver a preset volume unless a specified circuit pressure limit is exceeded. Major advantages to volume targeting are the capacity to deliver unvarying tidal volumes (except in the presence of a gas leak), flexibility of flow and volume adjustments, and power to ventilate difficult patients. All ventilators currently used for continuous support in adults offer volume cycling as a primary option. Despite its advantages for acute care, volume cycling also has important disadvantages. Unless the airway is well sealed, volume-cycled modes cannot ventilate effectively and consistently. Furthermore, after the flow rate and profile are set, the inflation time of the machine remains fixed and remains unresponsive to the patient’s native cycling rhythm and flow demands. Just as importantly, excessive alveolar pressure may be required to deliver the desired tidal volume. Pressure-targeted modes confer flexibility to flow demand and, when flow cycled, to cycle duration as well.
Standard Modes of Positive Pressure
Controlled Mechanical Ventilation
With the sensitivity adjustment turned off during controlled mechanical ventilation (CMV), the machine provides a fixed number of breaths per minute and remains totally uninfluenced by the patient’s efforts to alter frequency. This “lockout” mode demands constant vigilance to make appropriate adjustments for changes in ventilatory requirements and is used only for situations in which pH and/or PaCO2 must be controlled tightly (e.g., some neurologic patients). Most patients require deep sedation to ensure comfort and to ablate breathing efforts. Under these conditions, assist-control ventilation has similar capability and offers additional advantages (less patient-ventilator asynchrony).
Assist-Control Ventilation
During assist-control ventilation (or assisted mechanical ventilation [AMV]), each inspiration triggered by the patient is powered by the ventilator using either volume-cycled or pressure-targeted breaths (Fig. 7-4). When pressure is the targeted variable and inspiratory time is preset, the mode is
known as pressure control or pressure assist control. Sensitivity to inspiratory effort can be adjusted to require a small or large negative pressure deflection below the set level of end-expiratory pressure to initiate the machine’s inspiratory phase. Alternatively, modern machines can be flow triggered, initiating a cycle when a flow deficit is sensed in the expiratory limb of the circuit relative to the inspiratory limb during the exhalation period. As a safety mechanism, a “backup rate” is set so that if the patient does not initiate a breath within the number of seconds dictated by that frequency, a machine cycle begins automatically. A backup rate set high enough to cause alkalosis blunts respiratory drive and terminates the patient’s efforts to breathe at the “apneic threshold” for PaCO2. In awake, normal subjects, this threshold usually is achieved when PaCO2 is abruptly lowered to 28 to 32 mm Hg; it may be considerably higher during sleep or when sedating drugs are given. Note that unlike CMV or synchronized intermittent mandatory ventilation (SIMV; see following), changes in set machine frequency during AMV have no effect on [V with dot above]E unless this “backup” frequency is set high enough to terminate the patient’s own respiratory efforts. Hence, AMV is not an appropriate mode for weaning.
known as pressure control or pressure assist control. Sensitivity to inspiratory effort can be adjusted to require a small or large negative pressure deflection below the set level of end-expiratory pressure to initiate the machine’s inspiratory phase. Alternatively, modern machines can be flow triggered, initiating a cycle when a flow deficit is sensed in the expiratory limb of the circuit relative to the inspiratory limb during the exhalation period. As a safety mechanism, a “backup rate” is set so that if the patient does not initiate a breath within the number of seconds dictated by that frequency, a machine cycle begins automatically. A backup rate set high enough to cause alkalosis blunts respiratory drive and terminates the patient’s efforts to breathe at the “apneic threshold” for PaCO2. In awake, normal subjects, this threshold usually is achieved when PaCO2 is abruptly lowered to 28 to 32 mm Hg; it may be considerably higher during sleep or when sedating drugs are given. Note that unlike CMV or synchronized intermittent mandatory ventilation (SIMV; see following), changes in set machine frequency during AMV have no effect on [V with dot above]E unless this “backup” frequency is set high enough to terminate the patient’s own respiratory efforts. Hence, AMV is not an appropriate mode for weaning.
Synchronized Intermittent Mandatory Ventilation
During SIMV, the intubated patient is connected to a single circuit that allows both spontaneous breathing and a set number of mechanical cycles timed to coincide with spontaneous effort. Ventilator breaths— volume cycled or pressure controlled—are interspersed to supplement spontaneous ventilation, which is usually pressure supported. Because SIMV can provide a wide range of ventilatory support, it can be used either as a full support mode or as a weaning mode, depending on the mandatory frequency selected.
Pressure-Support Ventilation
Description
Pressure-support ventilation (PSV) is a method with which each breath taken by a spontaneously breathing patient receives a pressure boost. After the breath is initiated, pressure builds rapidly toward an inspiratory pressure target. Airway pressure is then maintained constant at the level set by the clinician until flow decays to satisfy the machine’s “off-switch” criterion. At relatively slow respiratory rates, the resulting airway pressure profile resembles a “square” wave. Because PSV is flow cycled (and therefore influenced by pleural pressure), the patient retains control of cycle length and tidal volume. Modern ventilators allow the clinician to modify the aggressiveness with which the pressure target is approached as well as the flow off-switch criterion to span the full spectrum of patient mechanics and requirements. PSV provides the basis for a number of other ventilatory modes, such as SIMV and volume-assured pressure support (VAPS).
