The ability to evaluate and manage a critically ill patient is one of the most important skills any intensivist brings to the bedside. Patients already resident in the intensive care unit (ICU) can decompensate because of any of several causes; thus, the differential diagnosis is broader than it is in less complex patients. Appropriate management is dependent on correct identification of the cause or causes of decompensation. The classes of shock are cardiogenic, hypovolemic (e.g., hemorrhagic), vasodilatory (e.g., septic, adrenal insufficiency, anaphylaxis), or obstructive (e.g., tension pneumothorax, cardiac tamponade).
The goal of evaluating circulation is ultimately to determine whether the patient has adequate end-organ perfusion. Clinically, mental status and urine output are widely accepted as the most reliable indicators of adequate end-organ perfusion, but they may not be readily assessed in a substantial percentage of critically ill patients. Signs of hypoperfusion may include tachycardia, tachypnea, low mean blood pressure, and low urine output. Encephalopathy, a marker for cerebral perfusion, is also a predictor for higher mortality when associated with hemodynamic instability.
Targets of Treatment
Mean arterial blood pressure is a primary indicator of hemodynamic instability. End-organ perfusion is maintained over a wide range of mean arterial pressures (MAPs) because of autoregulation. In patients with chronic hypertension, the autoregulation curve is shifted to the right, indicating that these patients require higher MAPs to achieve adequate end-organ perfusion. Because of its close association with sufficient perfusion, MAP goals become a target for the initial resuscitation efforts in shock. Recent literature suggests that a MAP of 65 mm Hg is sufficient for patients with septic shock and is generally associated with good outcomes in critically ill patients.
Mixed venous or central venous oxygen saturation or content can be reliably used to make inferences about the balance of oxygen delivery to the peripheral tissues and oxygen uptake by them. Mixed venous oxygen saturation (S v O 2 ) is measured from the pulmonary arterial port of a pulmonary artery catheter. For patients without pulmonary artery access, central venous oxygen saturation (S cv O 2 ) is often used as an alternative. ScvO 2 is typically measured from a central line placed within the superior vena cava. Multiple studies have revealed variable correlation between S v O 2 and S cv O 2 . However, Chawla et al. found that S cv O 2 drawn from a port within the right atrium had good correlation with S v O 2 , although there was a bias toward S cv O 2 being 5.2% higher than the S v O 2 . This difference can be explained by the addition of blood from the coronary sinus, which drains the high oxygen extraction system of the heart, into the measurement of S v O 2 but not the S cv O 2 . Multiple studies focused in goal-directed resuscitation have used S cv O 2 to determine if fluid administration is sufficient. A S cv O 2 greater than 70% is common in resuscitation algorithms and may improve outcomes.
Is This Hypovolemia? Is the Patient Volume Responsive?
Hypovolemic shock, when recognized, is readily treatable, which makes recognition imperative. Because only approximately 50% of patients in shock will respond to a volume challenge, it is important to determine which patients will benefit from volume infusion. Hemodynamic parameters may be used to predict fluid responsiveness. There is essentially no evidence that traditional measures, such as central venous pressure (CVP) and pulmonary artery occlusion pressure predict volume responsiveness.
Systolic Pressure Variation and Pulse Pressure Variation
Dynamic parameters, namely systolic pressure variation (SPV) and pulse pressure variation (PPV), are superior to all alternatives in making inference about volume responsiveness. These methods assess the variation in the blood pressure components caused by cyclic increases in intrathoracic pressure by positive pressure mechanic ventilation. Increased intrathoracic pressure will cause increase in stroke volume as well as systolic and pulse pressures early in mechanic inspiration secondary to a transient increase in left ventricular (LV) end diastolic volume, decrease in afterload, and decrease in right ventricular (RV) volume. This increase in systolic pressure is termed delta up (dUp). Immediately after, the sustained intrathoracic pressure decreases RV preload, and increased transpulmonary pressures during this phase of mechanic inspiration result in elevated RV afterload. The combination of reduction in RV preload and increase in RV afterload leads to decreased RV stroke volume, which leads to decreased LV preload and stroke volume. This decrease in systolic pressure is termed delta down (dDown). SPV is defined as the difference between dUp and dDown within one mechanic breath. dDown has independently been shown to predict whether a patient will be volume responsive.
