Chapter 6 Immersion Into Cold Water
For online-only figures and videos, please go to www.expertconsult.com
The authors of this chapter hail from two different countries that view and measure temperature differently. The United States uses ° F (Fahrenheit), whereas the country up north uses ° C. (Some people think that the letter C stands for “Celsius,” but it actually means “Canadian.”) The author from up north has provided a conversion chart to allow neighbors from either side of the border to get on the same thermal wavelength (Box 6-1).
Immersion in cold water is a hazard for anyone who participates in recreational, commercial, or military activities in the oceans, lakes, and streams of all but the tropical regions of the world. Recreational aquatic activities include swimming, fishing, sailing, power boating, ocean kayaking, white-water rafting, canoeing, ocean surfing, windsurfing, waterskiing, diving, hunting, and using personal watercraft. In addition, winter activities like driving on icy roads and snowmobiling can involve cold-water exposure as a result of accidental entry into lakes and streams.1 Commercial activities that involve water include fishing, shipping, offshore oil drilling, and diving. Military operations over cold water include Coast Guard (Figure 6-1), Navy, and Marine Corps missions. Army, Air Force, and Marine Corps forces may encounter cold-water exposure during winter operations on land.
The definition of cold water is variable. The temperature of thermally neutral water, at which heat loss balances the heat production of a nude subject at rest (i.e., not shivering), is approximately 33° to 35° C (91.4° to 95° F).16,18 Hypothermia eventually results from immersion in water at less than this temperature. For practical purposes, significant risk for immersion hypothermia usually begins in water colder than 25° C (77° F),114,162,163 and it is quite significant in water colder than 15° C (59° F). Table 6-1 shows the variation in water temperature throughout the year at various sites in North America.180 Figure 6-2 shows typical worldwide sea-surface temperatures for April, with data from 2005.126 With the use of 25° C (77° F) as the defining point for cold water, the risk of immersion hypothermia in North America is nearly universal during most of the year.
TABLE 6-1 Mean Water Temperatures in ° C (° F)
Temperatures of <25° C (77° F) are noted in red.

FIGURE 6-2 Worldwide sea surface temperatures for April 2005.
(From the National Center for Environmental Prediction, Space Science and Engineering Center, University of Wisconsin–Madison. http://www.ssec.wisc.edu/data/sst/latest_sst.gif.)
Cold-water immersion is associated with two significant medical challenges: near drowning and hypothermia. This chapter discusses the physiologic responses to and treatment of immersion hypothermia, the risk of near drowning with respect to the physiologic consequences of sudden immersion in cold water, and the problems of survival in rough seas. Controversies are also identified and discussed. Chapter 5 discusses land-based hypothermia; Chapter 75 provides a more complete discussion of drowning; and Chapter 77 discusses diving injuries. Since the previous edition of this textbook was published, many audiovisual resources have been produced; several are listed in the Video Resources section at the end of this chapter.
History and Epidemiology
The history of human association with the sea and with inland waters provides abundant examples of the effects of accidental cold-water immersion. Case studies demonstrate the scope of the problem. Perhaps the most famous occurrence was the sinking of the Titanic in 1912. After striking an iceberg at approximately 11:40 PM on April 14, the ship sank in calm seas, but the water temperature was near 0° C (32° F). Of the 2201 people on board, only 712 were rescued, all from the ship’s lifeboats. The remaining 1489 people died in the water, despite the arrival of a rescue vessel within 2 hours. Nearly all of these people were wearing “life preservers,” yet their causes of death were officially listed as drowning.119 However, it is more likely that their deaths were caused by immersion hypothermia. Survivors reported hearing victims’ screams for periods long enough (i.e., more than 1 hour) to indicate that the individuals in the water survived for a period of time sufficient to result in severe hypothermia.102,110
Case 6-1 Longterm immersion in moderately cold water
On February 28, 2009, four friends who were all former or current football players in their mid-twenties set out on a fishing trip in a 21-foot boat into the Gulf of Mexico west of Tampa Bay, Florida. According to the survivor’s account,148 their boat capsized in a storm before they could return to shore, and the four men were thrown into the 18° C (65° F) water. Weather conditions were 4.3-m (14-foot) seas and 15 to 18 m/sec (30 to 35 knot) winds. None of the men were wearing PFDs at the time of the capsizing. One of the survivors dove under the boat to retrieve three keyhole PFDs and one seat cushion. Despite their large size (≈190 cm [74.8 inches] tall and ranging from 98 to 120 kg [216 to 265 lb] in weight) and high level of muscle mass and physical fitness, the men all developed hypothermia as a result of the combination of a low level of body-fat insulation, the cold water temperature, the effect of the wind on their wet clothing, and the strenuous work to remain on the boat or to get out of the water. Two victims died after about 13 hours, a third died after 25.5 hours, and the lone survivor was rescued after 43 hours by the U.S. Coast Guard. The first casualty was the lightest (≈98 kg [216 lb]) and wore only swimming trunks and a keyhole PFD; he had discarded his t-shirt, wind pants, and jacket to try to dive under the boat for supplies. The second casualty, who died at about the same time, was the heaviest (≈120 kg [265 lb]), but he had spent far more time immersed in the water than the others had. The third casualty survived another 12 hours, but he perished nearly 18 hours before the lone survivor was rescued. That casualty had also taken off his wind jacket and pants, which were later lost, so that he could dive under the boat. During the first 13 hours, he struggled to help the other victims while he was in the water, and he had little insulation from the seat cushion, which he wore on his back; he later switched to the keyhole PFD that had been worn by the first casualty. These early conditions likely put him behind the survival curve of his remaining companion. The survivor (≈109 kg [240 lb]) had the double advantage of less direct water exposure (he spent more time positioned on top of the boat, often holding onto the motor) and more insulation (i.e., sweatpants, sweatshirt, water-resistant and fleece-lined hooded coat, skullcap, and gloves). He had a core temperature (Tco) of 31.9° C (89.5° F) after 43 hours at sea (Figure 6-3, online). This case will be referenced throughout this chapter.
