An interesting case report published in 2002 perhaps best sets the stage for understanding what anesthesiologists face when caring for a patient who suffers from severe blood loss while under an anesthetic.1 In this case report, a 70-year-old woman is anesthetized using a total intravenous technique with propofol and alfentanil for an elective aortic abdominal aneurysm repair. Routine monitors and a Bispectral Index Scale (BIS) monitor were used. The patient was enrolled as a study subject exploring the antioxidant effects of propofol. The procedure and anesthetic were unremarkable until after the cross-clamp was removed; then, the BIS values dropped first (mid-30s to below 20) followed 7 minutes later by a blood pressure drop (systolic pressure fell from about 120 to about 60 mm Hg) (Figure 23–1). Results from the measured plasma propofol concentrations were also very compelling. At the time of cross-clamping, target and measured propofol concentrations were 5.0 and 4.7 mcg/mL, respectively. After the cross-clamp was removed, they were 3.0 and 7.2 mcg/mL, respectively. Thus, even though clinicians sought to decrease propofol dosing, plasma concentrations were more than double the desired level.
Figure 23–1
Changes in bispectral index and mean arterial blood pressure after declamping (arrow.).1
Reproduced with permission from Honan DM, Breen PJ, Boylan JF, McDonald NJ, Egan TD. Decrease in bispectral index preceding intraoperative hemodynamic crisis: evidence of acute alteration of propofol pharmacokinetics. Anesthesiology 2002;Nov;97(5):1303-5.

Dr. Halford, a surgeon, submitted a letter to the editor of Anesthesiology after caring for several trauma victims after the attack on Pearl Harbor in 1941. He noticed poor outcomes in patients anesthetized with intravenous anesthetic sodium pentothal. He commented: “Then let it be said that intravenous anesthesia is also an ideal form of euthanasia…With this heterogeneous mass of emergency anesthetists, it is necessary to choose an anesthetic involving the widest margin of safety for the patient … Stick with ether.”2
Anesthesiologists recognize that (1) a full dose of certain anesthetics can lead to pronounced and often unwanted side effects with potentially disastrous consequences, (2) the need to be selective in choice of anesthetic, (3) and the need to incrementally dose their anesthetics for patients who have significant blood loss before or during surgery. The scientific basis for this practice, however, has not been well established. The main reason for this gap in anesthetic pharmacology research is that it is difficult and unethical to study how significant blood loss influences anesthetic in humans who suffer from hemorrhagic shock. As such, much of what drives clinical practice is based on research in animal models of hemorrhagic shock and limited observations in clinical practice, largely from case reports.
This chapter will review what is known about how blood loss and resuscitation influence the pharmacologic behavior of commonly used anesthetics (opioids, sedative–hypnotics, and inhalation agents) and the rational selection and dosing of these anesthetics when used for induction and/or maintenance of anesthesia. It will then formulate a set of key points targeted at improving patient safety.
Researchers have investigated how blood loss and hemorrhagic shock influence the behavior of numerous anesthetics that include sedative hypnotics,3,4,5,6,7,8 and 9 benzodiazepines,8,10 opioids,11,12,13 and 14 and local anesthetics.15 The most consistent finding is that equivalent dosing leads to higher drug concentrations in severe blood loss when compared with normal hemodynamic conditions. Decreased blood volume and cardiac output16,17 along with compensatory changes in vascular tone may explain these pharmacokinetic changes.
As an example, consider prior work exploring how moderate hemorrhage influences the pharmacokinetic profile of propofol.5 This study included a series of pilot studies in swine to identify:
The extent of blood loss (mL/kg) required to reach and maintain a target mean arterial blood pressure (MAP).
The propofol infusion rate (mcg/kg/min) that would achieve near-maximal drug effect (ie, BIS near 0) but allow animal subjects to survive the study period.
Based on prior work with remifentanil,13 an isobaric hemorrhage protocol (ie, blood removal to maintain a target pressure) removed a large volume of blood (48 mL/kg) over approximately 1 hour to maintain a MAP of 40 mm Hg. This was followed by a high remifentanil infusion of 10 mcg/kg/min for 10 minutes. This infusion rate is approximately 50- to 100-fold more than is used in clinical practice (0.1 to 0.2 mcg/kg/min). It is interesting to point out that when using this hemorrhage protocol and the high-dose remifentanil infusion, all animals survived.