There are disadvantages to the use of SPV and PPV. The patient must be in sinus rhythm to interpret the dynamic data. Although PPV and LV stroke volume are tightly coupled in mechanically ventilated patients, PPV has been regarded as less reliable in spontaneously breathing patients. Recent literature suggests that PPV and SPV may also be of value in predicting volume responsiveness in spontaneously breathing patients and are likely superior to all other techniques. Hong et al. studied a group of nonintubated adult patients undergoing elective thoracic surgery. The patients were instructed to breathe in a pattern of forced inspiration followed by slow, passive expiration. The authors found that forced inspiration during spontaneous breathing can predict fluid responsiveness with a PPV threshold of 13.7% with an area under the curve of 0.910 (95% confidence interval 0.806–0.969, P < 0.0001). In comparison, PPV in tidal breathing failed to accurately predict volume responsiveness in these patients.
Inferior Vena Cava Collapsibility on Ultrasound
Bedside ultrasound of the inferior vena cava (IVC) is an alternative means for assessing volume responsiveness. The advantages of bedside ultrasound include its noninvasive nature and easy availability. Measurement of the dynamic change in the diameter of the IVC with the respiratory cycle has been shown to be a means to determining whether a patient will respond to a fluid challenge. Because the IVC does not have the same compensatory vasoconstriction to volume loss, it may better reflect a patient’s volume status than arterial measures such as blood pressure, pulse rate, and aortic diameter. Although the performance of IVC ultrasound is better in mechanically ventilated patients than in spontaneously breathing patients, its performance in either context is adequate (sensitivity 0.81 vs. 0.7 and specificity 0.87 vs. 0.85, respectively). Similar to the PPV/SPV literature, it is likely that uncontrolled spontaneous ventilation may result in variable and inconsistent changes in intrathoracic pressure, leading to less consistent changes in IVC diameter. In a meta-analysis of spontaneously breathing patients, Dipti et al. found that the maximal IVC diameter during exhalation was significantly lower in those who were hypovolemic as compared with those who were determined to be euvolemic.
Passive Leg Raise
Passive leg raise predicts the volume responsiveness of patients with shock. For this maneuver to be performed, the patient is placed in the supine position and bilateral lower extremities are raised to a 45-degree angle. Passive leg raise is correlated with increases in pulmonary artery occlusion pressure and end-diastolic dimension. Monnet et al. revealed passive leg raise to be the equivalent of a 500-cc fluid challenge. Importantly, passive leg raise is effective in determining fluid responsiveness in cardiac arrhythmias and in patients who are spontaneously breathing. An algorithm for assessing volume responsiveness appears in Figure 15-1 .

In recent years, a series of studies have been published that have measured the mean systemic pressure in hypotensive patients, and they have shown that it reliably predicts volume responsiveness. If these observations are replicated and instruments that can be used to measure this become available in clinic practice, then it is possible that determination of the mean systemic pressure may become the gold standard for making inferences about volume status and volume responsiveness.
Treating Hypovolemic Shock
There is no consensus on what fluids should be used to treat hypovolemic shock, although there is growing consensus that lower-sodium crystalloid solutions are superior to normal saline. The surviving sepsis guidelines recommend initial resuscitation with crystalloid because it is less expensive than albumin. More recent analysis suggests that resuscitation with albumin may have some outcome benefit in sepsis, particularly in its most severe form (Albios Study, supplementary material). Hydroxyethyl starches should be avoided because of a link to increased need for renal replacement therapy and mortality when used in septic shock. Although many experts continue to recommend volume resuscitation to a CVP endpoint, clinic endpoints (mental status, urine volume), MAP, lactate levels, and mixed/central venous saturations are likely more valid endpoints.
Is this Septic Shock?
Septic shock is caused by infection/inflammation and manifests as decreased vascular tone, resulting in hypotension and redistribution of blood flow. Surviving sepsis guidelines recommend a protocol driven by early resuscitation at the earliest sign of hypoperfusion. Because elevated lactate is an independent marker of morbidity and mortality, experts also recommend normalization of lactate levels as a marker of normalization of tissue perfusion. Jansen et al. incorporated a normalization of lactate algorithm for the management of patients with sepsis. Jansen’s study, protocol-driven normalization of lactate, resulted in a significant reduction in ICU length of stay and ICU and hospital mortality in the treatment group.
Prompt administration of appropriate antibiotics after the diagnosis of septic shock is imperative to improving outcomes. Broad-spectrum empiric antibiotics should be given within the first hour of recognition of sepsis or septic shock. Every hour of delay in administration of antibiotics increases mortality in septic shock. Empirically administered antibiotics should provide coverage for infection with gram-positive, gram-negative, and anaerobic bacteria, and it may include fungal and viral coverage in some patients. Source control with diagnosis of a specific infectious source and subsequent intervention should be performed.