Case 6-2 panic in ice water
In February 1990, four teenage boys broke through the ice on Convict Lake in California. After one boy escaped and summoned help, a series of adults—including two lay and three professional rescuers—approached and also broke through the ice. It was not until a properly equipped rescue team arrived “that a safe rescue attempt could be made … on one lone adult. In just fifteen minutes, three boys and four of their five adult rescuers were dead.”48 Despite the common belief at the time, the victims did not perish as a result of hypothermia but rather from cold- and panic-induced incapacitation and drowning.
Case 6-3 “skating” on thin ice
In the winter of 1997-1998, four people died when their snowmobile broke through the thin ice of Lake Scugog, which is near Toronto, Ontario, Canada. The deaths occurred, ironically, during Snowmobile Safety Week in North America. The Canadian Safety Council reported that, in 1997, 101 people were killed in snowmobile mishaps, the majority of them by drowning and hypothermia after crashing through ice on frozen rivers and lakes.1
Case 6-4 problems with cold-water rescue
In the winter of 1988, two men died of hypothermia when their nine-man rowing scull sank during a winter storm on a small lake in western Canada. The nine rowers were immersed in 4° C (39.2° F) water. They struggled to hang on to their overturned vessel in 0.6 to 1.0 m (1.97- to 3.28-foot) waves and 30 km/hr (18.7 mph) winds for more than 50 minutes before rescuers could reach them. One man drowned just before rescue; the others were all conscious when they were pulled from the water. However, during the 13-minute boat transport to shore, three of the thinnest victims lost consciousness, and one of these men suffered cardiopulmonary arrest. His Tco in the hospital 44 minutes after rescue was 23.4° C (74.1° F); subsequent resuscitation efforts were unsuccessful. The remaining seven survivors were resuscitated from Tcos of as low as 25° C (77° F). This case illustrates the rapidity of onset of immersion hypothermia in lean subjects and the potentially serious consequences of cardiovascular instability, circum-rescue collapse, and post-rescue Tco afterdrop in individuals with immersion hypothermia (see Physiologic Responses to Cold-Water Immersion with the Head Above Water, later).58
Case 6-5 dangers of fishing in cold water
In December 2004, the fishing vessel Northern Edge capsized near Nantucket, Massachusetts, in 4° C (39.2° F) water and 3- to 4-m (9.84- to 13.12-foot) seas. Five of the six crewmembers lost their lives.184 In several similar cases in the same region, seven fishermen on three different vessels lost their lives during a 30-day period in the winter of 2004-2005.181 In June 2003, the charter fishing vessel Taki Tooo, which was carrying 17 passengers and crew, capsized in the turbulent waters of the Tillamook River bar on the Oregon coast, with the loss of 11 lives. The water temperature was 10° C (50° F).183 In April 2001, the fishing vessel Arctic Rose sank in the Bering Sea of Alaska, with a loss of 15 crewmembers.184 The vessel sank so quickly that there was no time for either a distress call to be made or for the crewmen to don survival suits.
Case 6-6 cold-water fishing fatalities
A 1999 U.S. Coast Guard Fishing Vessel Casualty Task Force Report181 found that, in just 2 months (December 1998 and January 1999), 20 commercial fishing vessels were lost at sea. During these mishaps, 21 persons on board died, mainly from exposure to cold water as a result of the vessel sinking or capsizing or from persons falling overboard. From 1994 to January 1999, U.S. Coast Guard statistics show that 396 fishermen lost their lives while fishing. Of these, 298 died from cold-water immersion as a result of falling overboard or of the vessel sinking, capsizing, or flooding. In 1998, the death rate for fishermen was 179 per 100,000 workers; this is 16 times higher than the rates for firefighters and police officers.
Commercial Activities on Cold Water
Numerous examples of maritime occupational accidents exist. In 1982, the offshore mobile oil-drilling platform Ocean Ranger collapsed in mountainous seas near Newfoundland, Canada; the water temperature was −1° C (30.2° F). Eighty-four workers were plunged into the water, and, despite the presence of a rescue vessel, all of them died. Immersion hypothermia was a significant factor in these deaths, although the inabilities of the workers to combat rough seas and to maintain airway freeboard (i.e., the distance between the water surface and the mouth) were also major problems.127
Fishing vessel mishaps are a common cause of immersion hypothermia. Cases 6-5 to 6-6 illustrate the problems that face the commercial fishing industry (Figure 6-4).
Military Activities and Cold Water
The extensive use of combat ships and aircraft in World War II, particularly in the north Pacific and north Atlantic oceans, provided many examples of accidental immersion in cold water. Molnar reviewed several hundred of these cases.124 Among them was the following: “A ship was rammed, and it sank in 3 minutes. Thirty survivors were picked up from rafts after exposure of 1.5 to 4 hours. Some drowned and others died of exposure because the water was 4° C (7.2° F) with high seas and a wind velocity of scale 7. Some of the survivors who held on to ropes couldn’t let go and rescuers had to cut frozen rope to release them. It appears miraculous how the survivors could have endured such cold water. Most of those who were rescued were in an unconscious state and, when they became conscious, complained of numbness of extremities and hands.”
