Immunosuppression in Solid-Organ Transplantation
Amit Basu
Arthur J. Matas
Abhinav Humar
Clinically successful solid-organ transplantation required breakthroughs in our understanding of immunology and immunosuppressive therapy. Alexis Carrel, in the early 1900s, described what was to become the modern method of vascular suturing; experimental transplants soon followed [1], but the first successful clinical transplant was not done until five decades later. During that interval, it gradually became apparent that early rapid destruction of allografts was due to an immune process, which came to be known as rejection.
Organ transplantation has now become commonplace as the results have improved remarkably with the use of more potent and specific immunosuppressive agents. Progress in nonrenal transplantation has especially accelerated with the use of newer and more potent immunosuppressive agents. Besides the developments in techniques and immunosuppression protocols, progress in tissue typing and cross matching, and in preservation and transportation of harvested organs have played major roles in the rapid development of organ transplantation.
This chapter reviews the clinical use and the adverse reactions associated with commonly used immunosuppressive agents.
Pharmacologic Agents
Calcineurin Inhibitors
Cyclosporine (CSA) and tacrolimus (TAC), although structurally dissimilar, have a similar mechanism of action. Both drugs interfere with the cellular pathway for cytokine production and proliferation. Early events in the T-cell activation process are associated with a rise in the levels of intracellular calcium. The protein calcineurin has been validated as part of the calcium-dependent signal transduction pathway of interleukin-2 (IL-2) production in T cells [2]. CSA and TAC bind to two intracellular receptors, CypA and FKBP12, respectively; these receptors are found in virtually all cell types. The resulting receptor complex binds to calcineurin, blocking its phosphatase ability and thereby stopping the production of IL-2 [2].
The two calcineurin inhibitors (CNIs) currently used are described separately in the following sections.
Cyclosporine
CSA was isolated from a soil sample in Norway and produced by the fungus Tolypocladium inflatum. The first formulation of CSA that was approved by the U.S. Food and Drug Administration (FDA) was Sandimmune®; this was modified in the early 1990s by the microemulsion (ME) formulation called Neoral®. In 2000, the first generic versions of CSA were launched. CSA has remained a major component of many transplant regimens.
Pharmacokinetics
CSA is a lipophilic decapeptide, consisting of several amino acids in a ring structure. The original oral formulation (Sandimmune®) is in an olive-oil vehicle, which is necessary to promote absorption [3]. Absorption of Sandimmune® is erratic and it requires the presence of bile in the upper small intestine for absorption. Because many liver transplant recipients require diversion of bile to external drainage, absorption of Sandimmune® is problematic for them [4]. Absorption is also complicated by the presence of food and the length of drug therapy. Neoral® self-emulsifies in water, making absorption much more reliable and much less dependent on the presence of bile.
Studies comparing the two formulations showed these advantages with the ME: a more consistent and linear elimination of CSA; higher area-under-the-curve (AUC) values, leading to reduced dose requirements; reduced effects of diet, and, especially for liver recipients, much better absorption [4]. The side effect profiles were unchanged. The ME has become the primary formulation for CSA. CSA is generally considered a narrow-therapeutic-range drug, so whether generic versions can be used without additional pharmacokinetic study has been controversial.
The oral bioavailability of the ME formulations is approximately 30%. The average half-life of CSA ranges from 6 to 9 hours, with a tmax (ME) of approximately 1 hour. CSA is highly bound in plasma to red blood cells. It is extensively metabolized by the liver to multiple metabolites via the cytochrome P450 3A4 enzyme system; however, most of the metabolites are considered essentially inactive. Significant liver impairment can slow the clearance of CSA by the body. Because very little drug is eliminated by the kidney, renal failure does not change CSA elimination [3].
CSA is available as an oral soft gelatin capsule (Neoral®, Sandimmune®), as an oral solution (Sandimmune®, Neoral®), and as an intravenous (IV) preparation (Sandimmune®). To convert to IV use, the IV dose must be calculated as one-third of the daily oral dose. The IV dose can be administered over 6 hours; however, a continuous infusion is usually desired to minimize toxicity.
