Human Studies of Experimental Pain From Muscle
Peter Svensson
Messoud Ashina
Experimental pain studies in healthy subjects and patients with well-defined pain complaints represent one of many approaches to gaining more insight into the pathophysiologic mechanisms involved in headache as well as in other musculoskeletal pain conditions. In research on tension-type headache, special emphasis has been given to the function of the epicranial, temporomandibular, and neck muscles. This chapter describes human experimental models developed and used specifically to investigate pain originating from muscle tissue.
In general, experimental pain research involves a standardized induction of pain and the appropriate assessment of the responses (Fig. 69-1). The evoked pain responses can be recorded by multiple techniques ranging from simple measures of self-reported pain to advanced psychophysical and electrophysiologic testing, biological markers, and sophisticated imaging of nociceptive processing. The particular assessment technique depends on the specific aim of the experimental study; the reader is referred to recent reviews for more detailed descriptions (23). This chapter focuses on the models available to induce pain in the cervicotrigeminal system, including chemical, mechanical, and electric stimulation and exercise-induced activation of human muscle nociceptors and their contribution to the understanding of muscle pain mechanisms.
CHEMICAL STIMULATION
Hypertonic Saline
Injection of hypertonic saline (4 to 6%) has been by far the most frequently used chemical stimulus in human experimental muscle pain research and therefore is described in detail. A major reason for the popularity of hypertonic saline is the safety and reliability of this technique; no side effects after numerous intramuscular injections have been reported (71). Kellgren (35) was the first to use hypertonic saline to evoke pain in various muscles including the temporomandibular and suboccipital muscles. He noted a rapid increase in pain intensity shortly after a bolus injection of 0.1 mL of 6% saline into the masseter muscle associated with a spread of pain to adjacent regions of the face, including the teeth. The pain peaked after 1 to 2 minutes and faded over a period of 3 to 5 minutes. This preliminary description of one subject was later verified in larger study populations both with bolus injections into the temporalis muscle and masseter muscle (28,63,66, 67, 68). The bolus injection technique has also been refined so that a computer-controlled syringe pump can maintain a continuous slow infusion of hypertonic saline for up to 15 to 20 minutes with relatively constant pain in the temporomandibular muscles (72,79). This type of tonic experimental pain seems to have similar qualities to clinical pain conditions (62) and allows sufficient time for elaborate studies of sensory motor interactions or other physiologic effects of pain (33,71). The osmolarity of the hypertonic saline solution probably contributes to the pain responses by a direct mechanical effect on the terminal endings and sodium channels and/or a release of substance P (SP) (12). Recordings from nociceptive C-fiber afferents from the rat masseter muscle have indeed documented strong activity evoked by injection of hypertonic saline (12). Furthermore, the dominant sensation caused by injection of hypertonic saline is a deep, diffuse pain sensation (71). Interestingly, it has been shown that injection of hypertonic saline in the vicinity of motor end plate regions is associated with higher pain scores than injection in other muscle sites suggesting focal areas within the muscle with a higher density of nociceptors (52). This evidence indicates that hypertonic saline is a potent chemical stimulus for activation of muscle nociceptors.
A particularly interesting feature of intramuscular saline injection is the spread and referral of pain to adjacent regions. Pain induced in the suboccipital muscles
of one subject was perceived as a headache (35), and repeated injections into the temporalis muscle of another single subject caused pain in the neck muscles (57). More recent studies in larger populations showed that pain from the temporalis muscle can be referred to both the upper and lower jaw, ear, and eye region (28,68) and that pain from the masseter muscle is described as being located above the temporomandibular joint, posterior teeth in the upper and lower jaw, and temple region (63,68,72) (Fig. 69-2). The available data do not suggest major differences in the quality or intensity of the pain from hypertonic saline injections into the anterior part of the temporalis or masseter muscles, and the localization of pain is partly overlapping (68). However, major differences in the pain patterns are clear when the posterior part of the temporalis muscle is injected with hypertonic saline because the pain spreads toward the neck, vertex, and temple but rarely toward the lower jaw region (54). Thus, pain patterns evoked by stimulation of the masseter muscle seem to resemble pain patterns reported by patients with temporomandibular disorders and pain patterns evoked by stimulation of the posterior temporalis muscle and neck muscles look similar to pain patterns from patients with tension-type headache (54).