When switching to propofol, in euvolemic control animals, a propofol infusion of 750 mcg/kg/min for 10 minutes was required to reach maximal effect (ie, BIS near 0). Using the same hemorrhage protocol used with remifentanil, a propofol infusion rate of 750 mcg/kg/min for 10 minutes was lethal. So were infusion rates of 500, 250, and 125 mcg/kg/min. Similar to what Dr. Halford experienced with sodium pentothal, it was clear that propofol’s cardiovascular depressant properties were harmful in severe blood loss.
Unlike remifentanil, propofol infusion rates required to achieve maximal effect under euvolemic conditions were not tolerated after a 50% loss in blood volume. Key points from these pilot studies were:
Selected sedative–hypnotics known to have cardiovascular depressant effects to include propofol and sodium pentothal are not well tolerated during or following moderate to severe blood loss.
Even conventional doses can lead to cardiovascular collapse and death. If these drugs are to be used, doses should be markedly reduced to achieve desired clinical endpoints in sedation and hypnosis.
By contrast, opioids, ketamine, and etomidate are better tolerated during or following moderate to severe hemorrhagic shock.
By conducting additional pilot studies, it was discovered that with moderate blood loss (30 mL/kg), animals would tolerate a low propofol infusion rate (200 mcg/kg/min). Although not nearly as severe as the blood loss tolerated with the remifentanil infusion, animals did exhibit hemodynamic signs and a metabolic profile consistent with hemorrhage shock (ie, tachycardia, low central venous pressure, low cardiac index [decreasing from 5.0 to 2.6 L/min/m2], and lactic acidemia). The most important finding was that plasma propofol levels during and 3 hours following a brief 10-minute infusion were approximately 2-fold higher in the shock group (Figure 23–2). A pharmacokinetic analysis fitting a 3-compartment model to the propofol plasma concentrations over time for euvolemic controls and the shock group revealed that compartment volumes were smaller and compartmental clearances were decreased in the shock group (Table 23–1).
Figure 23–2
Presentation of measured plasma propofol concentrations and Bispectral Index Scale (BIS) levels in a swine hemorrhage model.5 The top plots present the mean propofol plasma concentration versus time following an infusion of propofol. The left top plot presents the plasma concentrations from a 10-minute 200-mcg/kg/min infusion to bled (30 mL/kg) and euvolemic swine. The right top plot presents the plasma concentrations from a 10-minute 200-mcg/kg/min infusion to bled swine and a 500- mcg/kg/min infusion to euvolemic swine. The y-axis is on a log scale. The bottom plots represent the mean BIS levels in each group. Key points are:
At an equivalent infusion rate (200 mcg/kg/min), propofol produces an effect (change in BIS) in bled animals but not euvolemic normotensive animals.
When the infusion rate is increased 2.5-fold for euvolemic normotensive animals, the effect is near equivalent to what is observed in bled animals.
(Reproduced with permission from Johnson KB, Egan TD, Kern SE et al: The Influence of Hemorrhagic Shock on Propofol: A Pharmacokinetic and Pharmacodynamic Analysis, Anesthesiology 2003 Aug;99(2):409-420.)

Parameter | Control Group | Shock Group |
---|---|---|
Volumes (L) | ||
Central compartment (V1) | 4.7 | 3.5 |
Rapidly equilibrating peripheral compartment (V2) | 16.7 | 7.4 |
Slowly equilibrating peripheral compartment (V3) | 232 | 165 |
Clearance (L/min) | ||
Elimination clearance (Cl1) | 1.6 | 0.8 |
Fast distribution clearance (Cl2) | 4.6 | 1.0 |
Slow distribution clearance (Cl3) | 1.9 | 0.9 |
Although compartment volumes and clearances do not represent true organ drug distribution and clearance, the changes do suggest that blood flow to peripheral tissues is markedly decreased such that intravenous anesthetics are essentially pumped straight to the brain in higher concentrations, leading to a more pronounced anesthetic effect.18
In this analysis, BIS was used as a surrogate measure of drug effect. In hypovolemic group, the propofol infusion produced a large decrease in the BIS that returned to baseline within 30 minutes of the infusion but had minimal effect in the euvolemic control group. To achieve a similar change in the BIS in the control group, the infusion rate had to be increased to 500 mcg/kg/min (see Figure 23–2).