Adverse Events
The extensive side effect profile of CSA has long been a reason for attempts at minimizing drug exposure. Of most concern is its acute and chronic nephrotoxicity. Acute nephrotoxicity from CSA initially is characterized by vasoconstriction of the intrarenal arterioles, resulting in a reduced glomerular filtration rate. This mechanism of vasoconstriction is not well understood, but may be a result of increase in the vasoactive substance endothelin I [5], the activation of the renin-angiotensin system resulting in increased levels of angiotensin II [6], and possibly a decrease in production of nitric oxide [7]. CSA may also affect prostacyclin levels and induce vasoconstriction by increasing thromboxane A2 [3].
CSA-induced thrombotic microangiopathy (TMA) was first reported in liver allograft recipients, then in kidney and heart recipients [8]. TMA can present as a full-blown syndrome consisting of hemolytic anemia, thrombocytopenia, neurologic abnormalities, fever, and renal failure. Pathogenic mechanisms include a direct cytotoxic effect on endothelial cells, reduction in prostacyclin synthesis leading to vasoconstriction, platelet aggregation, and thrombus formation. CSA reduces the generation of activated protein C from endothelial cells and increases thromboplastin production from mononuclear and endothelial cells, thus contributing to a prothrombotic effect. Discontinuation of CSA is an important step in management along with plasmapheresis and fresh frozen plasma replacement. TAC or sirolimus (SRL) can be substituted as immunosuppressive agents, although TMA can occur with both these agents.
In heart and lung transplant recipients, the effect of CSA on long-term kidney function has been significant. Kidney biopsies of their native kidneys reveal wrinkling and thickening of the glomerular basement membrane, with some kidneys exhibiting microthrombotic angiopathy and fibrosis. Clinical findings showed that several of these recipients had advanced to end-stage renal disease requiring dialysis; others developed significant proteinuria [9]. However, a study of kidney recipients showed that the incidence of rejection correlated with poorer long-term graft function; higher CSA levels were associated with better, not worse, graft function [10]. Whether or not higher CSA levels are to blame for chronic CSA nephrotoxicity is still a matter of discussion. Transforming growth factor-B type 1 (TGF-B type 1) and platelet-derived growth factor, both fibrogenic cytokines, are produced in increasing amounts by human renal proximal tubular cells by increasing concentrations of CSA [11]. An increase in the activation of the renin-angiotensin system has been linked with the morphological changes that occur in chronic CSA nephrotoxicity by experimental studies [12], and angiotensin II receptor blockers reduce these changes.
Hypertension is another significant adverse event with CSA. Most patients receiving CSA develop hypertension, sometimes requiring multiple drug therapy. The mechanism for CSA-induced hypertension is primarily related to small-vessel vasoconstriction. The renal vasoconstriction may be affected, in part, by increased endothelin production. Patients also develop sodium retention and lower plasma renin levels [13]. Treatment of hypertension has focused on calcium-channel blocker use, because calcium-channel activation induces endothelin vasoconstriction and increases blood pressure. Calcium-channel blockers, such as diltiazem, nifedipine, and amlodipine, have been shown to decrease renal vascular resistance and improve glomerular filtration rate. Given these beneficial renal effects, calcium-channel blockers have been used to try to reduce chronic nephrotoxicity associated with CSA. Clinical evidence of a salutary effect has been conflicting, and further study is needed.
Table 181.1 Significant Drug Interactions (Cyclosporine, Tacrolimus, Sirolimus) | |||||||||||||||||||||||||||||||||||||||
---|---|---|---|---|---|---|---|---|---|---|---|---|---|---|---|---|---|---|---|---|---|---|---|---|---|---|---|---|---|---|---|---|---|---|---|---|---|---|---|
|
CSA has been associated with several neurologic toxicities, including headaches, tremors, seizures, and encephalopathy. In most instances, but not always, these effects are seen with higher CSA levels. A decrease in dosage may prevent serious tremors and headaches. Reversible posterior leukoencephalopathy can occur after CSA use and affects the posterior white matter and the frontal lobes and gray matter as well [14]. It manifests with confusion, coma, cortical blindness, cerebellar syndrome, hemiplegia, and flaccid paralysis or various combinations of these features. This neurological syndrome and brain imaging abnormalities usually resolve within 2 weeks of stopping CSA, or after dosage reduction if blood levels were high [15]. Hypertrichosis and gingival hyperplasia can reduce patient compliance to CSA. Many patients develop hair growth on their backs and arms; although not life threatening, these cosmetic changes can have emotional and physical repercussions, potentially resulting in graft loss if noncompliance ensues. Electrolyte imbalances may occur with CSA, including hyperkalemia, hyperuricemia, and hypomagnesemia. Patients usually need diet instruction and sometimes electrolyte replacement to control these changes. CSA can increase cholesterol and triglyceride levels, sometimes requiring treatment with lipid-lowering medications [3].
