Chapter 78 Hyperbaric Medicine
For online-only figures, please go to www.expertconsult.com
Portable hyperbaric chambers are available for use in remote wilderness settings; however, their use is currently limited to diving injuries and altitude illness. The use of HBOT has been proposed for other applications related to wilderness medicine, such as frostbite, brown recluse spider bites, and, most recently, heatstroke (Box 78-1). However, few data exist to support the routine use of HBOT for these indications. Additionally, HBOT has been suggested as an adjunct to field management of combat trauma in remote settings.
History of Hyperbaric Medicine
Treatment with compressed air therapy dates back to 1662, when the British clergyman Henshaw treated acute medical disorders with increased pressure and treated chronic disorders with decreased pressures.163 Compressed air was used to treat caisson disease, or decompression sickness (DCS), in the late 1800s. Medical use of hyperbaric oxygen (HBO) began in the 1930s with the first systematic studies on the physiologic effects of oxygen.8 Extensive research on oxygen toxicity and safe oxygen limits was conducted during the 1940s, when oxygen was incorporated into the treatment tables of the U.S. Navy (USN) for diving injuries.
True clinical hyperbaric medicine began in the 1950s and 1960s, with the report of HBOT use for anaerobic infections, including gas gangrene and clostridial infections, and for carbon monoxide poisoning.24,164 During the 1970s, HBOT was used widely to treat a variety of conditions with no scientific basis. In 1976, the Undersea Medical Society (UMS) established a multidisciplinary committee to review research and clinical data on the uses of HBOT. Every 3 years, the committee publishes a critical review of the approved indications for HBOT (Table 78-1).57 In 1986, the UMS changed its name to the Undersea and Hyperbaric Medical Society (UHMS) to incorporate the expanding field of hyperbaric medicine.
TABLE 78-1 Approved Indications for Hyperbaric Oxygen Therapy
Indication | Treatment Profile | Number of Treatments |
---|---|---|
Air or gas embolism | USN Table 6 or 6A | 1-10 |
Repeat treatment with USN Table 5, 6, or 2.4 ATA for 90 min | ||
Carbon monoxide poisoning | 2.4-3.0 ATA for 90-120 min | 1-5 |
Clostridial myositis and myonecrosis (gas gangrene) | 3.0 ATA for 90 min TID in first 24 hr, then BID for 2-5 days | 3-10 |
Crush injury, compartment syndrome, and acute ischemia | 2.0-2.5 ATA for 90-120 min | 2-12 |
Decompression sickness | USN Table 6 | 1-10 |
Repeat treatment with USN Table 5, 6, or 2.4 ATA for 90 min | ||
Arterial insufficiencies | ||
Central retinal artery occlusion | 2.0-2.8 ATA for 90 min BID | 6 |
Consider USN Table 6 if no improvement | ||
Enhancement of healing in select problem wounds | 2.0-2.5 ATA for 90-120 min | 10-30 |
Severe anemia | 2.4-3.0 ATA for 90-120 min | No data |
Intracranial abscess | 2.0-2.5 ATA for 60-90 min | 10-20 |
Necrotizing soft tissue infection | 2.0-3.0 ATA for 90-120 min | 5-30 |
Refractory osteomyelitis | 2.0-2.5 ATA for 90-120 min | 20-40 |
Delayed radiation injury and soft tissue and bony necrosis | 2.0-2.5 ATA for 90-120 min | 30-60 |
Compromised grafts and flaps | 2.0-2.5 ATA for 90-120 min BID initially until graft/flap appears stable | 10-20 |
Acute thermal burn injury | 2.0-2.5 ATA for 90-120 min TID in first 24 hr, then BID | 20-40 |
ATA, Atmospheres absolute; BID, twice a day; TID, three times a day; USN, U.S. Navy.