of one subject was perceived as a headache (35), and repeated injections into the temporalis muscle of another single subject caused pain in the neck muscles (57). More recent studies in larger populations showed that pain from the temporalis muscle can be referred to both the upper and lower jaw, ear, and eye region (28,68) and that pain from the masseter muscle is described as being located above the temporomandibular joint, posterior teeth in the upper and lower jaw, and temple region (63,68,72) (Fig. 69-2). The available data do not suggest major differences in the quality or intensity of the pain from hypertonic saline injections into the anterior part of the temporalis or masseter muscles, and the localization of pain is partly overlapping (68). However, major differences in the pain patterns are clear when the posterior part of the temporalis muscle is injected with hypertonic saline because the pain spreads toward the neck, vertex, and temple but rarely toward the lower jaw region (54). Thus, pain patterns evoked by stimulation of the masseter muscle seem to resemble pain patterns reported by patients with temporomandibular disorders and pain patterns evoked by stimulation of the posterior temporalis muscle and neck muscles look similar to pain patterns from patients with tension-type headache (54).
The neurophysiologic mechanisms responsible for the spread and referral of muscle pain are not entirely clear, but are likely to involve central convergence of peripheral afferents onto wide dynamic-range neurons in the dorsal horn and subnucleus caudalis (55). It has also been shown that intramuscular administration of hypertonic saline results in neuronal activity in convergent spinal dorsal horn neurons and in neurons encoding nociceptive information in the nucleus submedius in the thalamus (34). Central sensitization of wide dynamic-range and nociceptive specific neurons and unmasking of new receptive fields owing to the central sensitization are also likely to mediate referred pain (55).
In addition to standardized descriptions of somatosensory changes induced by experimental muscle pain (72), the model with hypertonic saline also can be used to examine the effects of pain on motor function. Muscle dysfunction has long been thought to be an important etiologic factor for the development and maintenance of
myofascial pains. Thus, induction of pain in healthy subjects may provide insight into the cause-and-effect relationship between pain and muscle function, which is difficult to establish from clinical studies of patients with tension-type headache or temporomandibular disorders (71). Experimental pain from the masseter muscle has a profound effect on dynamic repetitive movements such as chewing; that is, the amplitude of the jaw movements are smaller, and there is less electromyographic (EMG) activity in the jaw-closing phase and more EMG activity in the jaw-opening phase, suggesting a guarding and protective effect (66). These experimental results are in accordance with the pain-adaptation model presented by Lund et al. (40). The model with hypertonic saline also shed light on the classic, yet still controversial, problem of increased or nonincreased postural EMG activity in patients with tension-type headache and temporomandibular disorders. Stohler et al. (64) showed a small increase (1 to 2 μV) in the temporalis and masseter muscles during a period of saline-induced pain from the masseter muscle in healthy subjects; however, these authors attributed the small EMG activity recorded by using surface electrodes to contamination from mimic muscles, because similar changes were observed in control experiments in which pain was “imagined.” Svensson et al. (73) used intramuscular electrodes but could not show any relation between pain intensity and EMG changes. Interestingly, it has recently been shown that painful stimulation of the masseter muscle is associated with significant EMG increases in the cervical muscles pointing to functional relationships between pain in the trigeminal and cervical regions (75). Additionally, trigeminal reflex pathways and jaw tremor have been shown to be modulated by saline-evoked muscle pain (25,78), for example the short-latency jaw-stretch reflex is facilitated during jaw muscle pain, which could contribute to a reflexmediated stiffness of the jaw. However, no experimental evidence has so far been found to suggest a long-lasting muscle hyperactivity induced by pain in temporomandibular muscles.
myofascial pains. Thus, induction of pain in healthy subjects may provide insight into the cause-and-effect relationship between pain and muscle function, which is difficult to establish from clinical studies of patients with tension-type headache or temporomandibular disorders (71). Experimental pain from the masseter muscle has a profound effect on dynamic repetitive movements such as chewing; that is, the amplitude of the jaw movements are smaller, and there is less electromyographic (EMG) activity in the jaw-closing phase and more EMG activity in the jaw-opening phase, suggesting a guarding and protective effect (66). These experimental results are in accordance with the pain-adaptation model presented by Lund et al. (40). The model with hypertonic saline also shed light on the classic, yet still controversial, problem of increased or nonincreased postural EMG activity in patients with tension-type headache and temporomandibular disorders. Stohler et al. (64) showed a small increase (1 to 2 μV) in the temporalis and masseter muscles during a period of saline-induced pain from the masseter muscle in healthy subjects; however, these authors attributed the small EMG activity recorded by using surface electrodes to contamination from mimic muscles, because similar changes were observed in control experiments in which pain was “imagined.” Svensson et al. (73) used intramuscular electrodes but could not show any relation between pain intensity and EMG changes. Interestingly, it has recently been shown that painful stimulation of the masseter muscle is associated with significant EMG increases in the cervical muscles pointing to functional relationships between pain in the trigeminal and cervical regions (75). Additionally, trigeminal reflex pathways and jaw tremor have been shown to be modulated by saline-evoked muscle pain (25,78), for example the short-latency jaw-stretch reflex is facilitated during jaw muscle pain, which could contribute to a reflexmediated stiffness of the jaw. However, no experimental evidence has so far been found to suggest a long-lasting muscle hyperactivity induced by pain in temporomandibular muscles.