Drug Interactions
CSA is metabolized by the cytochrome P450 3A4 enzyme system that is found not only in the liver but also in the cells lining the intestine; so CSA levels can be increased or decreased by changes in gut absorption or in liver metabolism [16]. Some centers try to manipulate the interaction, intentionally using compounds that inhibit CSA metabolism to decrease the dosage required and, thus, the cost [17]. This practice is controversial, because any change in the interacting drug used affects CSA levels. CSA interactions may also occur with medications that change gut motility and with other nephrotoxic agents [16]. Table 181.1 lists the drugs that affect CSA metabolism, efficacy, and nephrotoxicity.
Clinical Use
CSA was and continues to be extensively used in organ transplantation, especially renal transplant, although now a different CNI, tacrolimus, has become the more commonly used primary immunosuppressive agent [18]. When CSA is used, it is often the ME formulation (Neoral®),
although patients with stable allograft function from earlier years may still be using Sandimmune. As Sandimmune® and ME formulations are not considered bioequivalent by the FDA, one cannot be substituted for the other without careful monitoring of doses and serum concentrations. Most centers initiate CSA therapy at 4 to 8 mg per kg per day orally, starting the day after transplant. If the transplanted kidney shows signs of acute tubular necrosis posttransplant, some centers may delay the initiation of CSA. Anti–T-cell preparations may be used during this time to provide T-cell suppression if CSA cannot be started [3]. Because of the better bioavailability of the ME formulation, the need for IV CSA has decreased but still may be necessary if the patient has significant diarrhea or cannot tolerate any oral or nasogastric medications.
although patients with stable allograft function from earlier years may still be using Sandimmune. As Sandimmune® and ME formulations are not considered bioequivalent by the FDA, one cannot be substituted for the other without careful monitoring of doses and serum concentrations. Most centers initiate CSA therapy at 4 to 8 mg per kg per day orally, starting the day after transplant. If the transplanted kidney shows signs of acute tubular necrosis posttransplant, some centers may delay the initiation of CSA. Anti–T-cell preparations may be used during this time to provide T-cell suppression if CSA cannot be started [3]. Because of the better bioavailability of the ME formulation, the need for IV CSA has decreased but still may be necessary if the patient has significant diarrhea or cannot tolerate any oral or nasogastric medications.
Therapeutic Drug Monitoring
Monitoring CSA levels is vital. Maintaining the appropriate levels in the first 6 months posttransplant has a significant effect on graft survival [19]. Monitoring CSA is a challenge because of the differences in bioavailability between patients, the narrow therapeutic range, and the number of compounds available that affect CSA blood concentrations. Several different assays are currently in use to measure CSA. The various methods used today measure whole-blood CSA levels and include radioimmunoassay (RIA), high-performance liquid chromatography (HPLC), and monoclonal antibody assays. HPLC only measures the parent compound of CSA, whereas radioimmunoassay and the monoclonal assays measure CSA plus several metabolites. When deciding whether a blood concentration is appropriate, it is important to know which assay the laboratory is using.