Septic patients who have been adequately volume resuscitated may require therapy with vasoactive drugs to achieve adequate blood pressure and vital organ perfusion. The goal of the addition of vasopressors (e.g., norepinephrine) is not solely to increase MAP to a target of 65 mm Hg but also to obtain acceptable end-organ perfusion.
Norepinephrine and vasopressin can be used to support the circulation of septic patients. The VASST (Vasopressin and Septic Shock Trial) demonstrated a survival benefit in a subgroup of patients requiring less than 15 μg/min of norepinephrine with the addition of vasopressin. If end-organ perfusion remains insufficient despite sufficient volume resuscitation and adequate MAP, then inotropes (i.e., dobutamine) may be added to increase cardiac output.
Is this Obstructive Shock?
The evaluation of a patient for cardiogenic or obstructive shock requires the concurrent or sequential completion of several diagnostic evaluations in short order. These include the inspection of ventilator flow waveforms for the presence of auto-positive end-expiratory pressure (PEEP), chest radiography or ultrasonography to assess for the presence of tension pneumothorax, measurement of bladder pressures in patients with a tense or distended abdomen to evaluate for abdominal compartment syndrome, and finally the performance of echocardiography (e.g., FOCUS [focused cardiac ultrasound] examination or FATE [focused assessed transthoracic echocardiography]) to evaluate the patient for cardiogenic shock and tamponade.
Abdominal Compartment Syndrome
Cardiogenic and obstructive shock are important causes of hypotension, and patients who have neither hypovolemic nor septic shock will need to be evaluated for these. Obstructive shock can be pericardial, thoracic, or abdominal in origin. Intra-abdominal hypertension is the result of diminished abdominal wall compliance, increased intraluminal contents, increased intra-abdominal contents, or capillary leak/fluid resuscitation. Intra-abdominal hypertension progresses to abdominal compartment syndrome when the compartment pressure is greater than 25 mm Hg, at which point the transmitted pressure affects cardiac, pulmonary, and renal functions as well as cerebrospinal pressure. Patients in the intensive care setting have multiple risk factors for the development of intra-abdominal hypertension, including abdominal surgery, trauma, burns, ileus, acute pancreatitis, intra-abdominal infections/abscesses, intraperitoneal fluid (ascites, hemoperitoneum), sepsis, acidosis, and massive fluid resuscitation. High peak airway pressures on the ventilator can be a trigger to consider increased abdominal pressures. At present, assessment of bladder pressures is the gold standard of measurement for abdominal compartment syndrome. If abdominal compartment syndrome is suspected, then the World Society for Abdominal Compartment Syndrome recommends various medical and surgical interventions that include decompressive laparotomy.
Auto-PEEP
Additional causes of obstructive shock arise from the thorax. Auto-PEEP is the process by which the respiratory system is unable to return to functional residual capacity at the end of the expiratory phase of ventilation. Auto-PEEP impairs venous return to the heart, increases risk of barotrauma to the lungs, and can increase the patient’s work of breathing while impeding their ability to trigger the ventilator. Short respiratory cycles, high minute volumes, and obstructive lung disease predispose a patient to auto-PEEP. It is diagnosed on ventilator waveforms by persistence of flow at end expiration. Management of auto-PEEP includes decreasing respiratory rate and/or decreasing the I:E ratio to allow for more expiratory time.
Tension Pneumothorax
Tension pneumothorax occurs when air is able to enter the chest via a one-way valve type of mechanism causing increased intrapleural pressure. As intrathoracic pressure increases, there is mediastinal shift, which results in ventilatory compromise, compression of the vena cava at both the thoracic inlet in the neck and at the diaphragm leading to decreased venous return to the heart, and direct compression of the heart. In the hands of experienced intensivists, thoracic ultrasound is at least comparable to and likely superior to the gold standard of chest radiography in diagnosing pneumothorax. Management includes needle thoracostomy followed by chest tube placement.
Cardiac Tamponade
Pericardial effusion can produce cardiac tamponade physiology by compressing cardiac chambers (especially right-sided structures), causing decreased LV preload and decreased cardiac output. In some instances (e.g., patients with a right ventricular assist device [RVAD]), mediastinal fluid can compress the great veins of the thorax and obstruct venous return without directly impinging on the pump itself. Pulsus paradoxus and electric alternans accompanying tachycardia and hypotension can often signal the presence of tamponade physiology. Bedside echocardiography can reveal pericardial effusion and collapse of right-sided cardiac chambers. Prompt needle decompression with or without ultrasound guidance is the definitive treatment of hemodynamically significant pericardial effusions.