Brain imaging techniques have also been used to examine the central processing of pain from the temporomandibular muscles. A recent positron emission tomography study showed that saline-evoked masseter pain is associated with significant increases in regional cerebral blood flow (rCBF) in the dorsal-posterior insula, anterior cingulate and prefrontal cortices, right posterior parietal cortex, brainstem, cavernous sinus, and cerebellum, whereas no rCBF changes occurred in the primary (SI) or secondary somatosensory (SII) cortices (37) (Fig. 69-3). Nonpainful von Frey stimulation produced a significant rCBF increase in the contralateral SI face representation, whereas von Frey stimulation in combination with ongoing muscle pain produced mechanical hyperesthesia and significant rCBF increases in the subgenual cingulated and the ventroposteromedial and dorsomedial thalamus. These results suggest that mechanical hyperesthesia has a unique representation in the brain (37). Saline-evoked jaw muscle pain has also been shown to induce regional release of endogenous opioids and activation of μ-opioid receptor system in the ipsilateral amygdala and contralateral ventrolateral portion of the thalamus (81). Furthermore, activation of the μ-opioid system is linked to reductions in sensory
and affective ratings of the jaw muscle pain (81). Studies on the genetic polymorphism of the catechol-O-methyltransferase enzyme have revealed striking associations between genotypes, the endogenous μ-opioid system activation and phenotypic responses such as sensory and affective ratings of jaw muscle pain (80).
and affective ratings of the jaw muscle pain (81). Studies on the genetic polymorphism of the catechol-O-methyltransferase enzyme have revealed striking associations between genotypes, the endogenous μ-opioid system activation and phenotypic responses such as sensory and affective ratings of jaw muscle pain (80).
In conclusion, the model with hypertonic saline may help us to explore peripheral and central mechanisms in various chronic pain conditions including tension-type headache. Brain imaging studies used in combination with hypertonic saline model seem to be a fruitful avenue to study the complex neurobiology and psychophysiology related to muscle pain at a system level.
Endogenous Algogenic Substances
Intramuscular injections of numerous endogenous algogenic substances and neuropeptides also have been used for chemical activation of human muscle nociceptors (Table 69-1). Substantial evidence has been found from studies in animal models that bradykinin and 5-hydroxytryptamine (5-HT) can trigger action potentials in nociceptive group III and IV fibers (42). When injected into the human temporalis muscle, 5-HT does not induce significant levels of pain, and bradykinin induces only relatively low levels of pain (30). However, Ernberg et al. (19,20) have shown that injection of 5-HT into the human masseter causes a significant reduction in pressure-pain thresholds, that is, allodynia to mechanical stimuli. Injections of combinations of 5-HT and bradykinin cause significantly more pain than injections of isotonic saline and a significant reduction of pressure-pain thresholds, a finding that supports the importance of presensitization with 5-HT for bradykinin-induced neural activity (7,8,30). Etype prostaglandins also can sensitize muscle afferents to bradykinin, which releases prostaglandin E2 (PGE2) from tissue cells, thereby potentiating its own action (42). SP has been studied extensively in cutaneous pain, but it does not appear to sensitize muscle nociceptors to mechanical stimuli (42). In itself, SP does not produce pain when injected into the human temporalis muscle (31); however, in combination with calcitonin gene-related peptide (CGRP) and bradykinin, it does induce muscle pain and a significant reduction of pressure-pain thresholds in the temporal muscle (31,49). Recently, an extensive examination of various combinations and concentrations of 5-HT, bradykinin, histamine, PGE2, and adenosine triphosphate (ATP) injected into the trapezius muscles was carried out and this “inflammatory soup” was shown to produce both pain and prolonged tenderness in healthy subjects (45). ATP (> 18,000 nmol/mL) was associated with unacceptable side effects, but did not appear to be essential for the development of pain or tenderness; thus injections of 5-HT (156 nmol), bradykinin (92 nmol), histamine (140 nmol), and PGE2 (1.95 nmol) were suggested to be valuable model for the study of myofascial pain mechanisms (45).