Traditionally, trough concentrations (C0) of CSA have been used to determine the appropriateness of a dosing regimen. Earlier studies were performed with the Sandimmune® formulation, which had quite variable dose-response curves. After the use of the ME preparation became standard, several studies suggested that measuring the AUC would be more predictive of toxicity and rejection (compared with the C0) [20]. AUC monitoring requires more blood samples per measurement, and it is therefore more costly and impractical in clinical practice [20]. A monitoring strategy measuring AUC for the first 4 hours after dosing (AUC 0 to 4 hours) correlates well with clinical outcomes, although it still requires multiple blood samples [21]. A blood sample taken 2 hours after intake of Neoral® (C2) is the most accurate one-point predictor for AUC 0 to 4 hours and shows less variability than either C0 or C1. In retrospective analysis, the risk of acute rejection is reduced in patients in whom C2 were greater than 1,500 μg per L in the 2 weeks following transplantation [22].
In a prospective study, 45% of C2-monitored patients failed to reach the target levels by day 5 posttransplantation compared with 2.5% of Co-monitored patients [23]; this may explain why the theoretical benefit of C2 monitoring in the early posttransplant period is not borne out. Due to the lack of prospective evidence showing an advantage for C2 monitoring in the early posttransplant period, trough levels (C0) remain the standard.
Tacrolimus
With the success of CSA, researchers studied soil samples from around the world, looking for another compound that might turn out to display immunosuppressive properties. TAC, initially known as FK-506, was isolated from a soil sample in Tsukuba, Japan, in May 1984, from the fungus Streptomyces tsukubaensis [24]. It has a completely different chemical structure from CSA, yet its effect on the lymphocyte is remarkably similar. A few differences have been found on the cellular level between CSA and TAC. The FKBP12-TAC complex is 10 to 100 times as potent as CSA, possibly due to greater affinity for its binding protein [24,25].
Pharmacokinetics
The pharmacokinetics of TAC are similar to CSA. TAC has an extremely lipophilic, macrocytic lactone structure. Its oral bioavailability ranges anywhere from 4% to 93% (average 25%), with variable dose-response curves between patients. Because of this poor oral bioavailability, the IV dose should be calculated at approximately one-third of the oral daily dose [26]. One significant difference between CSA and TAC is that with TAC the presence or absence of bile in the digestive tract does not significantly alter absorption. This was a problem with Sandimmune® and a reason that TAC was initially studied in the liver transplant population. TAC binds extensively to erythrocytes and exhibits the same temperature-dependent properties as CSA. The metabolism is also similar to CSA, with the cytochrome P450 3A4 system as the primary metabolic pathway. The many metabolites for TAC are still being studied. Less than 1% of active drug is excreted through the urine. The average elimination half-life ranges from 8 to 20 hours, depending on the population studied [26].
TAC is available as a 0.5-mg, 1.0-mg, and 5.0-mg capsule, formulated as a solid dispersion in hydroxymethylcellulose. A suspension can be compounded if necessary for pediatric or nasogastric administration. An IV preparation is solubilized in alcohol and a surfactant. It is available as a 5 mg per mL concentration that must be diluted and administered as a continuous infusion to avoid toxicity [26].
Adverse Events
The adverse event profile of TAC is similar to CSA in many respects. TAC appears to have the same nephrotoxicity seen with CSA, and the mechanism also appears to be the same. However, in one study, mean or median serum creatinine levels in renal transplant recipients were lower in TAC-treated patients, with 5 years follow-up, than in patients treated with cyclosporine ME (or standard formulation) [27]. As with CSA, the nephrotoxicity of TAC is concentration dependent, making drug level monitoring equally important [28].
Hypertension has also been reported with TAC. However, the 5-year follow-up results from the U.S. randomized trial indicate that significantly fewer TAC than CSA recipients were receiving antihypertensive treatment (80.9% vs. 93%, p < 0.05) [27]. Immunosuppression with TAC-based regimens is associated with better lipid profiles than is immunosuppression with CSA-based regimens [29]. Neurotoxicity appears to be somewhat worse than with CSA. In randomized trials, liver recipients had more trouble with the neurotoxicity of TAC versus CSA, even when controlling for previous liver failure–induced encephalopathy [30]. Headache, tremor, neuropathy, seizures, blindness, coma, and various other neurologic complaints have been seen with TAC [30]. Patients usually recover when the drug is stopped. The incidence of hyperkalemia appears to be similar to that with CSA, although hypomagnesemia is more likely to occur with TAC-treated patients [31].
