To address the question whether ventilators damage lungs, one must first understand several commonly used related terms. Ventilator-induced lung injury (VILI) denotes acute lung damage that develops during mechanical ventilation. VILI is pathologically characterized by inflammatory-cell infiltrates, hyaline membranes, alveolar hemorrhage, increased vascular permeability, pulmonary edema, loss of functional surfactant, and ultimately alveolar collapse. Ventilator strategies designed to reduce VILI have improved outcomes among patients with acute respiratory distress syndrome (ARDS), characterized by progressive hypoxemia requiring a greater fraction of inspired oxygen to maintain an acceptable arterial oxygenation and chest imaging that demonstrates bilateral interstitial or alveolar opacities. In fact, the clinical presentation of VILI is largely indistinguishable from ARDS, highlighting the clinical importance of VILI. Alveolar overdistension, lung strain, and atelectasis are the key inciting and defining features of VILI. Overdistension reflects the presence of an elevation in transpulmonary pressure, defined as the difference between the airway pressure and the pleural pressure at the end of inspiration. Strain is the ratio of the volume of gas delivered during a tidal breath to the amount of aerated lung receiving that breath: Larger strain is matched by a higher mechanical stress on alveolar structures. When there is heterogeneous consolidation or atelectasis, a disproportionate volume from a given tidal breath is delivered to the open alveoli. The result is regional alveolar overdistension and excessive strain because a normal tidal volume is selectively directed to only a portion of the lung. This process is conceptually akin to delivering a standard tidal volume to a “baby lung.”
Atelectrauma occurs with repetitive, cyclic opening and closing of airways and lung units during inspiration and expiration. It is magnified when focal consolidation causes heterogeneous ventilation because more alveoli are exposed to the injurious impact of neighboring alveoli that are opening and collapsing. The stretching or shear forces between aerated and atelectatic lung are greater than those in other lung regions. The relevance of intermittent atelectasis has been questioned because alveolar edema, rather than collapse, seems to dominate the pulmonary microenvironment in many conditions. Recent experimental data show that air fluid levels and surfactant dysfunction cause epithelial shear and cell damage in the conducting small airways.
Barotrauma, which grammatically should reflect ventilation with high pressures, actually results from ventilating lungs using high volumes. This process can lead to alveolar rupture, air leaks, or even pneumothoraces, pneumomediastinum, and subcutaneous emphysema. Despite the misnomer, the critical component in barotrauma is regional overdistension and not high airway pressure. Some experts have suggested the use of the term volutrauma , which implicates excessive volume, with alveolar lung stretching, and not airway pressure, as the determinant of injury.
Biotrauma results from the physical forces of atelectrauma, barotrauma, and volutrauma that cause the release of intracellular mediators. Cells are either directly injured by these mediators or indirectly injured through the activation of cell-signaling pathways in epithelial, endothelial, or inflammatory cells. The translocation of these mediators or bacteria from the airspaces into the systemic circulation through areas of increased alveolar-capillary permeability, as is classically seen in ARDS or as a result of volutrauma, has been touted as a contributor to multiorgan dysfunction and death.
How Do Ventilators Damage the Lungs in Patients?
Although a clear entity, the exact mechanisms of VILI in patients, and the appropriate treatment in each individual, remain unknown. Although VILI is typically considered an independent form of secondary injury, damage by the ventilator is probably an essential contributor to the natural history and evolution of ARDS. The vast majority of research in the field of optimal ventilator management has focused, with limited success, on developing new ways to treat well-established ARDS; however, relatively little attention has been paid to understanding the progression of injury, particularly in patients who do not initially have ARDS.
Moderate tidal volume (V T ; i.e., 12 mL/kg) worsens survival in patients with preexisting ARDS. According to the baby lung construct, inspired gas is concentrated in a smaller, yet otherwise functional, fraction of ventilated parenchyma (i.e., lung capacity), causing overstretching. In an effort to reduce inspiratory strain, clinicians prescribe V T according to patient weight, but there are two corollaries to the baby lung model that have relevant implications on clinical management.
First, the volume and the strain of the ventilated lung are variable and reflect the severity of injury. Indeed, patients with small lung capacity may undergo unacceptable stretch even with a very low V T . Because it is hard to quantify overdistension and strain at the bedside (inspiratory airway pressures are notoriously inaccurate and a safe threshold is unknown), it is difficult to identify those patients at increased risk of VILI who might benefit from an even greater reduction in V T .