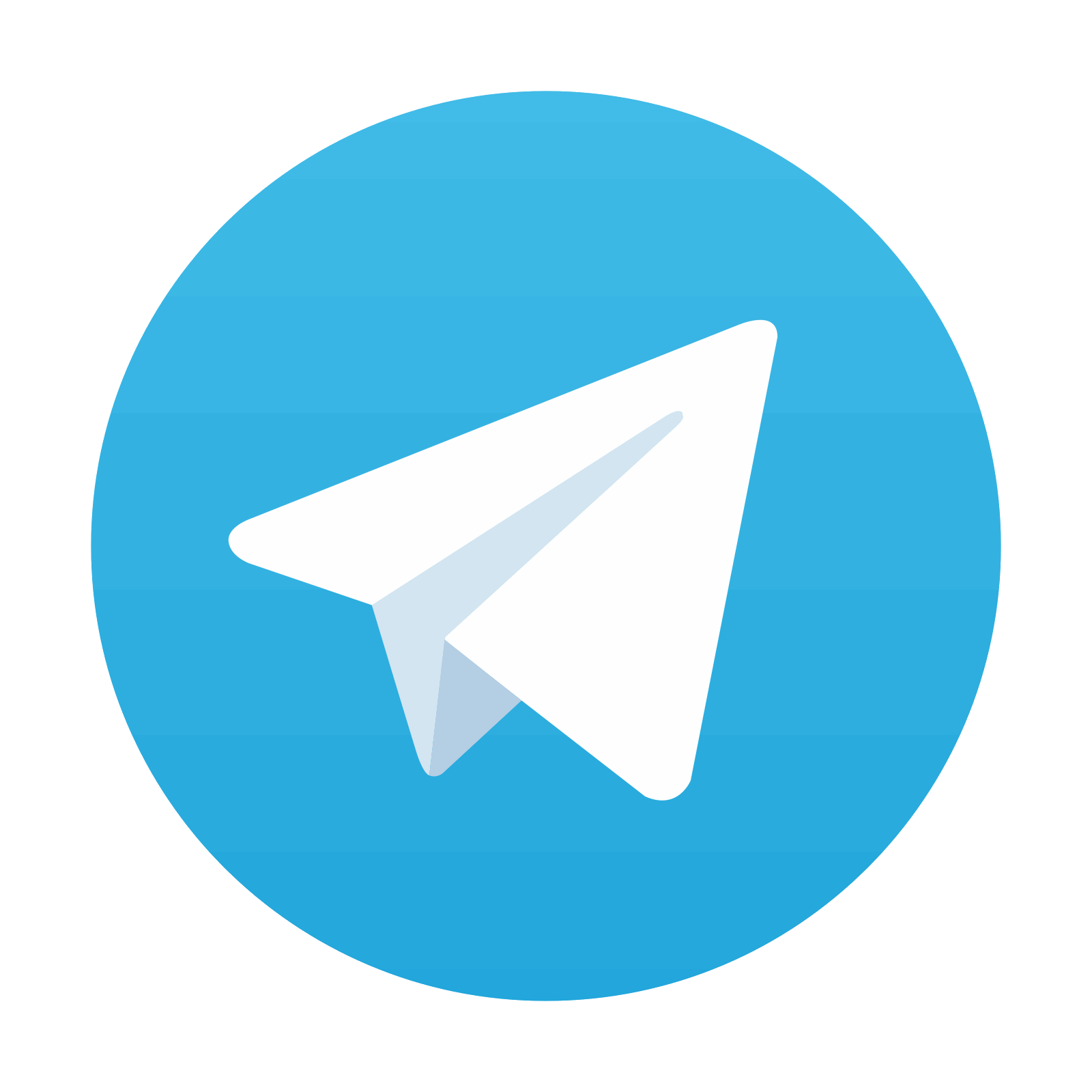
Stay updated, free articles. Join our Telegram channel

Full access? Get Clinical Tree
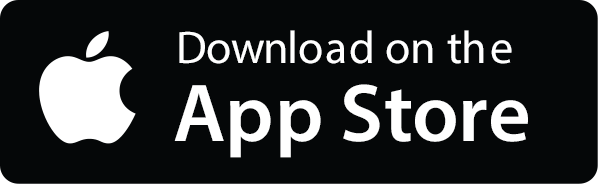
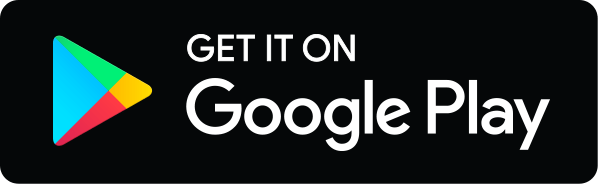