TAC-associated TMA has a reported incidence between 1% and 4.7% [32]. All patients have an elevated serum creatinine, but do not always show signs of hemolysis. Renal allograft biopsy provides a conclusive diagnosis. Treatment consists of reduction or discontinuation of TAC, anticoagulation, and/or plasmapheresis with fresh frozen plasma exchange and leads to resolution of TMA in most instances. Rarely, there may be loss of kidney function or patient death.
The incidence of posttransplant diabetes mellitus (PTDM) was significantly higher among TAC-treated patients than CSA-treated patients (9.8% vs. 2.7%) according to a meta-analysis [33]. Many patients with PTDM have reversal of diabetes mellitus, with eventual discontinuation of insulin. In a U.S. trial combining TAC with mycophenolate mofetil (MMF) and corticosteroids, the 10-year incidence was 6.5%, and the 1-year prevalence was 2.2% [34]. TAC does not appear to cause hypertrichosis or gingival hyperplasia, but instead is associated
with hair loss. Sometimes these differences become important enough to cause a change in therapy.
with hair loss. Sometimes these differences become important enough to cause a change in therapy.
Drug Interactions
TAC is metabolized through the same pathway as CSA and has been subject to the same interactions with the cytochrome P450 3A4 system. If the medication is known to alter P450 3A4 activity, it probably alters TAC concentrations. Drugs that cause nephrotoxicity also have the same additive effects with TAC as with CSA (Table 181.1).
Therapeutic Drug Monitoring
As with CSA, careful blood concentration monitoring is required; TAC also has a narrow therapeutic range. TAC is extensively bound to erythrocytes, so whole-blood trough measurements have become the standard for drug monitoring. The primary assay used currently is an automated microparticle enzyme immunoassay, available from Abbott Laboratories (Abbott Park, IL). Several generations of this assay have been used, with the current assay more sensitive at lower drug concentrations. The current suggested therapeutic range for TAC is 5 to 20 ng per mL; however, this range is still controversial and under study [25].
Clinical Use
Because TAC does not require bile to be absorbed, its use has attracted a great deal of interest in liver transplantation. Sandimmune® required bile in the small intestine, and if the bile drainage was being diverted it was almost impossible to obtain adequate CSA blood levels. TAC provided a possible advantage in liver transplantation, so the first major trials were in liver recipients.
The U.S. Multicenter FK-506 Liver Study Group compared the efficacy and safety of a CSA-based regimen (using Sandimmune®) versus a TAC-based regimen in adult and pediatric liver recipients at 12 different centers in the United States [30]. Recipients were randomized to CSA in combination with Azathioprine® (AZA) and steroids, or to TAC in combination with steroids. The investigators looked at patient and graft survival rates as well as the incidence of acute rejection, steroid-resistant rejection, and refractory rejection. At 1 year posttransplant, patient and graft survival rates were similar between the two groups, but TAC was associated with fewer episodes of all categories of rejection. The TAC group did have an increased incidence of adverse events, including nephrotoxicity, neurotoxicity, and hyperglycemia. Follow-up studies using lower doses of TAC have shown a reduction in these adverse events [35,36].
TAC is usually initiated at a dose of 0.05 to 0.10 mg per kg per day. Some centers use a standard starting dose of 2 mg BID, and adjust doses based on the blood concentration. As with CSA, TAC may be delayed after a kidney transplant in the case of graft dysfunction, and started when the kidney is recovering from acute tubular necrosis.
In studies of TAC and CSA in kidney recipients, results have been similar to those with liver recipients (i.e., same graft and patient survival rates, fewer rejection episodes) [37]. This pattern has also been seen in higher-risk patient populations, such as black recipients [38]. Other transplant categories with historically higher rates of rejection, such as pancreas transplant recipients, have seen benefit with TAC-based immunosuppressive regimens [31]. TAC continues to be the primary maintenance immunosuppressive agent in heart, lung, and bowel recipients, and was approved by the FDA for heart transplantation in 2006 [39,40,41,42].