Second, it remains unclear how ventilation affects patients without ARDS. Moderate V T does not injure healthy lungs : Clinically acceptable V T sizes may not cause enough stress to worsen injury if the lung capacity is not significantly reduced. However, observational studies and interventional trials, including some conducted on intraoperative patients, have suggested that V T reduction may improve pulmonary outcomes in patients without ARDS. Animal studies have shown that the presence of systemic inflammation, as in sepsis, may raise the sensitivity to mechanical stress in lung tissue; that is, less deformation is required to cause injury. Whether this particular mechanism is relevant to humans is unknown, but it is debatable whether it explains the beneficial effects of low V T in patients without a severe inflammatory process. Because the key mechanism inciting VILI in the absence of severe lung injury is undefined, generalized application of low V T in heterogeneous populations of ventilated patients may not necessarily yield the desired beneficial effects.
The role of atelectasis in ventilated humans is also unclear. Despite compelling evidence from animal experiments on atelectrauma, clinical trials have shown only marginal effects of lung recruitment and high positive end expiratory pressure (PEEP) in established ARDS. This discrepancy could be due to ineffective study recruitment. Indeed, studies have shown that dynamic responses to recruitment and PEEP as measured with radiological and functional instruments better predict ARDS outcomes than one-time measurements of hypoxemia. A key characteristic of ARDS is inflammatory changes that are heterogeneously distributed throughout the lung parenchyma, an aspect ignored by consensus definitions of ARDS and in most study enrollment criteria. This spatial variation may explain the inconsistent findings in many human trials. Although a specific treatment may very well improve one area of lung, it may simultaneously worsen another.
Atelectasis may also generate VILI by increasing susceptibility to V T dimensions. According to accepted paradigms of VILI, atelectrauma occurs in injured, collapsible lung regions, but recent evidence has shown limited inflammatory activation when the effects of atelectasis are separated from those of high inspiratory stretch. However, atelectasis might modify the intrapulmonary distribution of mechanical stress and consequently of inflammation. Said differently, poorly recruited atelectasis may render lungs more vulnerable to moderate V T by reciprocally increasing stretch in ventilated airspaces. Mechanical stress would then be focally amplified in areas of the lung that are adjacent or interspersed with collapsed tissue. A recent study showed a correlation between localized aeration heterogeneity as seen on computed tomography scan (a possible marker of elevated local stretch) and outcomes in ARDS. Recruitment may decrease the amount of stretch the lungs receive from a given V T , thereby limiting the injury.
Atelectasis could contribute to generating injury in non-ARDS patients. A controlled study in ventilated intensive care unit (ICU) patients without ARDS showed improved pulmonary outcomes with the use of high and low PEEP. Furthermore, two successful trials on perioperative lung protection compared low V T in combination with aggressive lung recruitment in the treatment group to higher V T and low PEEP in the controls. Although this approach was designed to determine whether adequate PEEP could prevent the atelectasis associated with low V T , avoiding atelectasis, and not PEEP per se, could be the determinant of success. Because of the design of these trials, it is not possible to separate the contributions of the two interventions. Counter to the hypothesis that atelectasis causes new injury in healthy lungs, a trial of higher and lower intraoperative PEEP—with the same low V T in both arms of the study—failed to demonstrate benefit with higher PEEP.
How to Minimize Lung Damage?
The ARMA trial established improved survival with the use of 6 rather than 12 mL/kg V T . As a result of this study and prior work by other authors, ventilation with low V T (i.e., low-stretch ventilation) is currently the accepted standard of care in established ARDS. Earlier studies, with less dramatic differences in V T between the experimental and control groups, failed to improve outcome. However, a recent observational study confirmed a dose–response relationship between V T and ARDS mortality. This study also showed that the effects of V T selection during the ICU course extend over the long term.
The current clinical approach is to set the ventilator index V T to predicted body weight (PBW), based on height, as a surrogate of lung size. T V is set to 4 to 6 mL/kg IBW while also ensuring that the plateau pressure is less than 30 cm H 2 O and high Paco 2 (partial pressure of carbon dioxide in arterial blood) levels are tolerated. PEEP is adjusted according to ARDSnet nomograms to maintain a goal Paco 2 (partial pressure of oxygen in arterial blood) of 55 to 80 mm Hg and a SpO 2 (O 2 saturation by pulse oximetry) of 88% to 95%. Hypercapnia is limited by acceptable pH, and the effectiveness of metabolic correction remains unclear. Although this strategy decreases mortality, it represents a “one size fits all” model that does not account for individual patient characteristics, such as lung capacity, recruitable atelectasis, and chest wall mechanics. As a consequence, weight-based V T settings may leave a proportion of severely ill patients underprotected, whereas others may be treated with unnecessary aggressiveness (i.e., obese patients with low chest wall compliance). It is not clear how to personalize treatment. Although bedside measurements of lung capacity and strain remain experimental, titration to transpulmonary pressure measured via esophageal manometry seems to be a promising approach. This methodology allows the clinician to correct airway pressure for the effect of chest wall mechanics and to personalize both PEEP and V T settings to minimize peak pulmonary distension while maintaining recruitment.