Mechanisms of Hyperbaric Oxygen
Effects of Hbo on Oxygen Content
The oxygen content of blood is the sum of oxygen carried by hemoglobin and oxygen dissolved in the blood plasma. At 1 atm at sea level, 98% of the oxygen content of normal blood is bound to hemoglobin. The oxygen content of blood plasma is determined by the solubility of oxygen in plasma at 37° C (98.6° F), which at a partial pressure of oxygen (PO2) of 100 mm Hg results in oxygen in solution of only 0.31 mL/dL. Once hemoglobin is fully saturated, further increases in oxygen partial pressure can only increase the amount of oxygen physically dissolved in the plasma. As PO2 increases in inspired air, the amount of oxygen dissolved in plasma increases linearly (Figure 78-1). For every atmosphere of pressure increase, 1.8 mL/dL of oxygen is dissolved in plasma. At 3 ATA, approximately 6.8 mL/dL of oxygen can be dissolved in plasma. Because normal oxygen extraction at the tissue level is 5 mL/dL (at normal cardiac output), plasma alone can carry enough oxygen to meet the metabolic needs of the tissues. In addition, this increase in oxygen-carrying capacity dramatically increases the driving force for oxygen diffusion.
Effects of Elevated PO2
The increase in tissue oxygen concentration has important physiologic and biochemical effects in both normal and diseased tissue. These include vasoconstriction, antimicrobial effect, wound healing, and ischemia-reperfusion injury (Box 78-2).
BOX 78-2 Mechanisms of Hyperbaric Oxygen Therapy
Vasoconstriction
Hyperoxia-induced vasoconstriction occurs in both arterial and venous vasculature and has been demonstrated in cerebral, retinal, renal, muscle, and myocardial circulations. Despite reduction in blood flow from vasoconstriction, tissue oxygen partial pressures increase because of the increased amount of oxygen dissolved in plasma.54 Edema reduction occurs because filtration of capillary fluid is decreased, whereas vascular outflow and improved oxygen delivery are maintained. This vasoconstriction can be beneficial in peripheral edema and intracerebral edema and decreases edema in burns and post-ischemic tissues.15,130,135,136 Hence blood flow in the microcirculation is improved through decreasing interstitial fluid pressures from edema reduction. This effect is important in the treatment of brain injuries from carbon monoxide poisoning or arterial gas embolism, spinal cord decompression sickness, and crush injuries.
Antibacterial Effect
HBO has been shown to have a potent inhibitory effect on growth of various microorganisms. HBO causes a direct bactericidal effect on anaerobic bacteria through production of toxic radicals in the absence of free radical degrading enzymes, such as superoxide dismutase, catalases, and peroxidases.78 HBO also has a bacteriostatic effect on selected facultative and aerobic organisms.89 Tissue PO2 of 30 to 40 mm Hg is necessary for oxidative killing of microorganisms by neutrophils. Increased PO2 in infected and hypoxic tissue enhances neutrophil function and return of antimicrobial activity. HBO also augments the bactericidal action of aminoglycosides and potentiates the effect of certain sulfonamides.64,83 Aminoglycoside and cephalosporin antibiotic transport across the bacterial cell wall requires tissue oxygen tensions above 30 mm Hg. Overall, HBO enhances transport and augments antibiotic efficacy.
Wound Healing
The relationship between tissue wound hypoxia and wound healing has been well documented.75,76 Tissue hypoxia decreases fibroblast replication, collagen deposition, angiogenesis, resistance to infection, and intracellular leukocyte bacterial killing, which are all oxygen-sensitive responses essential to normal wound healing. Intermittent elevations of tissue PO2 reverse localized tissue hypoxia and correct the pathophysiology related to oxygen deficiency and impaired wound healing. HBO enhances collagen synthesis, stimulates angiogenesis, improves leukocyte function of bacterial killing, and blunts systemic inflammatory responses.74,76,103 In addition, hyperbaric oxygen stimulates vascular endothelial growth factor (VEGF) release and induces platelet-derived growth factor (PDGF) receptor appearance.17,160,208 The net result of serial increased PO2 from HBOT is improved local host immune responses, clearance of infection, enhanced tissue growth, angiogenesis with progressive improvement in local tissue oxygenation, and epithelialization of hypoxic wounds.