Antiproliferative Agents
Antiproliferative agents have been part of transplant protocols since the first transplant was performed in the 1960s. Early antiproliferative agents included radiation, azaserine, and actinomycin D. AZA, developed in the early 1960s, was part of the first successful transplant series reported in 1963. It continues to be used today in maintenance immunosuppressive regimens and for autoimmune diseases. Cyclophosphamide was used when AZA use was not possible, but because of side effects it has never been considered a suitable alternative. A major advance in antiproliferative agents has been the development and use of MMF, released for clinical use in 1995. MMF is now a component of most new transplant regimens, with AZA having been used in transplants performed before 1995.
Azathioprine
Pharmacology
AZA is actually a prodrug of 6-mercaptopurine, an antineoplastic agent used in leukemia regimens. It acts by the inhibition of purine synthesis in the de novo pathway. This purine inhibition leads to the inhibition of the mixed lymphocyte reaction, and to a lesser extent, the antigen–antibody reaction [43].
Pharmacokinetics
AZA is rapidly absorbed after oral administration, with peak levels occurring 1 hour after ingestion. The large first-pass effect after oral administration means that IV doses must be multiplied by a factor of two. AZA is metabolized by xanthine oxidase through several steps to 6-thiouric acid and excreted into the kidneys. Although the half-life of the parent drug is relatively short, the pharmacodynamic effects of the parent drug and metabolites far outlast the time that AZA is present in the bloodstream [43].
Adverse Events
AZA is relatively well tolerated by most patients. The most common side effect is myelosuppression due to suppression of purine synthesis by AZA. The myelosuppression is usually limited to the white blood cells, but occasionally red cell aplasia is observed. Most patients can tolerate this effect by reducing the daily dosage, although some need to discontinue the drug entirely. Liver function tests must be regularly monitored: AZA has been reported to cause hepatic necrosis and liver failure. Pancreatitis or a skin rash may indicate an allergic reaction, in which case AZA may need to be stopped. Hair loss is bothersome to some patients but is reversible. Gastrointestinal (GI) disturbances, including nausea and vomiting, are mild and usually tolerable [43].
Drug Interactions
Severe pancytopenia has been reported when AZA and allopurinol are used together. It is recommended that AZA doses be reduced by 75% if allopurinol is added to the patient’s drug regimen. With the development of MMF, the management of this interaction has become easier, as MMF (which is metabolized differently than AZA) can be substituted for AZA when allopurinol is indicated [43].
Clinical Use
AZA is available as a 50-mg tablet that can be split, if necessary. A compounded suspension of 5 mg per mL can be used if tablets are not an option. AZA is also available IV. Most recipients are maintained on a dose of 1.0 to 2.5 mg per kg per day. AZA has an important historical role in transplantation, but its use has declined as newer agents have been introduced. Most likely, recipients currently on AZA were transplanted before 1995 and have done well on that initial regimen. Some centers switched all their recipients when MMF became available, but many are still maintained on AZA due to a significant cost advantage over MMF.
Mycophenolate Mofetil
MMF was approved by the FDA in 1995 to prevent rejection in kidney recipients. Its use has grown to include liver, heart, lung, and pancreas recipients. It has been a major addition to
the immunosuppressive arsenal. Many centers have replaced AZA with MMF in their current protocols.
the immunosuppressive arsenal. Many centers have replaced AZA with MMF in their current protocols.
Pharmacology
MMF is also a prodrug, quickly metabolized to the active compound, mycophenolic acid (MPA). MPA acts as a noncompetitive inhibitor of inosine monophosphate dehydrogenase, thereby blocking de novo purine synthesis and proliferation in the T and B lymphocytes [44]. In vitro and in vivo data from rodent models of chronic allograft nephropathy suggest that MMF also decreases vascular smooth muscle cell proliferation, offering theoretical treatment possibilities for the morphology seen in chronic rejection [45].
Pharmacokinetics
Oral MMF is rapidly hydrolyzed in the bloodstream by esterases to MPA, with no measurable parent compound in serum [46]. The oral bioavailability for MPA approaches 100%, so the IV to oral conversion ratio is 1:1. IV administration of MMF provides measurable blood levels of the parent compound during infusion, with levels becoming immeasurable 12 minutes after the end of the infusion. Peak concentrations occur approximately 1 hour after IV or oral administration (but IV has a slightly higher peak than oral) [47]. MPA is subsequently glucuronidated in the liver to inactive metabolic mycophenolic acid glucuronide (MPAG). Enterohepatic cycling recirculates a significant percentage of MPAG secreted in bile back to MPA, displaying a secondary peak in plasma MPA concentration [48]. MPAG is eventually excreted, primarily in the urine; only 6% of MPAG is excreted in the feces [44].