For patients with the highest severity illness, it is possible that even the most aggressive V T reduction does not achieve sufficient lung protection. The use of extracorporeal lung assist methods has been implemented in select situations, but its generalized use is supported only by one randomized study with important design limitations. In addition, the risk/benefit assessment for this approach remains difficult and poorly understood. High-frequency oscillatory ventilation, an appealing technique for extreme V T reduction and aggressive recruitment, has not been shown to improve outcomes.
On the basis of the existing evidence, ventilator management targeted to recruitment with high PEEP (“open lung ventilation”) cannot be recommended as the standard of care. However, trials have showed some benefit in patients with the highest severity of illness. Expert sources, including society guidelines, recommend the use of high PEEP in this subset of patients. Future studies should use better instruments to stratify patients and monitor effectiveness. Techniques including esophageal manometry or bedside imaging tools such as electrical impedance tomography may provide more support for this approach. Absent these data, the hypothetical benefits of the open lung approach in each individual patient should be weighed against tangible adverse effects. Similar caution should be applied to alternative ventilator modalities that augment mean airway pressures with the goal of maximizing recruitment. Careful patient selection and monitoring of the effects of higher airway pressure on lung overdistension, pulmonary and systemic circulation, and alveolar dead space should be used to avoid undesired responses. Worsening hemodynamics in the face of the unclear biological effects of recruitment are considered by many clinicians to be unacceptable.
Recent trials have suggested that the outcomes of early severe ARDS can be improved with the early adoption of a course of muscle relaxation. A recent study suggested that by maintaining tight control of inspired strain in the early stage of severe ARDS, muscle paralysis optimizes protection from VILI. However, the design of this particular study did not clarify the effect of patient inspiratory effort.
Other usable complementary strategies of lung protection include prone positioning, which in a recent study showed dramatic positive effects on outcomes of severe ARDS. Prone positioning has been studied for many years because of its ability to improve oxygenation in a large proportion of patients. Placing patients in a prone position reopens collapsed dorsal lung regions and increases the homogeneity of the distribution of ventilation, thereby redistributing and attenuating regional inspiratory stress. This effect likely attenuates VILI and could explain observed outcomes. In one particular study, prone positioning was used for longer periods of time and in more severe patients than in previous studies that had more ambiguous results. Therefore prone positioning can be considered as an adjunct to stretch limitation and, possibly, an alternative to PEEP to improve recruitment and minimize regional stress.
Approximately 67% of ARDS cases arise after hospital admission, which provides ample opportunity for preventive interventions. Clinical studies have reported a variable incidence of ARDS (8% to 25%) in patients who undergo mechanical ventilation for other reasons. Thus, it is likely that ventilator management has a role in generating new lung injury and in worsening preexisting pulmonary lesions. However, predicting which patients are at risk for having lung injury and ARDS, as well as the potential severity of illness, remains challenging. Scoring systems have been developed to identify patients at risk, but their application has been inconsistent, and their ability to connect specific treatments with disease prevention is unconfirmed.
A recent meta-analysis showed beneficial outcome effects from the use of lower and higher V T in heterogeneous populations of non-ARDS patients in the ICU and in the operating room. On the basis of this evidence, the use of low V T seems logical in ventilated patients at risk of progression to ARDS, including victims of sepsis or trauma or those undergoing high-risk surgical procedures. However, a word of caution is warranted. Many studies in patients without ARDS have shown outcome differences when comparing control and treatment V T values that greatly differed. Whether smaller differences in V T would have produced similar results is unclear, but many practitioners have already abandoned large V T (i.e., >10 mL/kg) in non-ARDS patients. A recent meta-analysis that included intraoperative ventilation studies showed that, relative to standard management, use of lower V T decreased the onset of ARDS but did not demonstrate any effects on survival or ICU length of stay.
Use of surrogate outcomes such as onset of ARDS to gauge the effectiveness of preventive strategies does not guarantee success in improving death or long-term functional impairment. The success of low V T ventilation in a population at high risk for mortality due to ARDS does not necessarily translate into benefits in patients who are at much lower risk, such as those ventilated in the operating room. Conversely, undesired responses, often undetected by underpowered clinical trials, may affect more patients than the beneficial ones. For example, decreasing V T in a patient without ARDS may worsen atelectasis and lead to increased lung damage and hypoxemia. Although studies to date have not reported an increased requirement for sedation when higher V T are used, sedation practices and goals vary greatly from center to center. If minimal sedation and spontaneous breathing modes, as opposed to deeper sedation and controlled ventilation, are the usual practice, then a reduction in V T may be most relevant to patient discomfort, ventilator asynchrony, and eventually sedation usage, with all of the resultant sequelae.