Maritime military operations are not the only source of hypothermia casualties in the armed forces; land operations, either during combat or training, also have immersion hypothermia risks. In March 1995, four U.S. Army Rangers died and four others were hospitalized during a training mission in a Florida swamp. The water at the exercise site was deeper and colder than was customary for this exercise. Consequently, the Rangers were forced to spend more than 6 hours in cold and wet conditions, much of the time partially immersed in the 15° C (59° F) water. Night conditions and difficulties encountered during the medical evacuation of the accident victims contributed to the hypothermia deaths.17,186
In March 2001, two U.S. Coast Guard crewmen drowned after immersion in cold water, when their four-man vessel capsized in the waves of the Niagara River bar in Lake Ontario. The water temperature was 2° C (3.6° F). All of the crewmen were wearing dry suits with insulation as well as PFDs, but they were without insulated headgear. They left the boat to swim towards a buoy. Vigorous swimming caused water intrusion into two of the dry suits, as did the coxswain’s use of a neck ring device before capsizing. This leakage resulted in severe immersion hypothermia and subsequent drowning.182
Vehicular Activities on Ice
Motor vehicle submersions account for approximately 10% of all drownings, including approximately 400 fatalities in North America each year.177,201 Although most of these deaths occur in open water during spring, summer, or fall, many also occur during the winter, when vehicles break through the ice while traversing either makeshift (e.g., ice fishing) or official (e.g., winter roads) transport routes. In 2005, a heavy machine operator was maintaining an icy road when his snowplow broke through the ice, and he drowned.91 The ice-cold water temperature was cited as a contributing factor to his death.
Physiologic Responses to Cold-Water Immersion With the Head Above Water
The effect of sudden cold-water immersion on humans is poorly understood by the general public as well as by medical and rescue personnel. Many individuals believe that an individual who falls into ice water will die of hypothermia within minutes, even if that person is dressed in winter clothing. In a series of surveys of continuing medical education attendees,47 75% of respondents felt that, under these conditions, a victim would experience life-threatening hypothermia in less than 30 minutes. As discussed later in this chapter, most adults can survive for an hour or more if they take appropriate action. The improper assumption of rapid and impending death only serves to induce panic, poor decisions, and exhaustion as a result of thrashing about, thereby causing problems for both primary victims and for the persons trying to rescue them.
The primary pathophysiologic effects of hypothermia are a decrease in tissue metabolism and the gradual inhibition of neural transmission and control. However, during the initial stages of cooling of an intact and conscious victim, secondary responses to skin-temperature cooling predominate. Sudden immersion in cold water results in an immediate decline in skin temperature, which, in turn, initiates shivering thermogenesis with increases in metabolism, ventilation, heart rate, cardiac output, and mean arterial pressure. As body temperature declines and shivering ceases, metabolism, heart rate, mean arterial pressure, and cardiac output decrease proportionally with the fall in Tco, whereas hematocrit and total peripheral resistance increase. Renal diuresis and extravascular fluid shifts can lead to a considerable loss of intravascular volume, thus decreasing systemic perfusion. A more detailed description of the pathophysiologic effects of systemic hypothermia is provided in Chapter 5: Accidental Hypothermia. The following section pragmatically describes the effects of the pathophysiologic changes that accompany cold-water immersion and the impact of these changes on survival.
Phase 1: the Cold Shock Response (0 to 2 Minutes)
The cold shock response occurs within the first few minutes of cold-water immersion and depends on the extent and rate of skin cooling. The responses are generally those that affect the respiratory system, heart, and the body’s metabolism (Figure 6-5).36,175 Rapid skin cooling initiates an immediate gasp response, an inability to hold one’s breath, and hyperventilation. The gasp response may cause drowning if the head is submersed during the initial entry into cold water. The subsequent inability to hold one’s breath may further potentiate drowning in high seas. Subsequent hyperventilation will normally diminish within seconds to minutes, but it could be increased and exaggerated indefinitely as a result of emotional stress and panic. Uncontrolled hyperventilation can cause numbness, muscle weakness, or fainting, which may lead to drowning. Any of these respiratory responses can lead to pulmonary aspiration of water. Panic may ensue, with subsequent drowning. These responses may be viewed on several video links listed in the Video Resources section at the end of this chapter.

FIGURE 6-5 Possible cold shock responses. ↑, Increase; ↓ decrease.
(From Edmonds C, Lowry C, Pennefather J: Cold and hypothermia. In Diving and subaquatic medicine, Oxford, UK, 1992, Butterworth-Heinemann.)
Skin cooling initiates peripheral vasoconstriction as well as increased cardiac output, heart rate, and arterial blood pressure. Increased workload on the heart may lead to myocardial ischemia and arrhythmias, including ventricular fibrillation. Sudden death can occur either immediately or within a matter of minutes after immersion as a result of syncope or convulsions, which can lead to drowning, vagal cardiac arrest, or ventricular fibrillation in susceptible individuals.*
Cold shock can occur in water colder than 20° C (68° F), with symptoms increasing as water temperature decreases to freezing. Staged entry into cold water attenuates respiratory responses because of a gradual increase in thermal stimulation. The best advice to combat this phenomenon is, when possible, to control entry into cold water by entering slowly and most importantly by keeping the head from being submersed. One should never dive into cold water! After cold-water immersion, it is important to focus on surviving the first minute by not panicking and by consciously getting one’s breathing under control. Figure 6-6 shows that minute ventilation and breathing frequency increase dramatically with rapid immersion in cold water. These responses are blunted by more than 50% with graded immersion.
Phase 2: Cold Incapacitation (5 to 15 Minutes)
For those who survive the cold shock response, significant cooling of peripheral tissues—especially in the extremities—continues, with most of the effect occurring during the first 15 minutes of immersion. This cooling has a direct deleterious effect on neuromuscular activity.186 This effect is especially significant in the hands, where blood circulation is negligible,34 which leads to finger stiffness, poor coordination of gross and fine motor activity, loss of power,* and swim failure (Figure 6-7, online). It has been shown that this effect is primarily the result of peripheral rather than central cooling.63 The loss of motor control makes it difficult if not impossible to execute survival procedures, such as grasping a rescue line or hoist. The ultimate cause of death is drowning, either through the failure to initiate or maintain survival performance (i.e., keeping afloat, swimming, or grasping onto a life raft) or excessive inhalation of water under turbulent conditions.