Ischemia–Reperfusion Injury
Ischemia-reperfusion injury (IRI) is tissue damage that occurs after reperfusion of ischemic tissue. IRI is mediated by oxygen-derived free radicals, such as superoxide and hydroxyl free radicals, which are produced during prolonged periods of ischemia followed by reperfusion. These oxyradicals can lead to cell death by lipid peroxidation and generation of further free radicals. Oxyradicals are generated from xanthine oxidase and neutrophils. Neutrophil endothelial adhesion and subsequent release of free radicals play an important role in endothelial and microcirculatory damage.86,152
HBO protects tissues from reperfusion injury. HBO provides additional oxygen for reperfused tissues to generate scavengers such as superoxide dismutase, catalase, peroxidase, and flutathione, which detoxify destructive oxygen radicals before they damage tissues.49 HBO antagonizes the beta2 integrin system, which initiates the adherence of neutrophils to postcapillary venule endothelium.178,180 Reperfusion injury may be inhibited by HBO through a decrease in leukocyte venular endothelial adherence, release of toxic oxygen species, and arteriolar vasoconstriction; hence progressive arteriolar vasoconstriction is inhibited.206 HBO inhibits intracellular adhesion molecule (ICAM-1) expression, which plays a role in neutrophil adhesion and ischemia-reperfusion injury.26 Additionally, HBO upregulates transforming growth factor beta 1 (TGF-β1), which decreases reperfusion injury by upregulating bcl-2 and inhibiting tumor necrosis factor alpha (TNF-α).63 Nitric oxide (NO) plays an important role in IRI, regulating vascular tone and neutrophil adhesion. HBOT appears to increase NO production by inducing nitric oxide synthase (NOS) production.7,26,179 It is possible that the beneficial effect of HBOT in IRI is primarily mediated by NO.25
HBO has been shown to be beneficial in almost all animal models of ischemia-reperfusion studied and results in increased microvascular perfusion in skeletal muscle and improved skin flap survival.97,202,206,207 It may be an important adjunct for treatment of tissue at risk for reperfusion injury that can accompany crush injury, limb replantation, compartment syndrome, and decompression illness. New data suggest a role of HBOT in preventing ischemia-reperfusion injury associated with myocardial infarction and cerebral ischemia.
Types of Chambers
Monoplace Chambers
Monoplace or single-person chambers account for the majority of chambers in the United States. They accommodate a single patient, and the chamber is pressurized either with 100% oxygen or with air while the patient breathes oxygen through a mask or hood. Monoplace chambers are easier to maintain, require less space, and are less expensive than are multiplace chambers. They can be configured with noninvasive and invasive monitoring. Specially adapted ventilators and monitoring systems in some monoplace chambers allow for treatment of critically ill patients. During treatment, there is no direct access to the patient. Therefore, the chamber must be decompressed for an emergency, which can limit its use in some critically ill patients (Figure 78-2). Most monoplace chambers cannot exceed 3 ATA treatment depth.
Multiplace Chambers
Multiplace chambers hold two or more people and allow more than one patient to be treated at a given time, accompanied by attendants, including technicians, nurses, and respiratory therapists (Figure 78-3, online). The chamber is pressurized with air; 100% oxygen is delivered by face mask, with a head tent, or through an endotracheal tube. Multiplace chambers allow for intensive care–level monitoring, mechanical ventilators, vascular pressure monitoring, intravenous infusion pumps, blood gas analysis, and medication administration. Multiplace chambers can maintain a treatment pressure of 6 ATA and are ideal for treatment of diving injuries, particularly arterial gas embolism (AGE). General anesthesia, surgery, chest tube placement, and cardiopulmonary resuscitation have been performed inside multiplace chambers. They have the disadvantage of a risk for DCS to attendants during long treatments.
Portable Recompression Chambers
Altitude Illness
Several portable hyperbaric chambers are available for emergency treatment of severe acute mountain sickness, high-altitude pulmonary edema, and high-altitude cerebral edema, particularly when descent is not feasible or while waiting for evacuation (Figures 78-4 to 78-6; Figure 78-6, online). They are constructed of relatively lightweight fabrics and require no use of portable oxygen. The portable chambers are pressurized with ambient air using manual foot pumps or hand pumps and can simulate a descent of 1500 to 2500 m (5000 to 8200 feet). Hyperbaric treatment should only be used as an emergency measure and does not substitute for descent or evacuation.

