Perioperative Implications of Obstructive Sleep Apnea
Obstructive sleep apnea (OSA) was first described by novelist Charles Dickens in The Posthumous Papers of the Pickwick Club, published in 1937. The character Joe was an obese boy who was constantly hungry, red in the face, and always falling asleep in the middle of his duties. Dickens [1] writes:
OSA is a common cause of daytime sleepiness for millions of Americans. It is estimated that in adults between the ages of 30 and 60 years, 9% of women and 24% of men have OSA, whereas 2% of women and 4% of men have OSA with daytime sleepiness [2]. The prevalence of OSA increases with age [3]. OSA is associated with an increased incidence of hypertension [4], coronary artery disease [5,6], cardiovascular morbidity and mortality [7], cor pulmonale [8], stroke [9,10], neurocognitive dysfunction [11], motor vehicle accidents [12], and a decreased quality of life [13].
Definition of OSA
OSA is clinically characterized by recurrent episodes of upper-airway obstruction that result in cessation (apnea) or reduction (hypopnea) in airflow during sleep, often accompanied by hypoxia and/or hypercarbia. The diagnosis of OSA is based on the apnea-hypopnea index (AHI), which is a count of the number of apneas and hypopneas per hour of sleep. Apnea is defined as the cessation of airflow for at least 10 seconds in the presence of thoracoabdominal ventilatory efforts and hypopnea as a 50% or more reduction in breathing amplitude. The occurrence of more or less frequent oxygen desaturations or electroencephalography (EEG) arousals is taken as an additional diagnostic tool to identify these conditions [14]. Obstruction is often inferred from thoracoabdominal paradox, the shape of the airflow signal, or when snoring intensity increases during the event [15].
Anatomic Factors
Numerous studies have shown that patients with OSA have structurally narrower and more collapsible airways than patients who do not have OSA who are matched in age and body mass index (BMI, calculated as weight in kilograms divided by the square of height in meters) [16,17]. Craniofacial and upper-airway abnormalities have been shown to predispose patients with OSA to upper-airway obstruction [18]. Micrognathia with an inferior-located hyoid bone has been shown to be a major risk factor for OSA in Japanese patients. This risk factor was most significant in obese patients with large tongues [19]. An anatomic imbalance between upper-airway soft-tissue volume and bony enclosure size may result in pharyngeal airway obstruction during sleep and general anesthesia. Tsuiki and colleagues [20] measured tongue cross-sectional area and craniofacial dimensions through lateral cephalograms in 50 adult male patients with OSA and 55 adult male individuals who did not have OSA and found that when maxillomandibular dimensions were matched, patients with OSA had a significantly larger tongue for a given maxillomandibular size compared with individuals who did not have OSA. Schwab and colleagues [21] used volumetric analysis techniques with magnetic resonance imaging to study the upper-airway soft-tissue structures in sleep apnea and control individuals with a variety of ethnic backgrounds. These investigators showed that the volume of soft-tissue structure surrounding the upper airway is enlarged in patients with OSA. They also showed a greater risk for sleep apnea the larger the volume of the tongue, lateral pharyngeal walls, and soft tissue.