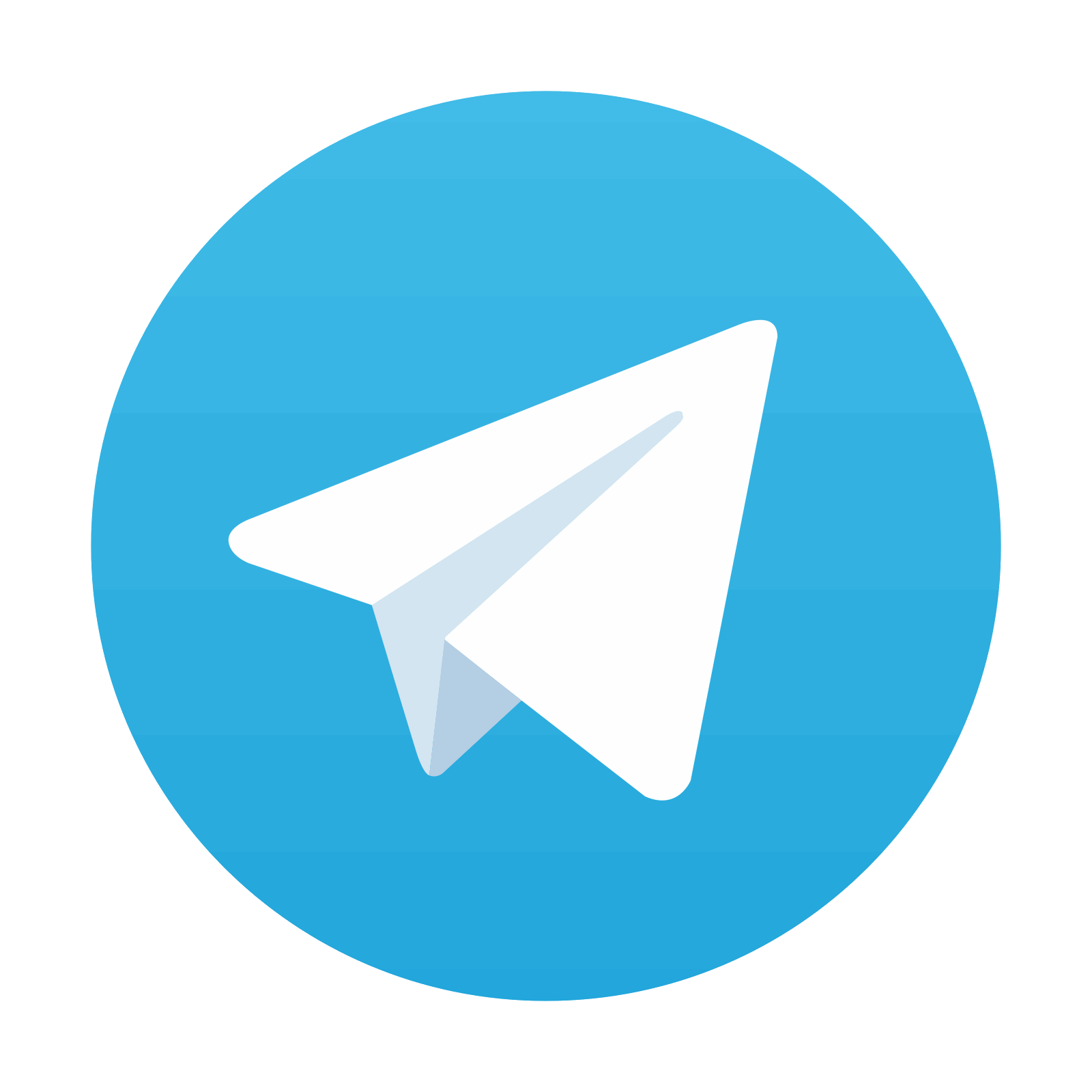
Stay updated, free articles. Join our Telegram channel

Full access? Get Clinical Tree
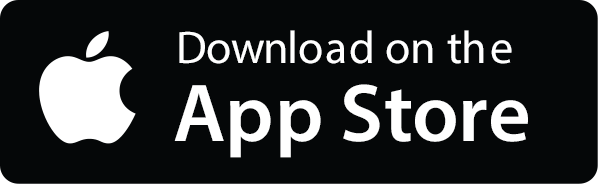
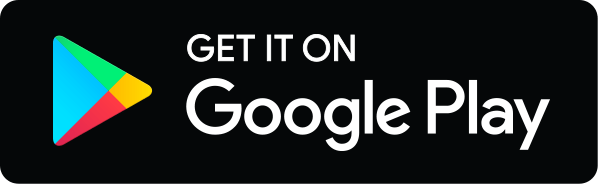