These phenomena have obvious survival implications. It is of course advisable to avoid cold-water exposure completely. If cold-water immersion occurs, it is best to quickly determine and execute a plan of action: (1) try to enter the water without submersing the head; (2) escape (i.e., pull oneself out of the water, inflate, and board a life raft); (3) minimize exposure (i.e., get as much of one’s body as possible out of the water and onto a floating object); (4) ensure flotation if one must remain in the water (i.e., don or inflate a PFD); and (5) call for assistance (i.e., activate signaling devices). It may be difficult to execute these actions when cold shock responses predominate. However, after respiratory effects are under control, immediate action should be taken. If self-rescue is not possible, actions to minimize heat loss should be initiated by remaining as still as possible in the heat escape lessening position (HELP), in which the arms are pressed against the chest while the legs are pressed together, or by huddling with other survivors (Figure 6-8, online).86 Drawstrings should be tightened in clothing to decrease the flow of cold water within clothing layers. Many of these responses can be viewed on several video links in the Video Resources section at the end of this chapter.
Phase 3: Onset of Hypothermia (Immersion of More Than 30 Minutes)
Most cold-water deaths likely result from drowning during the first two phases of cold-water immersion, as discussed previously. In general, true hypothermia usually only becomes a significant contributor to death if immersion lasts more than 30 to 60 minutes. A recent survey indicates that even medical practitioners greatly underestimate the time required for a person to become hypothermic in cold water.47 In fact, 75% of respondents felt that a properly clothed adult would become hypothermic after less than 15 minutes in ice water (0° C [32° F]) (Figure 6-9, online). An individual who survives the immediate and short-term phases of cold-water immersion faces the possible onset of hypothermia, because continuous heat loss from the body eventually decreases Tco. Many predictive models to determine the Tco response to cooling are based on the relationships between body composition, thermoregulatory response (i.e., shivering thermogenesis), clothing and insulation, water temperature, and sea conditions (see Cold-Water Survival, later).*
Even in ice water, a victim may not drown when he or she becomes unconscious as a result of hypothermia (≈30° C [86° F]) if a PFD is worn or if some other factor prevents the need for vigorous exercise to prevent drowning. If the head is kept above water, the victim could still survive for up to 1 hour before the heart stops as long as the sea is relatively calm and waves do not wash over the mouth. It is important for members of the public to be educated about the fact that they are not necessarily going to die if they are suddenly immersed in cold water (Box 6-2).
BOX 6-2 The 1-10-1 Principle
If you fall into ice-cold water, remember that you have 1 minute—10 minutes—1 hour.*
The relative contributions of the first three phases to death in cold water of varying temperatures are estimated schematically in Figure 6-10. In colder water, if no flotation is worn (top panel), death occurs as a result of cold shock or cold incapacitation. Only if flotation is worn (bottom panel) will a victim survive long enough to die as a result of hypothermia (Box 6-3).
Phase 4: Circum-Rescue Collapse
Anecdotal cases of fatalities among immersion hypothermia victims have been noted during the immediate pre- and post-rescue periods, despite the survivor being recovered in an apparently stable and conscious condition. The hypothermic victim may experience symptoms that range from fainting to cardiac arrest. These events are often referred to as cases of “rewarming shock” or “post-rescue collapse.” Golden and colleagues73 noted that deaths can occur either shortly before rescue, during rescue, or after rescue, and they have used the term circum-rescue collapse to describe these events. Deaths have occurred within minutes before rescue, while climbing out of the water, while being hoisted onto a helicopter, within a few minutes of entering a warm compartment of a rescue vessel, 20 to 90 minutes after rescue, in a hospital after transport, and during the 24-hour period after rescue.71,73,100,116
Three causes of circum-rescue collapse have been proposed: (1) afterdrop, which is a continued drop in Tco after recovery; (2) collapse of arterial pressure (Figure 6-11, online); and (3) factors that potentiate the risk of ventricular fibrillation (e.g., hypoxia, acidosis, rapid changes in pH). These causes are discussed further later in this chapter.

FIGURE 6-11 Heart rate and blood pressure responses during the vertical removal of a test subject from cold water.
(From Golden FS, Hervey GR, Tipton MJ: Circum-rescue collapse: collapse, sometimes fatal, associated with rescue of immersion victims, J Roy Nav Med Serv 77:139, 1991.)
Afterdrop is a well-known phenomenon that occurs after the removal of a victim from cold-water immersion. Tco continues to decline (i.e., afterdrop) in both animate and inanimate objects, although cold-water immersion has ended.* Afterdrop of as much as 5° to 6° C (9° to 10.8° F) has been observed in humans (Figure 6-12, online).6,43,161 This afterdrop was long proposed to be the major cause of post-rescue death, because further cooling of the heart might result in a temperature at which ventricular fibrillation or cardiac arrest could occur.13
Golden and colleagues73 discount the importance of this phenomenon and propose the collapse of arterial pressure as the cause of death during rescue. They correctly point out that afterdrop that occurs in victims who were hypothermic yet warm enough to climb on board a ship without assistance would be unlikely to cause enough myocardial cooling to result in cardiac arrest or ventricular fibrillation. Those clinicians also propose that removal from cold water results in a precipitous fall in blood pressure, inadequate coronary blood flow, and myocardial ischemia, which possibly precipitate ventricular fibrillation. This mechanism is likely the main factor in both pre-rescue collapse and collapse that occurs during rescue. A fall in blood pressure before removal from the water may be the result of reduced sympathetic tone or catecholamine secretion when rescue is imminent. Hypotension during and shortly after rescue may be caused by a sudden decrease in hydrostatic pressure on the body (i.e., removal of the hydrostatic squeeze of water pressure that exists during immersion), hypovolemia, or impaired baroreceptor reflexes. However, this mechanism cannot fully account for all of the other post-rescue deaths that occur 20 minutes to 24 hours after rescue.