Effect of Lung Volumes on Pharyngeal Patency
Lung volume normally decreases during sleep [22]. Obesity is associated with further reductions in lung volumes caused by increased elastic resistance and decreased compliance of the chest wall, resulting in reduced total respiratory compliance. Decreased pulmonary compliance leads to decreased expiratory reserve volume, functional residual capacity (FRC), vital capacity, and total lung capacity [23]. Whereas the decrease in FRC is responsible for the development of severe hypoxemia during obstructive episodes in obese patients with OSA, the decrease in lung volumes has been shown to contribute to pharyngeal airway obstruction. Van de Graaff [24] studied the effect of thoracic traction on upper-airway resistance during breathing. In anesthetized dogs with denervated upper airways, thoracic traction generated during inspiration reduced upper-airway resistance significantly. These findings indicate that tonic and phasic forces generated by the thorax can improve upper-airway patency. The increased patency during inspiration cannot be attributed solely to activity of upper-airway muscles. Likewise in humans, Heinzer [25] reported a significant decrease in respiratory events during non-rapid eye movement (REM) sleep in patients with OSA when lung volume was increased by negative extrathoracic pressure during sleep. Small changes in lung volume during sleep in patients with OSA have been shown to cause large changes in the continuous positive airway pressure (CPAP) required to prevent flow limitation in the upper airway [26]. Therefore, lung volume has an important effect on the upper airway in patients with OSA and is one factor that increases the risk of OSA in obese patients. Tagaito and colleagues [27] examined the structural interaction between a passive pharyngeal airway and lung volume before and during lung inflation in 8 anesthetized and paralyzed patients with sleep-disordered breathing. Increasing lung volume by applying negative extrathoracic pressure (thereby leaving the transpharyngeal pressure unchanged) improved pharyngeal collapsibility, especially in obese patients. Increased lung volume during inspiration is believed to provide caudal tracheal traction that increases the longitudinal tension on the upper airway independent of upper-airway muscle activity, causing unfolding of the pharyngeal mucosa and stiffening the airway. Traction on the trachea could be explained as a sum of mediastinal traction and the force generated by changes in intrathoracic pressure [28].
Neuromuscular Control
Neuromuscular control of the upper airway plays an important role in OSA pathogenesis. Because upper-airway dilator muscles are important in maintaining pharyngeal patency, reductions in activity of these muscles may be important in mediating REM-related obstructive apnea. Sauerland and Mitchell [29] showed that the electromyographic activity of the largest upper-airway dilator muscle, the genioglossus (GG), was increased during inspiration and markedly reduced during expiration. Mezzanotte and colleagues [30] reported that patients with OSA have significantly greater basal genioglossal activity during wakefulness compared with age and BMI-matched patients who do not have OSA. However, this augmented activity was significantly reduced during sleep. This finding suggests that the neuromuscular compensation present during wakefulness that is necessary to keep the anatomically compromised airway of patients with sleep apnea open is lost during sleep, leading to airway collapse. These investigators further showed that sleep onset is associated with significantly larger decrements in GG and tensor palatine muscle activity in patients with OSA compared with controls [31]. More recently Eckert and colleagues [32] studied the mechanisms of worsening OSA during REM sleep. When airway resistance and blood gas disturbances were minimized by CPAP, genioglossal activity was reduced during REM sleep to a similar extent in OSA and healthy individuals. This finding suggests a generalized reduction in genioglossal activity during REM, which likely renders individuals who are highly reliant on upper-airway dilator muscles vulnerable to pharyngeal collapse during REM sleep.
Ventilatory Control
Multiple feedback loops exist to control and stabilize breathing by adjusting arterial blood gas tensions [33]. Ventilation is constantly monitored and adjusted to maintain appropriate arterial pH and Pco2. That sleep can affect control of these feedback loops was first reported more than 50 years ago by Bulow [34], who observed that decreases in carbon dioxide sensitivity at sleep onset led to episodes of hypopnea or apnea with breathing instability. Sullivan [35] subsequently reported that suppression of respiratory stimuli (wakefulness, vagal, peripheral, and central chemoreceptors) results in near cessation of ventilation, suggesting that decreased metabolic control during sleep may explain the irregular breathing pattern characteristic of the sleep state. Several recent studies using a loop-gain model to measure respiratory system stability suggest that patients with OSA have less ventilatory stability. Loop gain is an engineering term that describes the stability of a system controlled by negative feedback loops. In the case of respiration, loop gain represents the sensitivity of the negative feedback loop that controls ventilation [36]. Younes and colleagues [37] reported that the chemical control system is more unstable in patients with severe OSA than in patients with milder OSA. In a follow-up study the same investigators confirmed chemical instability in patients with OSA and suggested that the mechanisms responsible for the instability may vary considerably among patients [38]. Wellman and colleagues [36] studied ventilatory instability in the context of airway collapsibility in patients with OSA and found a high correlation between loop gain in patients with pharyngeal closing pressure near atmospheric pressure, suggesting that this group may be highly susceptible to changes in ventilatory stability. However, whether loop gain is a cause or consequence of OSA has not been established. It is likely that a combination of upper-airway anatomic, neuromuscular, and ventilatory control mechanisms accounts for the pathogenesis of OSA.