FIGURE 78-4 Chinook Medical Gear’s Gamow portable altitude chamber. A, In the field. B, Close-up.
(Courtesy Chinook Medical Gear, Inc.)
Three portable chambers are currently available for use at high altitude. The Gamow bag (see Figure 78-4) was invented by Dr. Igor Gamow at the University of Colorado. It is cylindric, lightweight (the bag, pump, and carrying day pack weigh approximately 7 kg [15 lb]), and easy to use. It measures 2.5 × 0.6 m (8 × 2 feet) and can maintain an internal pressure of 2 psi (104 mm Hg or 0.13 atm). The Certec hyperbaric chamber comprises two bags: an outside envelope made to withstand tension and provide stability and an inside envelope of polyurethane that allows for more durability of the chamber (see Figure 78-5). The Certec chamber weighs 4.8 kg (10.5 lb) and measures 2.2 × 0.65 m (7 × 2 feet); it can be pressurized to a slightly higher inflation pressure (internal pressure of 165 mm Hg or 0.22 atm or 3.2 psi) than can the Gamow bag. This is equivalent to about 800 m (2625 feet) greater simulated altitude descent; however, it is unclear whether this is important clinically. The Portable Altitude Chamber (PAC) is about the same size as the Gamow bag but allows more room at the head and shoulders and has a radial zipper at the head, allowing easier assess for the patient (see Figure 78-6, online). All three chambers have been used successfully at altitude; early symptom relief and improvement in peripheral oxygen saturation and cerebral oxygenation have been reported.4,77 These chambers have no utility in the treatment of diving injuries.
Life Support Technology Group (LST) and the Center for Investigation of Altitude Medicine (CIMA) in Cusco, Peru, have developed a series of Acute Mountain Sickness (AMS) hyperbaric treatment profiles using standard hyperbaric chambers that can be pressurized to 3 ATA.28 These AMS profiles provide rapid pressurization to sea level and beyond, with exposure to hyperbaric oxygen followed by a gradual decrease in ambient pressure while the patient breathes air. LST and CIMA report that these AMS hyperbaric treatments virtually eliminate the AMS rebounding often seen with conventional treatment, in which the patient either remains at altitude or is recompressed back to 1500 to 2438 meters briefly and then returns to the same altitude where AMS symptoms first manifested. These are proposed protocols and are not the standard of care in treating AMS.
Diving Injuries
The SOS Hyperlite Hyperbaric Stretcher is the most widely used collapsible chamber for treatment and transportation under pressure of a patient suffering from DCS or AGE to a permanent recompression chamber (Figure 78-7). The chamber is made of Kevlar and has two removable endplates with communication system and availability to deliver 100% oxygen to the patient. It is capable of a standard treatment depth of 2.82 ATA and weighs approximately 75 kg (165 lb). Also called the Emergency Evacuation Hyperbaric Stretcher (EEHS), it has received certification for use within the U.S. Department of Defense and is currently in use by the U.S. Coast Guard, all four services of the U.S. military, the Russian Navy, and the U.S. space agency, NASA.