Adverse Events
MMF can cause significant GI problems, including nausea, vomiting, diarrhea, abdominal pain, and gastroesophageal reflux. Persistent diarrhea not accompanied by fever may be associated with an erosive enterocolitis causing malabsorption of nutrients that has been attributed to a toxic action of the acyl MPAG metabolite on absorptive cells [49]. Occurrence of these side effects has more frequently been linked to the MMF dose rather than to the plasma concentration of parent compound or its metabolites. Dividing the total daily dose into four doses instead of two has been effective in reducing GI problems in some recipients. An alternative, enteric-coated form of MPA—mycophenolate sodium (EC-MPS)—has been developed to mitigate the GI toxicities. Patients who had GI intolerance on MMF administration required fewer dose changes of EC-MPS, and showed reduced symptom burden, better functioning, and improved health-related quality of life [50]. Neutropenia and thrombocytopenia can also occur with MMF, requiring a dosage reduction [44]. At 2 g per day, the occurrence rate in the major trials was comparable to AZA. Teratogenic trials of MMF in rabbits showed changes in offspring at doses equivalent to those given to humans. No human teratogenic trials have been performed (but the manufacturer recommends that female patients wait at least 6 weeks after stopping MMF before trying to conceive). Female healthcare workers are also advised by the manufacturer to not open capsules for fear of aerosolization of the drug. It is also recommended that IV MMF be administered using standard chemotherapy precautions [51].
Drug Interactions
MMF is not metabolized by the cytochrome P450 system; therefore, interactions with MMF only affect its absorption, enterohepatic cycling, or renal excretion. As discussed earlier, a significant percentage of the AUC for MMF comes from enterohepatic cycling. Cholestyramine, a bile acid resin, decreases cholesterol by interfering with its enterohepatic cycling. The mixture of cholestyramine and MMF decreases the total AUC by 40%, so the combination of these two drugs is not recommended [51]. Antacids appear to reduce absorption of MMF by 20%, so adjusting dosing times, if possible, is recommended. Ganciclovir and acyclovir compete with MPAG for secretion by the kidney, and animal studies have suggested a possible interaction [51].
Recipients treated with CSA in combination with MMF display lower MPA concentrations than do patients who are not receiving CSA [52]. However, coadministration of MMF with TAC tends to increase MPA levels due to the lack of CSA inhibitory effects, and also possibly due to the inhibition of the uridine diphosphate—glucuronosyl transferase that generates MPAG [53].
Clinical Use
The success of MMF has allowed it to generally replace AZA in many transplant centers. The results of three major trials were instrumental. The U.S., Tricontinental, and European trials compared MMF, in combination with CSA and steroids, with conventional immunosuppression. The U.S. and Tricontinental trials randomized patients to MMF at a low (2 g per day) or high dose (3 g per day) versus AZA, whereas the European study used a placebo instead of AZA [54,55,56]. All three trials saw significantly reduced rejection in the MMF arm at 6 months posttransplant. The low-dose and high-dose arms demonstrated significantly fewer rejection episodes and clinically significant reductions in the severity and treatment of rejection episodes. Whether long-term MMF changes survival rates is still controversial. The 3-year data from the U.S. trial periods do not yet show a statistically significant difference in patient or graft survival [53].