Morbidity and OSA
Systemic Hypertension
Approximately 50% of patients with OSA are hypertensive and 30% of hypertensive patients have OSA [39]. The most convincing evidence to support OSA as a cause in the development of hypertension comes from epidemiologic data from the Wisconsin Sleep Cohort Study. This study evaluating the association between sleep-disordered breathing and hypertension showed a 3-fold increased risk of developing new-onset hypertension in patients with an AHI of 15 or more. This association was independent of age, gender, BMI, and antihypertensive medications [40]. The Sleep Heart Health Study also showed that sleep-disordered breathing is associated with systemic hypertension in middle-aged adults and older individuals even after controlling for demographics and anthropometric variables (BMI, neck circumference, waist/hip ratio, smoking, and alcohol intake) [4]. Subsequent analysis of the Sleep Heart Health data showed that there is a stronger association between sleep-disordered breathing and hypertension for those aged less than 60 years but no association was found for those more than 60 years of age [41]. In addition to human data, 2 animal models support a causal link between sleep-disordered breathing and increased arterial blood pressure. Fletcher and colleagues [42] showed that rats exposed to intermittent hypocapnic hypoxia for 8 hours each day showed significant increases in blood pressure compared with controls. Brooks and colleagues induced OSA in dogs by intermittent airway occlusion during nocturnal sleep. In this model OSA resulted in acute transient increases in nighttime blood pressure and eventually produced sustained daytime hypertension.
Although the physiologic studies provide strong evidence to support a causal relationship between OSA and hypertension, the mechanism by which OSA causes hypertension is less well established. A likely mechanism of increased arterial pressure is through sustained sympathetic nervous system excitation and vascular remodeling. Hypoxia and hypercapnia secondary to partial or complete closure of the upper airway during sleep result in oxygen desaturation and increased sympathetic nervous system activity in patients with OSA [43,44]. The primary role of the sympathetic nervous system is maintenance and regulation of blood pressure. The baroreceptors respond to stretching of the vessel wall (increased blood pressure) by decreasing sympathetic neural outflow, resulting in vasodilation and decreased blood pressure. Recent studies suggest that in addition to short-term regulation of blood pressure, the sympathetic neural system plays an important role in long-term regulation of blood pressure [45,47].
In addition to activation of the sympathetic nervous system, the repetitive hypoxemia-normoxia cycle results in activation of inflammatory pathways, which may be important in the molecular pathogenesis of cardiovascular disease [48] independent of increased sympathetic activity [49]. Hypoxia is a major stimulus of vascular endothelial growth factor, which is a potent angiogenic cytokine. Vascular endothelial growth factors are especially increased in patients with severe OSA [50,51]. Bokinsky and colleagues [51] showed that patients with OSA also have increased spontaneous platelet aggregation and activation, which may increase the risk of mural thrombus formation.
CPAP has been shown to attenuate the sympathetic response to obstructive respiratory events and to lower nocturnal blood pressure in patients with OSA [52,53], reduce platelet aggregation [51], and reduce vascular endothelial growth factor in patients with nocturnal hypoxia [54]. However, the effect of CPAP on daytime blood pressure is not so well established. Peppard and colleagues [40] found a small but significant reduction in systolic and diastolic blood pressure with therapeutic CPAP. Although these studies suffer from small sample size, with most patients normotensive at baseline, they do support considering OSA when evaluating all hypertensive patients.