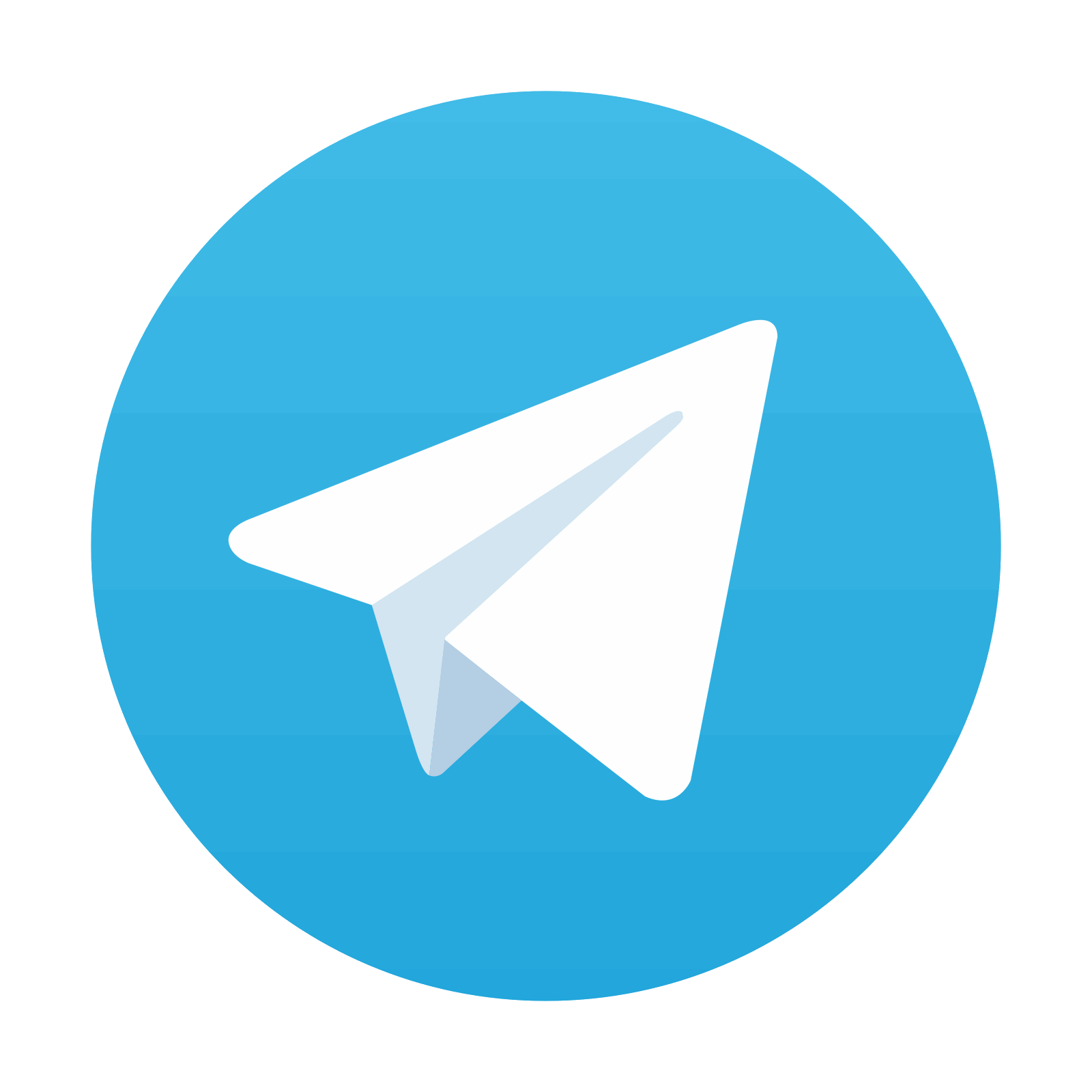
Stay updated, free articles. Join our Telegram channel

Full access? Get Clinical Tree
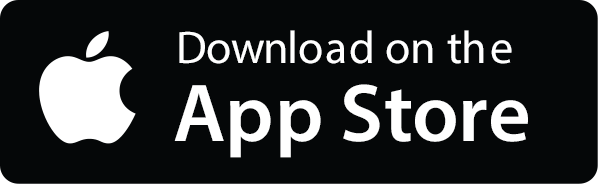
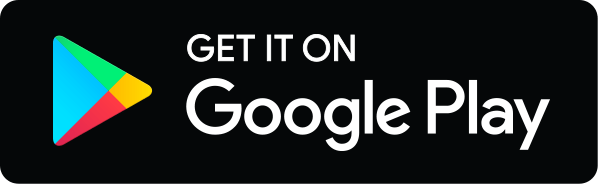