The importance of continued myocardial cooling cannot be discounted. Fibrillation of a cold heart can be initiated by mechanical stimuli, hypoxia, acidosis,131 and rapid changes in pH.101 In dogs, myocardial cooling from 30° to 22° C (86° to 71.6° F) has been shown to cause a five-fold decrease in the electrical threshold for ventricular fibrillation.25 It is also likely that an increase in the rate of myocardial cooling may stimulate fibrillation. On the basis of the large afterdrop values described previously, it is plausible that continued Tco cooling in significantly hypothermic victims could result in ventricular fibrillation, caused primarily by spontaneous fibrillation of the cold myocardium or secondary to a cold-induced increase in sensitivity to other fibrillation stimuli.
Factors That Affect Cooling of the Body’s Core
Normal body Tco fluctuates around 37° C (98.6° F). The clinical definition of hypothermia is a Tco of 35° C (95° F) or lower; however, any exposure to cold that lowers the Tco to less than a normal level results in the body becoming hypothermic. Although various temperatures and terms have been used to classify different levels of hypothermia, the following classifications (Figure 6-13) will be used here. With mild hypothermia (Tco = 32° to 35° C [89.6° to 95° F]), thermoregulatory mechanisms continue to operate fully, but ataxia, dysarthria, apathy, and even amnesia are likely. With moderate hypothermia (Tco = 28° to 32° C [82.4° to 89.6° F]), effectiveness of the thermoregulatory system (i.e., shivering thermogenesis) diminishes until it fails; there is a continued decrease in the level of consciousness, and cardiac dysrhythmias may occur. With severe hypothermia (Tco <28° C [82.4° F]) consciousness is lost, shivering is absent, acid–base disturbances develop, and the heart is susceptible to ventricular fibrillation or asystole. Death from hypothermia is generally a result of cardiorespiratory failure.

FIGURE 6-13 Classification of levels of hypothermia on the basis of core temperature and clinical presentation.
If a victim of cold-water immersion can avoid drowning during the initial few minutes after water entry, the prevention of hypothermia becomes an important problem. Survival time in cold water, which is based on the pathophysiologic effects of decreasing Tco, is not a precise calculation. Large individual variations among survivors with regard to body morphology and state of health and fitness, in combination with many exogenous variables that affect cooling rate (e.g., clothing, water temperature, sea state, flotation, behavior), preclude exact predictions of survival time. However, sufficient experimental data and case history findings exist to allow for generalizations. At a Tco of 34° C (93.2° F), there is a significant deleterious effect on manual dexterity and “useful function” in cold water.24,132 If a survivor is trying to combat rough seas, this level of dysfunction may potentiate drowning. At a Tco of 30° C (86° F), unconsciousness is probable. Even if a survivor is wearing a self-righting PFD that has been designed to maintain airway freeboard in an unconscious person, drowning is probable at this Tco in all but the calmest seas. At a Tco of less than 25° C (77° F), ventricular fibrillation or asystole often occur spontaneously.29 Of these three Tcos, 30° C (86° F) is the most practical for defining the limits of survival in cold water.*
Water Temperature and Sea State
These variables are critically important to the intensity of the cold shock response, the onset of physical and mental impairment, and the rate of Tco cooling. Figure 6-14 graphically illustrates the inverse linear relationship between water temperature and Tco cooling rate.82 Water conducts heat away from the body approximately 25 times as fast as does air at the same temperature.125
Thermal Protection
A large number of studies over the past few decades have evaluated the relationships of different types of protective clothing with heat loss and cooling rates.† Nearly all of these studies have been conducted in calm water or in laboratory settings. As illustrated by the cooling rate data in Tables 6-2 and 6-3, such studies have generally shown that, in calm water, intact “dry” and insulated garments provide better protection than do “wet” insulated garments and that well-insulated garments provide significantly better protection than do poorly insulated garments. Figure 6-15, online shows various types of cold-water protective garments.
TABLE 6-2 Mean Linear Cooling Rates for Lean Men Dressed in Various Types of Garments in Calm Water at 10° C (50° F)
Type of Protective Clothing | Cooling Rate Given as ° C/hr ± Standard Deviation (° F/hr ± Standard Deviation) |
---|---|
Control (equivalent to ordinary street clothes) | 3.2 ± 1.1 (5.8 ± 2.0) |
“Wet” Design | |
Thermal float coat (loose-fitted, 5.4-mm [0.21-inch] closed-cell, foam-insulated jacket) | 1.6 ± 0.6 (2.9 ± 1.1) |
Short wet suit (custom-fitted, 3.2-mm [0.13-inch] closed-cell, foam that covers the arms, the trunk, and the upper thighs) | 1.2 ± 0.4 (2.2 ± 0.7) |
Insulated coveralls (loose-fitted, 3.2-mm [0.13-inch] closed-cell foam that covers the extremities and the trunk) | 1 ± 0.4 (1.8 ± 0.7) |
Full wet suit (custom-fitted, 4.8-mm [0.19-inch] closed-cell foam that covers the extremities and the trunk) | 0.7 ± 0.3 (1.3 ± 0.5) |
“Dry” Design | |
Immersion suit (loose-fitted, 4.8-mm [0.19-inch] closed-cell foam with sealed openings) | 0.5 ± 0.3 (0.9 ± 0.5) |
From Steinman AM, Hayward JS, Nemiroff MJ, et al: Immersion hypothermia: Comparative protection of anti-exposure garments in calm versus rough seas, Aviat Space Environ Med 58:550, 1987.