Sleep Apnea and Heart Failure
Observational data support the association between OSA and heart failure [55,57]. Sustained respiratory effort against an occluded airway leads to negative intrathoracic pressure as low as -80 cm water. This negative intrathoracic pressure increases cardiac afterload by increasing the left ventricular transmural pressure, resulting in increased left ventricular wall stress/tension and impaired relaxation, causing decreased stroke volume and cardiac output [58,59]. Otto [60] compared cardiac structural and functional changes in obese adults with and without OSA and found that patients with OSA tended toward abnormal right ventricular filling and diastolic dysfunction suggesting that OSA, independent of obesity, may induce cardiac changes that predispose to atrial fibrillation and heart failure. This observation is important because OSA in patients with heart failure is associated with an increased risk of death independent of confounding factors [61]. Treatment of OSA with CPAP has been shown to improve left ventricular function in patients with congestive heart failure [62,63]. This evidence supports the use of CPAP in the perioperative period for patients with heart failure.
Cardiac Arrhythmias
Several studies show an association between OSA and nocturnal arrhythmias. Arrhythmias observed during sleep include atrial fibrillation, bradycardia, nonsustained ventricular tachycardia, sinus arrest, second-degree atrioventricular conduction block, and frequent premature ventricular contractions [64–67]. The recurrent apneic and hypopneic episodes resulting in arterial desaturation, hypercapnia, and increased sympathetic tone may acutely trigger nocturnal dysrhythmias, or chronically affect the electrical conduction system [66,68]. The Sleep Heart Health Study showed that patients with severe OSA (AHI>30 events/h) have a 4-fold increase in prevalence of atrial fibrillation [69]. Kanagala and colleagues [70] showed that treatment with appropriate levels of CPAP lowers the incidence of recurrent atrial fibrillation in patients with OSA following cardioversion. The bradycardic response to obstructive apnea is probably related to intrathoracic pressure swings, parasympathetic activity, hypoxia, and sleep phase. Changes in heart rate depend on a balance of sympathetic and parasympathetic tone. The stimulation of upper-airway receptors may increase parasympathetic activity, causing a decrease in heart rate [68,71]. In a study of nonsurgical patients with OSA, Becker and colleagues [72] found a 7.5% incidence of clinically significant (second- and third-degree atrioventricular block and sinus arrest of greater than 2 seconds’ duration) heart block. The incidence of pathologically significant rhythm disturbances such as supraventricular tachycardia and ventricular arrhythmias is less clear. In addition, some studies found no causal relation between OSA and arrhythmias [73].
OSA and Coronary Artery Disease
Several studies have found a positive association between OSA and ischemic heart disease. Peker and colleagues [5] showed that middle-aged patients with OSA followed for 7 years showed an almost 5-fold increase in risk of developing coronary heart disease independent of age, sex, hypertension, diabetes, and current smoking. In patients without clinical coronary artery disease, the presence and severity of OSA has been shown to be independently associated with the presence and extent of coronary artery calcification [74]. Cardiac sudden death is increased during sleeping hours in patients with OSA and the degree of nocturnal hypoxemia is related to the risk of sudden cardiac death [75]. Kuniyoshi and colleagues [76] found that the diurnal variation in the onset of myocardial infarction (MI) in patients with OSA was strikingly different from the diurnal variation in patients without OSA. Patients with nocturnal onset of MI between 12 midnight and 6 am had a high likelihood of having OSA. These findings suggest that OSA may be a trigger for MI.
Thus, OSA may increase cardiovascular risk by several different mechanisms. Repetitive hypoxemia and hypercapnia causing sympathetic activation, vascular endothelial dysfunction, increased oxidative stress, inflammation, increased platelet aggregation, and metabolic dysregulation are all implicated in the pathophysiology of cardiovascular disease associated with OSA [77].