Kurita et al recently studied the simultaneous infusion of propofol (100 mcg/kg/min) and remifentanil (0.5 mcg/kg/min) during compensated and uncompensated hemorrhagic shock in a swine model.14 Plasma propofol and remifentanil concentrations increased during both compensated and uncompensated hemorrhagic shock, but the increase was more pronounced during uncompensated hemorrhagic shock. Uncompensated hemorrhagic shock was defined as the transition from increasing to decreasing systemic vascular resistance. In this study, 10% of estimated blood volume was removed every 30 minutes for 90 minutes followed by removal of 5% of estimated blood volume every 20 minutes until circulatory collapse. The onset of uncompensated hemorrhagic shock occurred within 3 to 4 hours at a point where 35% to 40% of the blood volume had been removed.
During the compensatory phase of this hemorrhage protocol, the rise in remifentanil and propofol concentrations as a percentage change from baseline concentrations prior to hemorrhage were different. Remifentanil plasma concentrations rose as a function of blood loss 3 times faster than propofol. The study results indicated that 30 minutes into the hemorrhage protocol, remifentanil concentrations increased by 95% over baseline. Propofol required 50 minutes to achieve the same percentage change over baseline. Once in the decompensatory phase, both remifentanil and propofol plasma concentrations rose at an even faster rate, with remifentanil out-pacing propofol until cardiovascular collapse.
The authors put forth several proposed mechanisms to describe their observations. They suggest that propofol concentrations were relatively insensitive to changes in cardiac output during the compensatory phase of hemorrhagic shock but became very sensitive during the uncompensated phase associated with a large decrease in cardiac output. Remifentanil was somewhat less sensitive to cardiac output changes. This may be because of remifentanil’s metabolism by nonspecific esterases in peripheral tissues. This explains the large increase in remifentanil plasma concentrations once perfusion to peripheral tissues was decreased.14
Pharmacodynamics describes the concentration-effect relationship and parameters that describe a sigmoid curve (ie, effect-site concentration versus effect) to include Emax (a measure of the maximal effect), C50 (the effect-site concentration that produces 50% of the maximal effect), and γ (a measure of curve steepness) are used to characterize drug potency.
Prior work exploring changes in propofol pharmacodynamic parameters between euvolemic and hemorrhagic hypovolemic conditions in swine and rats has revealed that C50 was shifted to the left.3,5 For example, in swine the C50 is 2.7-fold less in the shock group (4.6 versus 1.7 mcg/mL for the control and shock groups, respectively; Figure 23-3). The mechanism of how blood loss increases propofol potency is not clear but may be the result of a rise in circulating endorphins. The effects of propofol are known to synergistically interact with opioids.19,20 Blood loss does lead to a rise in circulating β endorphins.21,22 and 23 Hence, high levels of beta endorphins may interact synergistically with propofol to increase its potency. However, De Paepe et al3 found that endorphin antagonism with naloxone did not influence end-organ sensitivity to propofol in a rodent hemorrhage shock model.