TABLE 6-3 Comparison of Mean Cooling Rates for Thin Men (Mean Body Fat = 9.1%) Wearing Various Types of Protective Clothing in Calm Water at 11.8° C (53° F)

Although calm-water studies have value in that they compare the relative degree of protection afforded by different types of protective clothing, many immersion accidents occur in rough water.143,168,169 In this environment, a survivor’s cooling rate may be affected by swimming to maintain airway freeboard, passive body movements caused by waves, flushing of cold water through “wet” suits, and leakage of cold water into “dry” suits. For example, subjects in a wave tank demonstrate higher energy expenditure and faster cooling rates than do subjects in calm water.81 Several experimental studies have demonstrated significantly faster cooling rates for human volunteers wearing “wet” protective garments in rough or turbulent water142,158,203 as compared with persons in calm water. Even “dry” suits have shown degradation of protection in rough water. In a study of dry immersion suits in 16° C (60.8° F) water, Ducharme and Brooks found that wave heights of up to 70 cm (28 inches) resulted in a 14% decrease in total suit insulation and an average 45% decrease in thermal resistance at the head and trunk regions of the suits.31
Figure 6-16 shows a comparison of cooling rates for lean males dressed in the various types of protective clothing shown in Figure 6-15 in both calm and rough waters at approximately 10° C (50° F).158 The most dramatic differences occurred in the loose-fitted “wet” protective clothing (e.g., float coat, insulated coveralls), where cooling rates nearly doubled in rough seas as compared with those seen in calm seas. This was primarily caused by the flushing of cold water through the garments. However, even the tight-fitted full “wet” suit allowed for a 30% faster cooling rate in rough water as compared with that seen in calm water. The “dry” suit, which did not leak, showed no significant difference between calm and rough seas.
Estimated survival times in rough seas, on the basis of experimental data, were published for thin males wearing different types of protective clothing in 6° C (42.8° F) water.159 Table 6-4 shows these times for three different levels of survival. Underlying these estimations are the following assumptions:
TABLE 6-4 Estimated Survival Times for Lean Subjects (Mean Body Fat = 11.1%) Wearing Various Types of Protective Clothing in Rough Seas

By comparing these estimated survival times with those of Figure 6-17 (i.e., calm-water survival times), the reader must recall that Figure 6-17 concerns only survival to a Tco of 30° C (86° F). Furthermore, the zone in the graph must be adjusted downward for rough seas and for survivors wearing only lightweight clothing, and it should be adjusted upward for survivors wearing insulated clothing. For 6° C (42.8° F) water, the survival times (to a Tco of 30° C [86° F]) correlate well between Figure 6-17 and Table 6-4. We must emphasize that the estimates in Table 6-4 pertain to lean individuals with a mean body fat level of only 11.1%. Because many populations of adults (e.g., offshore oil workers) in the 30- to 50-year-old age range average 25% to 30% body fat, the estimates of “time to unconsciousness” and “time to cardiac arrest” must be considered conservative for a broader spectrum of adults.
A recent video production called Cold Water Boot Camp (see Video References section at the end of this chapter) tested various flotation ensembles, including closed-cell and inflatable PFDs, heavy dry suits, and lighter extended-wear (paddling) dry suits. Figure 6-18 schematically shows that Tco and survival time decrease as the amount of thermoprotection and flotation decreases (Box 6-4).
BOX 6-4 Controversy Box
Flotation Clothing Impedes Escape from Submersed Vehicles
In many winter road jurisdictions, workers are mandated to wear thermoprotective flotation jackets or overalls. Some workers resist wearing this clothing because of fears that clothing buoyancy may impede exit by forcing the occupant upward against the roof of the vehicle and that increased bulk may impede passage through a window or hatch. Flotation jackets or overalls do not pose an exit threat and should be considered safe for ice road use. Inflatable PFDs have been considered as an option, but, because they may be inflated prematurely before exit, they might impede exit and are not recommended for vehicle use in any situation.59
Body Morphology (Size and Composition)
Children cool faster than adults because children have a greater surface area–to–mass ratio. Cooling rate is generally proportional to surface area, whereas the amount of heat that can be lost is proportional to mass. Thus, a large surface area–to–mass ratio favors cooling. Similarly, smaller adults generally cool faster than do larger adults, and tall, lanky individuals cool faster than do short, stout individuals. Body composition is also important. Subcutaneous fat is a very efficient insulator against heat loss, and cooling rate is inversely related to skinfold thickness. For example, subjects in the tenth percentile for skinfold thickness wearing light clothing in 5° C (41° F) water have nine times the cooling rate of subjects in the ninetieth percentile for skinfold thickness.132 Figure 6-19 shows the linear relationship between change in Tco and mean skinfold thickness.101 Shivering, which is a primary defense against Tco cooling, also varies with skinfold thickness. At a given skin temperature, the shivering response is less in humans with greater amounts of subcutaneous fat.108 In moderately cold water temperatures (18° to 26° C [64.4° to 78.8° F]), Tco cooling has been shown to proceed at the same rate in high- and low-fat individuals because of greater shivering thermogenesis in the low-fat subjects. However, at colder water temperatures (8° C [46.4° F]), Tco cooling is attenuated by greater amounts of subcutaneous fat and body mass as a result of increased insulation.53
In general, for a survivor who is immersed in cold water, the Tco cooling rate is fairly linear after Tco begins to decline. This has been shown to be true for mildly hypothermic experimental subjects. The only data that exist for severely hypothermic humans are those from the infamous Dachau concentration camp atrocities, in which conscious victims were inhumanely cooled to death in ice water.2 Because these unfortunate victims were emaciated and ill, these data do not apply to healthy cold-water immersion subjects and should be considered atrocious.