OSA and Stroke
OSA is both a possible risk factor and a consequence of stroke [78,79]. Habitual snoring alone has been shown to increase the risk of cerebrovascular disease [80,82]. The Wisconsin Sleep Cohort Study reported that moderate OSA (defined as AHI>20) was associated with a 3-fold increment in the risk of developing a stroke [83]. Studies looking at patients following stroke report a 44% to 72% incidence of OSA (defined as AHI>10) [84,85]. Proposed mechanisms for the increased risk of OSA following stroke include hypertension, reduction in cerebral blood flow, altered cerebral blood flow, altered cerebral blood flow autoregulation, impaired endothelial function, accelerated atherogenesis, thrombosis, and paradoxic embolism [86].
Clinical presentation
Boxes 1 and 2 list physical characteristics and signs and symptoms frequently associated with OSA.
Obesity and Neck Circumference
OSA is strongly correlated with obesity. A study of patients presenting for bariatric surgery reported a 71% to 77% prevalence of OSA in patients with a BMI of 35 to 59.9 kg/m2 and 90% prevalence with BMI 60 kg/m2 or greater [87]. Increases in weight have been shown to increase the prevalence of OSA, which is an independent risk factor for developing obesity. Young and colleagues [2] found that the prevalence of OSA increased 4-fold with each increase in the standard deviation of the BMI. In addition, an increase in weight has been shown to worsen OSA. An 11-year longitudinal study of 690 Wisconsin residents measuring the association between weight change and AHI showed that a 10% weight gain predicted an approximate 32% increase in the AHI and a 6-fold increase in the risk for developing moderate to severe OSA. A 10% weight loss predicted a 26% decrease in the AHI [88].
Several studies have reported that neck circumference is a better predictor of obstructive apnea than BMI [89–92]. Horner and colleagues [93] showed that more adipose tissue is present in those areas surrounding the collapsible segment of the pharynx in patients with OSA, compared with equally obese control subjects without OSA. Shelton and colleagues [94] performed magnetic resonance imaging and polysomnography on patients with and without OSA. They reported that all patients had a collection of adipose tissue adjacent to the pharynx, the volume of which correlated with the severity of OSA. Weight loss resulted in fewer apneas and hypopneas and a marked decrease in the pharyngeal adipose tissue volume, suggesting that the volume of pharyngeal adipose tissue is causally related to OSA. Similarly, Mortimer [95] studied fat distribution in obese and nonobese patients with OSA and found that both groups have excess fat deposition, especially anterolateral to the upper airway, when compared with weight-matched controls.
Recent data suggest that intraabdominal body fat distribution may be even more significant than fat accumulation in the pharyngeal region as a predictor of OSA. Shafer [96] reported that regional body fat distribution predicts the presence and severity of OSA, but fat accumulation in the neck and parapharyngeal region are of minor importance. Martinez-Rivera and colleagues [97] showed that truncal obesity was significantly better than BMI at predicting OSA. Vgontzas and colleagues [98] showed that sleep-disordered breathing correlated with the amount of visceral fat, but not BMI, total fat, or subcutaneous fat. Deegan and McNicholas [99] suggested a gender difference in the effect of fat distribution. After controlling for BMI and age, waist circumference correlated more closely with AHI than neck circumference among men, whereas the opposite was true for women.