Figure 23–3
The concentration-effect relationship as characterized by the pharmacodynamic model. The black line represents the mean change in the Bispectral Index Scale (BIS) over a range of propofol effect-site concentration (Ce) levels for unbled controls. The orange lines represent the mean change in the BIS over a range of propofol Ce levels for bled animals. The black arrows illustrate the shift in the C50 between groups.5

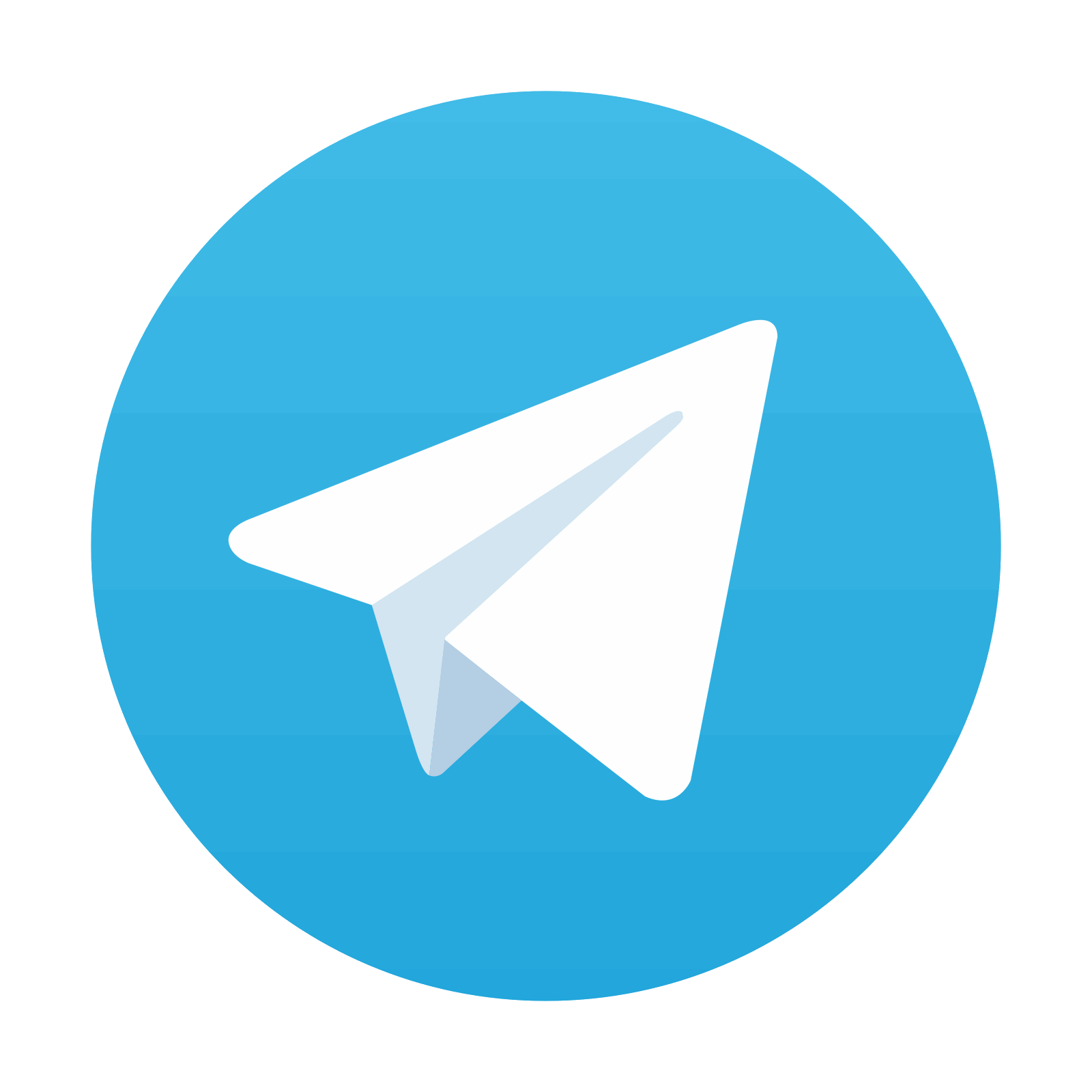
Stay updated, free articles. Join our Telegram channel

Full access? Get Clinical Tree
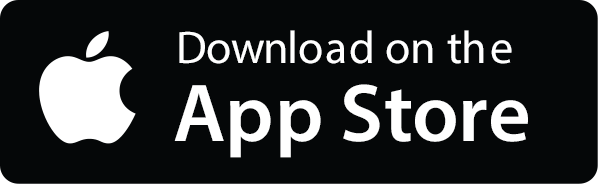
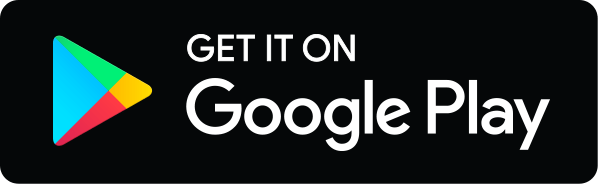