Another collapsible and portable hyperbaric chamber is the Italian-made GSE chamber. A smaller version, the Hyperbaric Backpack, is available. This chamber is 76 cm (30 inches) in diameter, 2 m (7 feet) long, and weighs 42 kg (92 lb). It is a double bag of translucent composite polyester and specified for 6 ATA. Another chamber, the Chamberlite 15, is probably the simplest and one of the lightest units on the market. It is capable of providing 100% oxygen at 2 to 2.4 ATA pressure with special adapters. This unit is constructed of foldable polyurethane, has 10 viewing ports, and weighs less than 18 kg (40 lb), thus making it highly portable by standard stretcher or two-person carry. However, depth of treatment is limited, because treatment of diving injuries requires a minimum treatment depth of 2.82 ATA. It has been used in studies on carbon monoxide (CO) poisoning and may be a useful tool for emergency treatment of CO poisoning in the field.161
Contraindications to HBOT
Absolute
HBOT is safe with very few contraindications. Untreated pneumothorax is an absolute contraindication to treatment, because it may progress to a tension pneumothorax during decompression. Once a chest tube has been placed, the patient can safely undergo HBOT. Treatment with bleomycin, disulfiram, and doxorubicin may enhance toxic effects when used concurrently with HBOT and are considered absolute contraindications to HBOT.88
Complications of HBOT
Oxygen Toxicity
In 1878, Bert described seizures and death in animals exposed to 3 to 4 ATA. This central nervous system (CNS) manifestation of oxygen toxicity became known as the Paul Bert effect.12 In 1899, pulmonary oxygen toxicity was described after prolonged exposures to 0.74 to 1.3 atm of oxygen.101 Tissue injury from oxygen is mediated by reactive oxygen species (ROS) that are produced by chemical reactions involving single electron transfers to molecular oxygen or its metabolites. These ROS include superoxide anion (O2−), hydroxyl radial (OH−), and hydrogen peroxide (H2O2).
Under normal oxygen conditions, cells have adequate antioxidant defenses. These defenses include antioxidant enzymes such as superoxide dismutase and catalase, and low-molecular-weight scavengers such as glutathione and vitamin E. As PO2 increases, these defenses are overwhelmed and ROS cause lipid peroxidation, protein oxidation, sulfhydryl depletion, and oxidation of pyridine nucleotides, leading to cell membrane disruption, cell injury, and death.50
Pulmonary Oxygen Toxicity
Symptoms of early pulmonary oxygen toxicity include substernal burning, slight cough, mild dyspnea, and chest tightness and can be seen in patients treated for decompression sickness, air embolism, and gas gangrene when treatments are prolonged. These symptoms resolve after discontinuing therapy. Pulmonary oxygen toxicity is rare using current treatment tables. Patients do not experience significant alteration in pulmonary gas exchange when treated with standard HBO treatment protocols.201
Visual Refractive Changes
Patients who undergo more than 20 HBOT treatments often develop progressive myopia that is temporary and reverses completely within a few weeks after discontinuing HBOT. The exact mechanism is not known but appears to be lenticular in origin.32,104,139 Treatment with HBOT beyond 150 treatments is associated with increased risk for irreversible refractive changes or development of new cataracts. In the United States, HBOT treatments rarely exceed 60 in number.
Practical Aspects of Hyperbaric Treatment
Preparation for Hyperbaric Treatment
Preparation of a hyperbaric patient requires strict attention to certain safety issues and protocols. For fire safety reasons, patients must wear hospital-supplied clothing of either 100% cotton or a blend of 50/50 polyester-cotton. Undergarments must be 100% cotton. Silk, wool, waffle weave, and nylon and other synthetic materials are prohibited. Watches must be inspected for pressure compatibility. Excessive cosmetics, lotions, and perfumes should be removed. Patients must be inspected for matches, lighters, and smoking materials, all of which are prohibited. The hyperbaric staff should follow established protocols at their facility. In general, staff must (1) ensure eustachian tube patency, (2) remove all medical devices not required during hyperbaric treatment, (3) cap all intravenous (IV) catheters that are not in use, (4) empty Foley bag or have patient void before chamber entry, (5) have labeled medications available, (6) confirm a good signal on all monitors and check that monitors and IV pumps are fully charged, and (7) ensure that the ventilator is functioning correctly on the surface. A list of equipment that is prohibited and allowed inside a hyperbaric chamber is presented in Box 78-3.
Manifestations of CNS Oxygen Toxicity
Convulsions due to CNS oxygen toxicity can occur in patients breathing oxygen at pressures of 1.4 ATA or greater. Convulsions may occur abruptly or be preceded by signs of CNS irritability, including facial twitching, diaphoresis, apprehension, visual field constriction, tinnitus, nausea, or vertigo (Box 78-4). Manifestation of any of the premonitory symptoms of CNS oxygen toxicity is treated by switching the patient’s breathing gas from oxygen to chamber air. Once all symptoms have resolved, oxygen therapy can be resumed; however, shortening the duration of oxygen exposure can prevent recurrence of symptoms. A small dose of benzodiazepines, such as IV diazepam 5 to 10 mg, will usually abate CNS oxygen toxicity symptoms if oxygen discontinuation fails to provide relief.