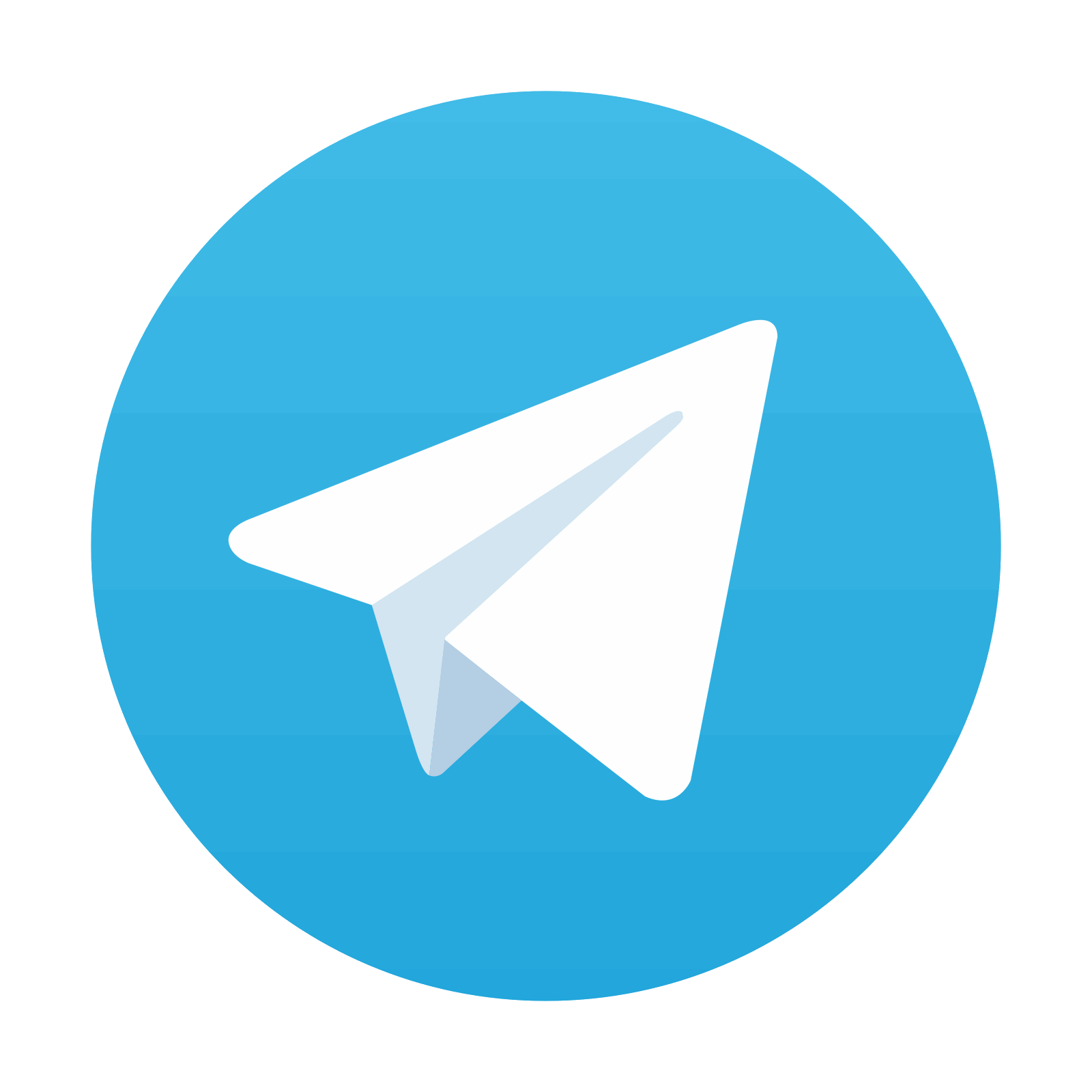
Stay updated, free articles. Join our Telegram channel

Full access? Get Clinical Tree
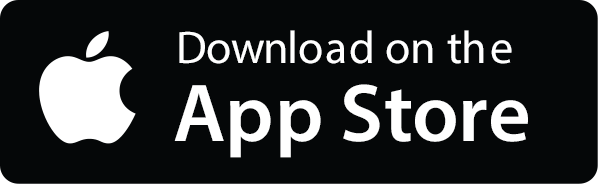
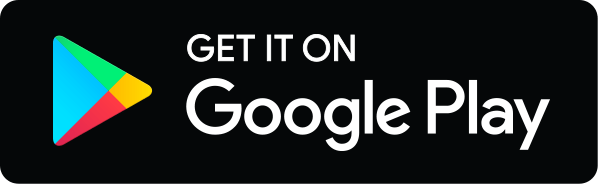