The advantage of body fat as an insulator against cold has been discussed previously in the section about pathophysiology and cooling rates. With the use of an extrapolation of a linear cooling rate to 30° C (86° F), Figure 6-17 shows predicted calm-water survival times of lightly clothed, nonexercising humans in cold water. The graph shows a line for the average survival expectancy. A broad zone indicates the large amount of individual variability associated with different body sizes, builds, and degrees of fatness. The zone would include approximately 95% of the variation expected for adult and teenage humans under the conditions specified. For the zone in which death from hypothermia is highly improbable, cold water still potentiates death from drowning as a result of cold shock (as discussed previously) during the first few minutes of immersion, especially for those who are not wearing PFDs. It is important to note that Figure 6-17 discusses only calm-water survival times. Because rough-water conditions decrease survival times, as will be discussed later in this chapter, Figure 6-17 may be useful for estimating maximum survival times for an individual who is immersed in cold water. Search and rescue organizations might find such a maximum survival time helpful, because they often use the longest possible survival time to decide when to terminate a search effort.
Amount of Body Immersed
Because of the difference in thermal conductivity between air and water (as discussed previously), heat loss from body surfaces immersed in cold water is far greater than from body surfaces exposed to cold air, even considering the effect of windchill. Thus, immersed victims should attempt to get as much of their bodies out of the water as possible (Figure 6-20). This issue is discussed in greater detail in Cold-Water Survival, later in this chapter.
Head Immersion
Exposure of the dorsal head to cold water may increase a survivor’s rate of cooling and adversely affect mental performance. Survival may depend on the ability to conduct multiple tasks to avoid drowning and hypothermia. The ability to carry out these tasks involves both physical components, which are affected by local muscle and nerve temperature,63 and mental components, which are affected by brain temperature;50 both may be adversely affected by hypothermia.
Head immersion has traditionally been of concern in survival situations.69 In the absence of water in the airways, heat is lost through the head either via convection (heat loss from the blood that perfuses the scalp) or conduction (direct heat loss through bone and soft tissue).206 One hypothesis predicts substantial heat loss through the head because of abundant scalp vascularity and because the scalp vasculature does not vasoconstrict in response to cold as do superficial blood vessels in other body areas.45 Alternatively, heat loss from the back of the head might be minimal, because dorsal head and neck immersion would only involve an additional 3% to 5% of the body surface area.107 In addition, conductive heat loss directly through the scalp and skull is likely to be minimal.206
A few studies have addressed the head cooling of animals in water22 or of humans in cold air,45,139 but only one human study has included cold-water exposure of the head. Alexander reported the data regarding head cooling from studies carried out on prisoners of war in Dachau during World War II.2 These studies were horrific, reprehensible, and grossly unethical, and the results are considered invalid and unusable because of the emaciated condition of the prisoners as well as questions regarding the protocol and accuracy of the results.
Few experimental data are available to evaluate the effects of mild hypothermia on mental performance. A few medical studies have evaluated the impairment of memory among divers during cold-water dives.9,190,193 Two other studies involved cognitive testing during hypothermia without direct head cooling.19,50
Lockhart and colleagues111 recently evaluated the effects of dorsal head and neck immersion among human subjects immersed in 10° C (50° F) water on Tco cooling rates and mental performance. Dorsal head and neck immersion (with the body insulated) resulted in a cooling rate of 0.4° ± 0.2° C/hr (0.7° ± 0.4° F/hr). Body immersion with the head, neck, and upper thorax out of the water resulted in a cooling rate of 1.5° ± 0.7° C/hr (2.7° ± 1.3° F/hr). Immersion of both the whole body and the dorsal head and neck nearly doubled the cooling rate to 2.8° ± 1.6° C/hr (5.0° ± 2.9° F/hr) (p <0.0002). In addition, there were significant correlations between diminished cognitive performance and decreasing Tco. The time required to correctly complete the Stroop Color-Word Test increased as Tco decreased (p <0.001). The number of correct responses decreased with Tco for digit symbol coding (p <0.02), backward digit span (p <0.05), and paced auditory serial addition testing (p <0.05). Figure 6-21 demonstrates the effects of different PFDs on the position of the head in water.
Giesbrecht and co-workers46 repeated the head-cooling study with the use of subjects immersed in 12° C (53.6° F) whose shivering thermogenesis had been inhibited with intravenous meperidine to model severe hypothermia.67 When the body was insulated, there was no Tco cooling with the head out of the water, and immersing the dorsum of the head had no further effect. However, when the body was not insulated but instead exposed to the cold water, Tco decreased at 3.6° C/hr (6.5° F/hr). In this condition, also immersing the dorsum of the head significantly increased the rate of Tco cooling to 5.0° C/hr (9° F/hr) (Figure 6-22).
These results did not confirm the supposition of proportionately greater heat loss from the head. The measured heat loss from the head in both head-immersed conditions was only about 60 kJ as compared with 18 kJ to 33 kJ in the two head-out conditions. By contrast, total body heat loss in the body-exposed configurations was about 1100 kJ and 1260 kJ, respectively, for head-out and back-of-the-head-in conditions. Thus the head accounted for only about 3% and 5% of the total body heat loss, respectively, in the body-exposed conditions. The majority of the difference in heat loss in the body-immersed conditions came from immersion of the anterior thorax rather than from the dorsal head and neck. Nevertheless, it is important to recognize that, although there was not a significant increase in heat loss through the head, there was a disproportionate increase in Tco cooling. Although the mechanisms for this disparity have not yet been determined, the implications are clear for manufacturers and survivors. During long-term cold-water immersion, it is advantageous to keep the head out of the water. If this is not possible (i.e., most survival suits place the victim in a supine position), the survival ensemble should include a hood with as much insulation as is practical (Box 6-5).