Pediatric Considerations
Generally, indications for HBOT in the pediatric population are similar to those in adult patients. Accidental carbon monoxide poisoning and serious burns are common in pediatric age groups. Invasive procedures can result in iatrogenic cerebral gas embolism in children as in adults. Figure 78-8 shows a 7-day-old infant with clostridial omphalitis from an umbilical vein catheter that developed into gas gangrene of the abdominal wall. The child was treated successfully with HBOT at University of California, San Diego, Hyperbaric Center.147 This center has also successfully treated a child with acute hepatic artery thrombosis after liver transplantation.62
Special attention must be paid to reduce evaporative, conductive, and convective heat losses in the chamber because children have an increased risk for hypothermia. An oxygen hood can be used for most children older than 3 to 4 years. In younger children, the neckdam can be pulled down to waist level, which allows the child’s hands to be free inside the hood (Figure 78-9, online). Although there are no studies on the safe limits of exposure to hyperbaric oxygen for children, in practical experience there is no increased risk for developing pulmonary or neurologic oxygen toxicity. Additionally, there are no significant differences in side effects or morbidity with HBOT for children compared with adults.154 One theoretical problem with HBOT exists for the child with a ductus arteriosus–dependent congenital heart disorder. The increased PO2 could result in ductal constriction, limiting cardiac output, and must be considered when treating an infant with congenital heart disease.191
Approved Clinical Applications of HBOT
Arterial Gas Embolism