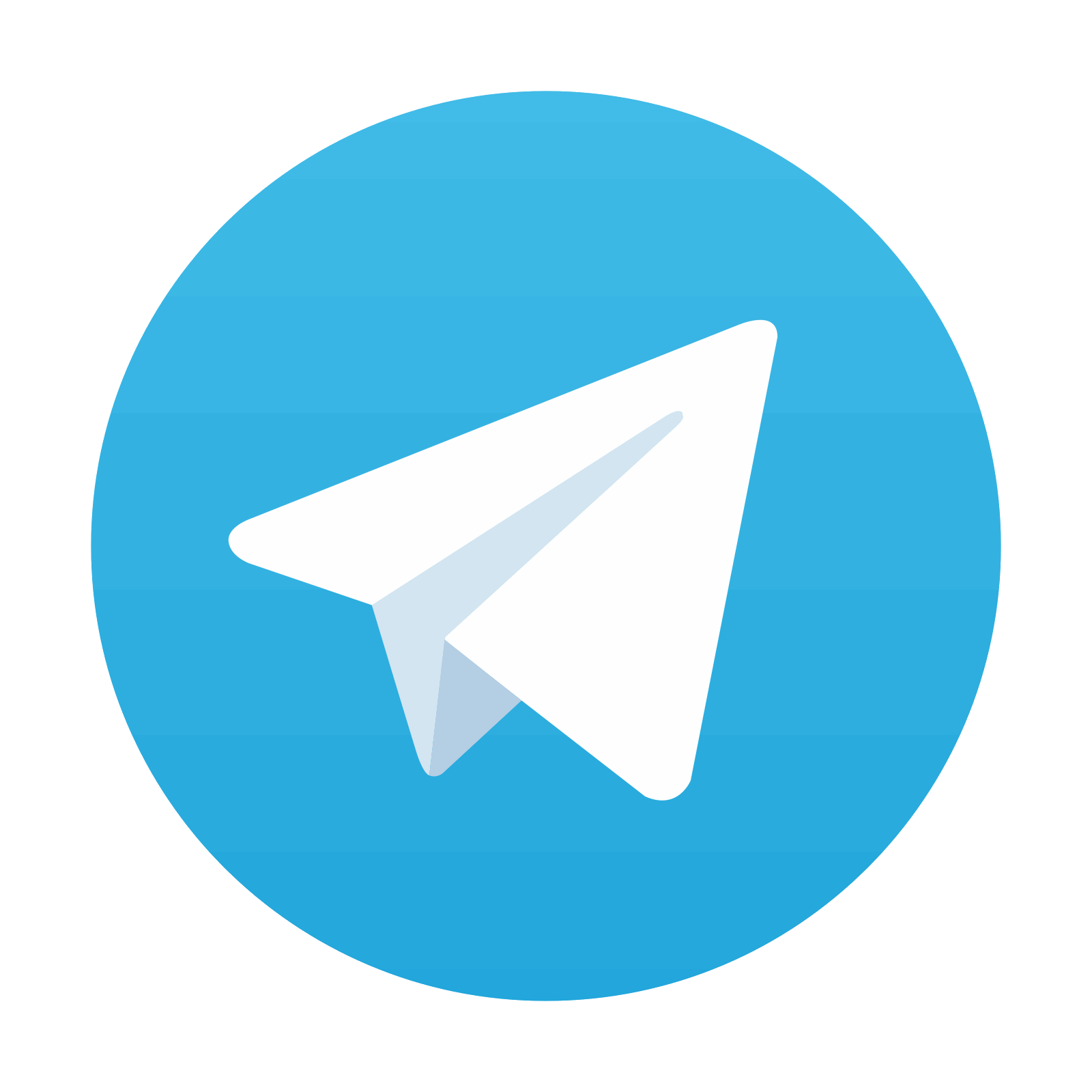
Stay updated, free articles. Join our Telegram channel

Full access? Get Clinical Tree
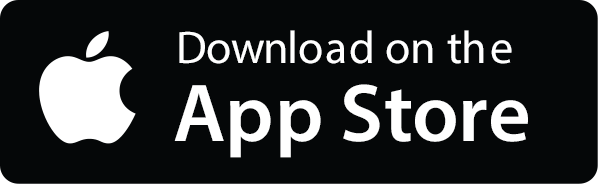
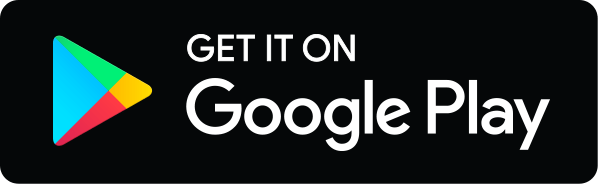