Behavior and Posture of the Body in Cold Water
Behavioral variables also affect Tco cooling rate. Hayward and colleagues used infrared thermography to demonstrate that, despite marked peripheral vasoconstriction, heat losses are high in the groin, the lateral and central thorax, and the neck.85 In the groin and neck, which are regions with relatively thin layers of peripheral soft tissue, blood flow through the large and relatively superficial femoral vessels, carotid arteries, and jugular veins potentiates heat flow to the cold water. In the lateral and central thorax, the relative absence of tissue (muscle and subcutaneous fat) insulation in combination with the high thermal conductivity of rib bone potentiates heat loss from the relatively warm lungs to the cold environment. Furthermore, exercise or excessive movement in the water greatly increases heat loss from active muscles.
The effect of activity on total heat balance depends on the balance among the many factors illustrated in Figure 6-23. In normothermic circumstances, heat produced locally in peripheral muscles is transferred to the core via venous return. By contrast, during cold-water immersion, physical activity may actually increase heat loss through increased blood flow to the periphery. This is especially true when immersed victims engage in excessive movement in the water (e.g., swimming, performing the vigorous extremity movements that are necessary to maintain airway freeboard in rough seas).
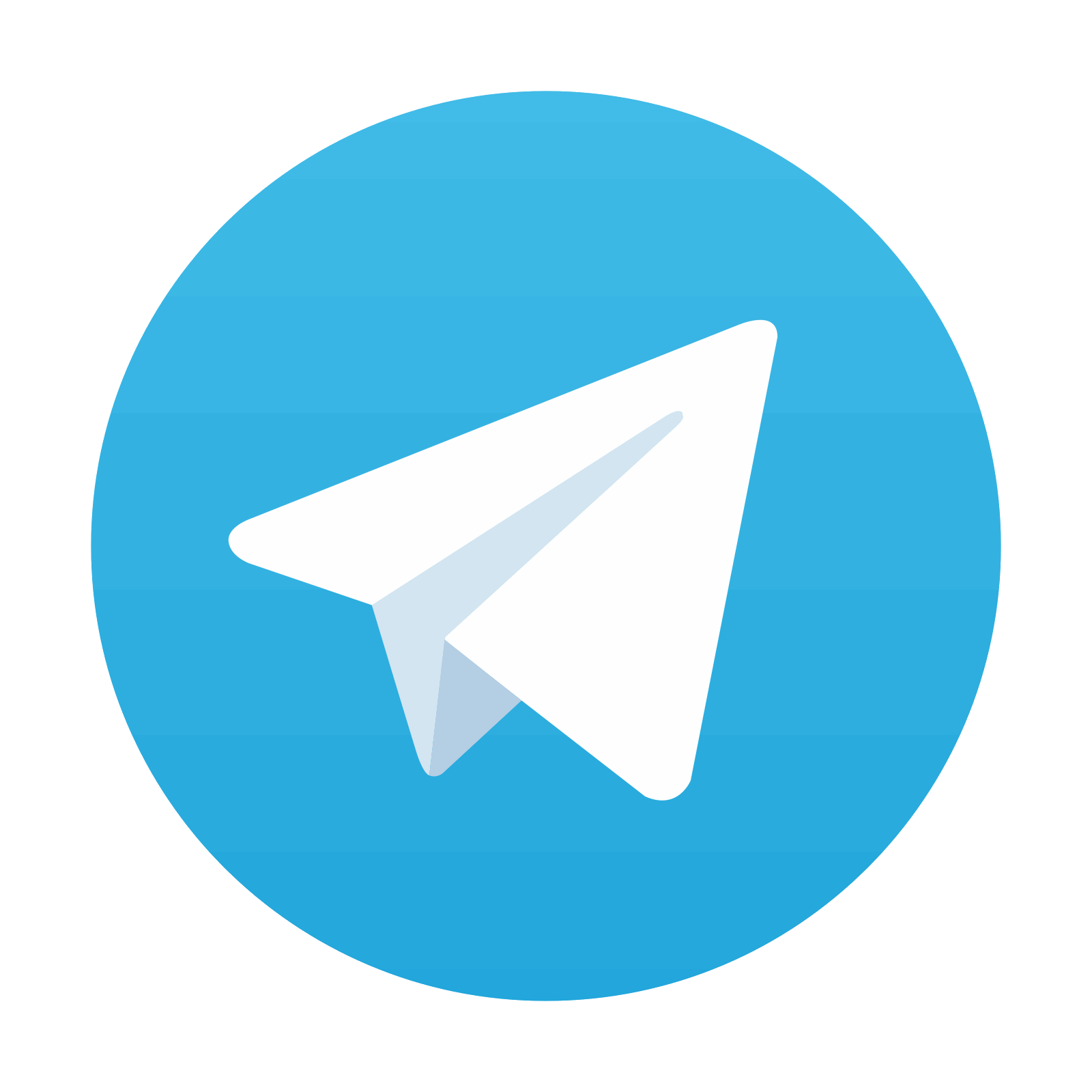
Stay updated, free articles. Join our Telegram channel

Full access? Get Clinical Tree
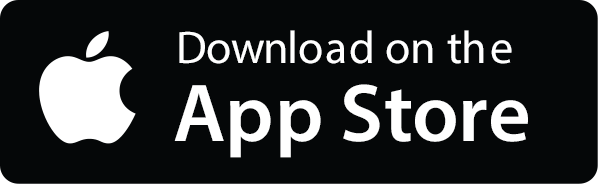
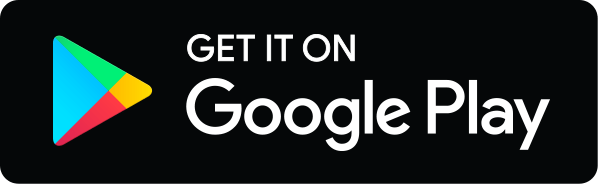