AGE can result from pulmonary barotrauma while diving (see Chapter 77). Pulmonary barotrauma typically occurs when a diver ascends rapidly while breath-holding because of panic or running out of air. Rapid expansion of gas causes pulmonary overdistention and disruption of the alveolar capillary membrane. Air enters the pulmonary veins, travels to the heart, and enters the systemic circulation. Air embolism may result from nondiving causes, many of which are iatrogenic. Documented AGE has occurred during central venous catheterization, mechanical ventilation, cardiopulmonary bypass, angioplasty, lung biopsy, percutaneous hepatic puncture, liver transplant, hemodialysis, penetrating chest trauma, oral sex, sexual intercourse after childbirth, transurethral prostatectomy, laparoscopy, arthroscopy, hysteroscopy, and cesarean delivery.* Abnormal neurologic findings after cardiovascular or neurologic surgery or dialysis should make one consider AGE.
AGE manifests with sudden alteration in consciousness, confusion, focal neurologic deficits, cardiac arrhythmias, and death. AGE requires immediate recompression. HBOT reduces the volume of gas bubbles, thereby restoring blood flow, and improves oxygen delivery to ischemic, hypoperfused tissues. HBOT reduces cerebral edema and decreases ischemia-reperfusion injury. Treatment for AGE had traditionally been with USN Table 6A with an initial excursion to 165 feet (6 ATA) for 30 minutes to enhance bubble compression (Figure 78-10). Animal studies showed no additional benefit from compression to 6 ATA compared with 2.82 ATA.98 Current recommendations include initial recompression to 2.82 ATA, breathing 100% oxygen. If there is no improvement or clinical deterioration, deeper recompression to 6 ATA can be instituted. The diver breathes a mixture of 50% nitrogen and 50% oxygen below 2.82 ATA. Repetitive treatments are recommended until there is no further stepwise improvement.122
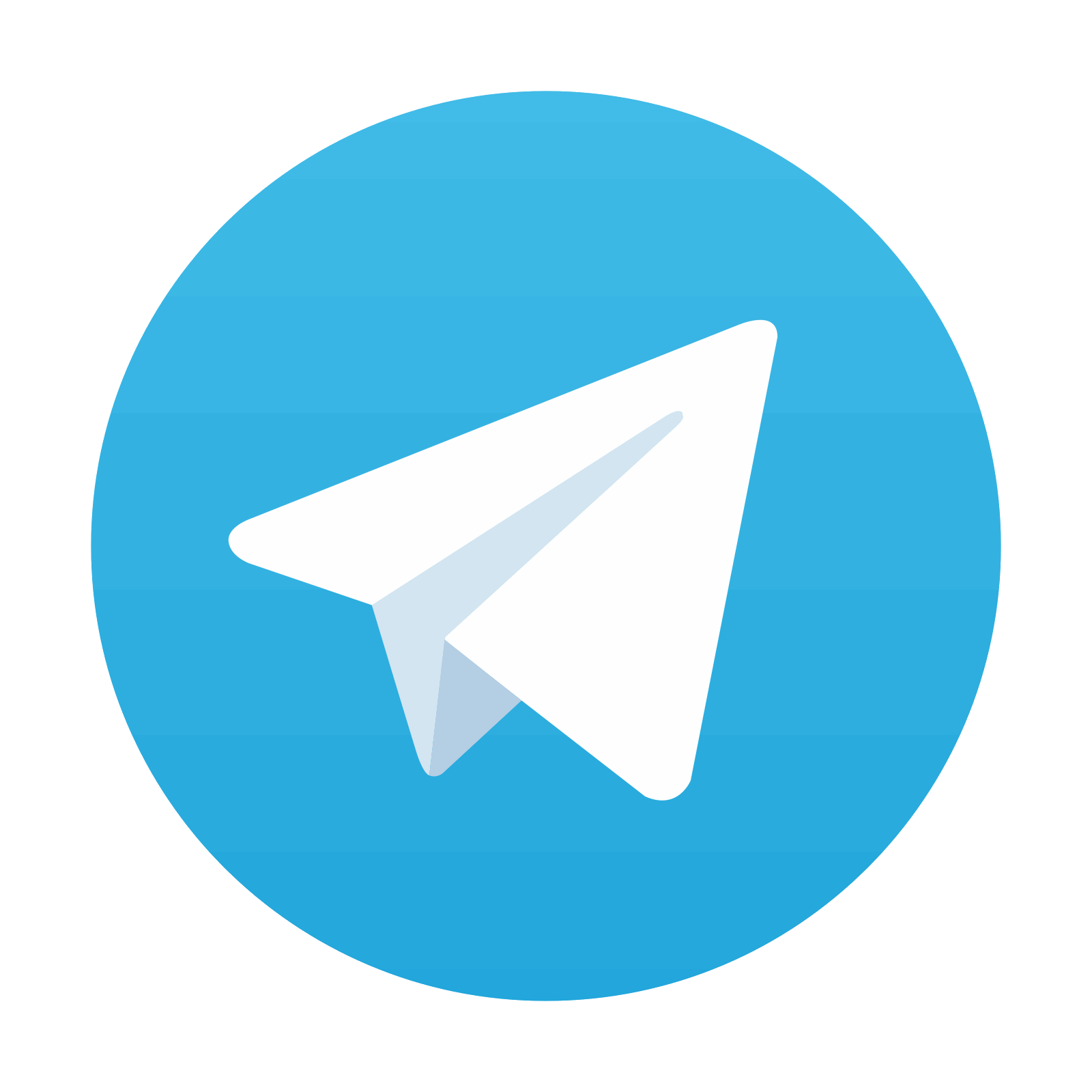
Stay updated, free articles. Join our Telegram channel

Full access? Get Clinical Tree
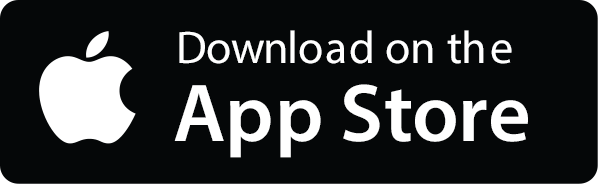
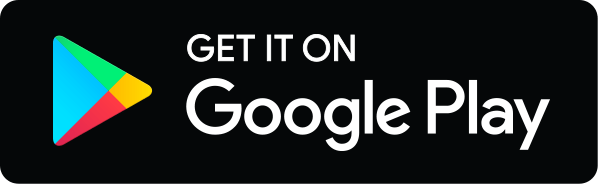