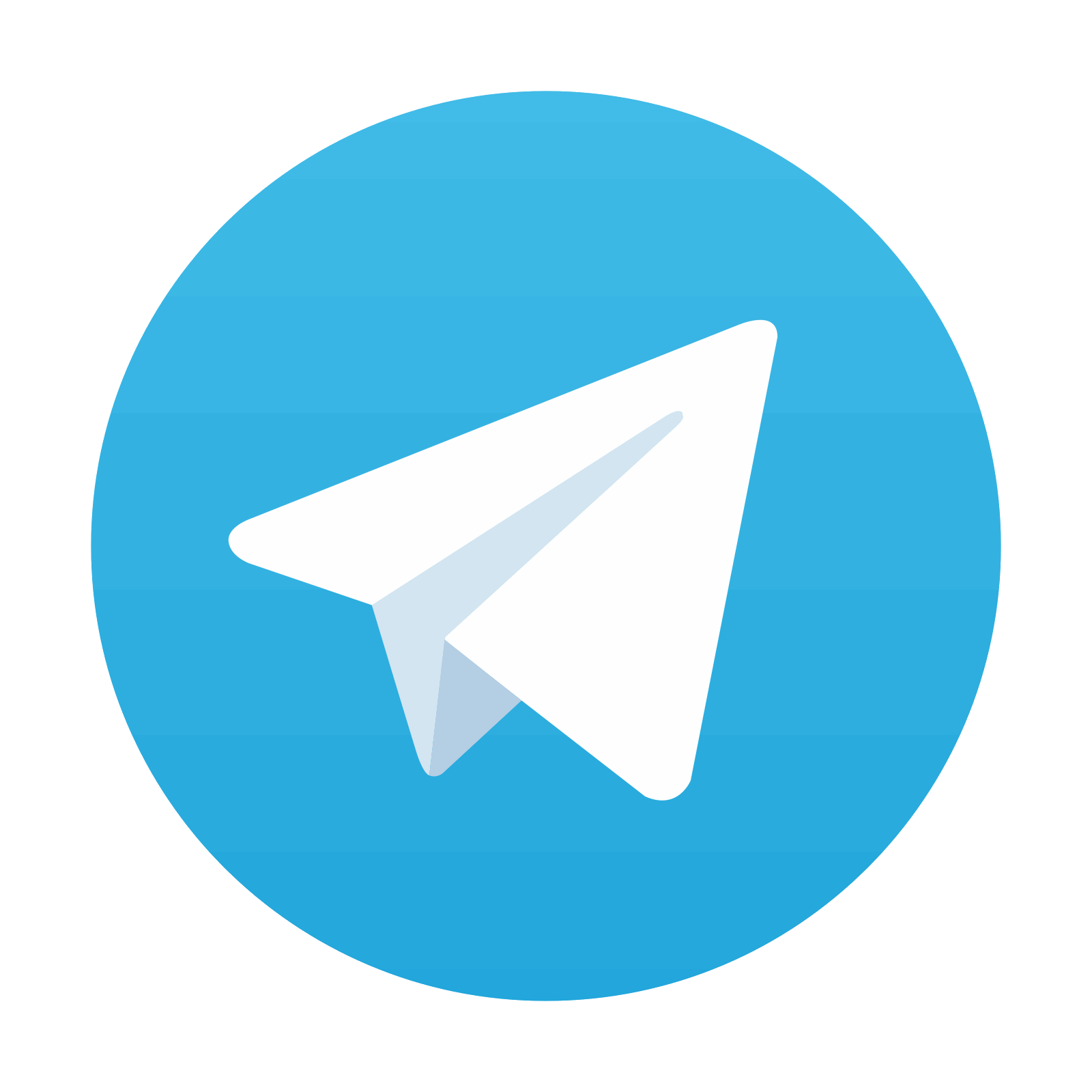
Stay updated, free articles. Join our Telegram channel

Full access? Get Clinical Tree
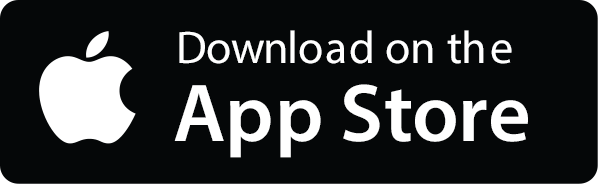
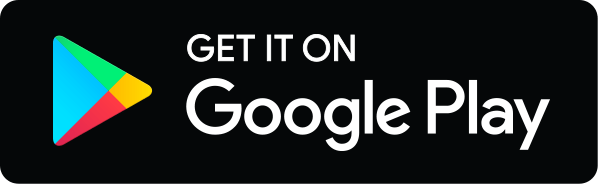
