How Can We Monitor the Microcirculation in Sepsis?
Altered Microcirculation in Sepsis
Sepsis is a clinical condition associated with high morbidity and mortality worldwide, and its management represents a challenge for the clinician in the intensive care unit (ICU). Septic shock is usually characterized by severe hemodynamic alterations. From a macrohemodynamic point of view, it is defined by a decrease in vascular tone with some degree of hypovolemia, with or without concomitant myocardial depression. Of note, even when these global hemodynamic parameters seem to be corrected, signs of tissue hypoperfusion may still persist. Evidence suggests that microcirculatory dysfunction is a fundamental pathologic feature of sepsis. Although until recently examination of the microcirculation has been hampered by technologic limitations, development of microcirculatory evaluation techniques has allowed direct study of this phenomenon. Microcirculatory alterations may produce tissue hypoxia by induction of oxygen supply–demand imbalance at the cellular level. Maintained over time, this situation can lead to cellular and organ dysfunction and ultimately death.
The microcirculation is the final destination of the structures and mechanisms responsible for delivering oxygen to the tissue cells and thus is essential for maintaining adequate organ function. It consists of a complex network of small blood vessels (<100 μm diameter) composed of arterioles, capillaries, and venules. Arterioles are responsible for maintaining vascular tone and are lined by smooth muscle cells. They respond to extrinsic and intrinsic stimuli to match oxygen delivery with local metabolic demand. Capillaries are the primary site of exchange for oxygen and metabolic waste, oxygen diffuses passively along its concentration gradient to the respiring tissue cells, and waste converges on and is taken up by the venules. Far from being just a vessel network, the microcirculation is a complex system that also involves interaction between the different cell types and their subcellular structures to achieve various physiologic functions. These include not just oxygen transport but also hemostasis, hormonal transport, and host defense. All these elements can interact with each other and are regulated by different complex mechanisms controlling microcirculatory perfusion.
Recently, multiple experimental and clinical studies have reported microcirculatory alterations in severe sepsis and septic shock. These studies observed a decrease in capillary density that likely reflects an alteration in microcirculatory autoregulation. The net effect is an increase in the diffusion distance of oxygen to tissues. Moreover, studies reveal changes in the heterogeneity of microcirculatory perfusion. As a consequence, the number of under- or unperfused capillaries in proximity to well perfused capillaries is increased. This change leads to functionally vulnerable microcirculatory units. Conventional systemic hemodynamic- and oxygen-derived variables may fail to detect this dysfunctional microcirculatory condition. Thus the key hemodynamic deficit in sepsis may well be microcirculatory shunting that results in an oxygen extraction deficit, an alteration that may be a potential target for resuscitation. From this point of view, microcirculatory shunting is considered to play a leading role in the pathophysiology of sepsis and multiorgan failure.
According to the previously mentioned and current evidence, bedside evaluation of microcirculation may be useful in management of severe sepsis and septic shock patients.
Current Methods to Monitor the Microcirculation in Patients with Sepsis
Evaluation of the microcirculation in critically ill patients presents certain methodological and technical difficulties that have retarded its use at the bedside. By definition, any technique for evaluating the microcirculation can monitor only the tissue bed to which it is applied. Therefore it is necessary to select sites that are easily accessible but that are also representative of the rest of the body. Nevertheless, it is important to understand that the microcirculatory alterations observed in a selected tissue area are a window that is likely to reflect the microcirculation in other areas, provided that there are no local interfering factors.
Current techniques to monitor the microcirculation can be divided into two main groups:
- 1.
Indirect methods to monitor function through evaluation of regional tissue oxygenation.
- 2.
Direct methods to monitor perfusion that allow direct visualization of the microvascular network and of microcirculatory blood flow.
Indirect Methods to Assess Microcirculation: Evaluation of Tissue Oxygenation
Indirect methods based on measures of tissue oxygenation, as surrogates of microcirculatory perfusion, include gastric tonometry, sublingual capnometry, tissue oxygen electrodes, and near-infrared spectroscopy (NIRS). Among these technologies, NIRS has aroused increasing interest in the evaluation of the regional circulation because of its noninvasive nature and easy applicability.
Near-Infrared Spectroscopy
NIRS measures the attenuation of light in the near-infrared spectrum (700 to 1000 nm) to measure chromophores, mainly hemoglobin, present in the sampled tissue. Choosing specific scan lengths minimizes the impact of other tissue chromophores on the NIRS signal. Thus the final signal is derived primarily from oxyhemoglobin and deoxyhemoglobin contained in the microvascular tree (vessels <100 μm) present in the sampled area. Measuring oxy- and deoxyhemoglobin permits calculation of the overall saturation of tissue hemoglobin or tissue oxygen saturation (StO 2 ). The NIRS system consists of a light source, optical bundles (optodes) for light emission and reception, a processor, and a display system.
Although StO 2 has been evaluated in several organs, skeletal muscle StO 2 , which is nonvital and peripheral, may be the optimal early detector of occult hypoperfusion. Because StO 2 measurements can be altered by local factors such as edema and fat thickness, the thenar eminence has been proposed as a reliable site for measurements. In healthy patients under basal conditions, the NIRS signal predominantly reflects the venous oxygenation because an estimated 75% of the blood present in the skeletal muscle is located in the venous compartment. Thus, StO 2 is similar to mixed venous oxygen saturation and reflects the balance between local oxygen supply and consumption. Thus changes in StO 2 can be altered by both changes in local microcirculatory flow and changes in local consumption.
In addition to monitoring the absolute value in the thenar eminence, the StO 2 response to a brief ischemic challenge can provide dynamic information on tissue performance. In the so-called vascular occlusion test (VOT) an artery proximal to the StO 2 probe is occluded until a given ischemic threshold is reached, and the occlusion is then released. This test generates some dynamic parameters: the initial deoxyhemoglobin slope (DeO 2 ) following ischemia has been proposed as a marker of local oxygen extraction. When the DeO 2 is corrected for the estimated amount of hemoglobin, the result is a parameter of local oxygen consumption, the nirV o 2 . The reoxygenation slope (ReO 2 ) that follows the release of the vascular occlusion has been proposed as a marker of endothelial function because it depends on blood inflow and capillary recruitment after the hypoxic stimulus. However, several studies also correlated ReO 2 with perfusion pressure. Thus, the resulting ReO 2 may be derived from the interaction of perfusion pressure with endothelial integrity.
Although septic patients tend to have lower StO 2 values than healthy subjects, there is a huge overlap between these two populations. These observations may be explained by the heterogeneity of microcirculatory alterations in sepsis (ischemic and highly oxygenated areas coexist), with an overall “normal” oxygen content in a given sensed area. The low sensitivity of this approach may be a major limitation of absolute StO 2 in sepsis. However, the use of VOT-derived variables appears to be more promising. Several studies have reported alterations in the StO 2 response to the VOT in sepsis, and the magnitude of these alterations correlated directly with prognostic factors and even with mortality.
Direct Methods to Assess Microcirculation: Evaluation of Microvascular Perfusion
Clinical Examination
On the basis of the concept that the peripheral circulation provides an early glimpse into a circulatory disturbance that may lead to shock, some classic clinical findings are used at the bedside as surrogates of the presence of an impaired circulation. This noninvasive peripheral perfusion evaluation includes several easy-to-evaluate bedside measures such as capillary refill time and mottling score and the central-to-toe temperature gradient that may be used to relate peripheral tissue hypoperfusion to the severity of organ dysfunction and outcome, independent of systemic hemodynamics. However, these methods have important limitations: they are difficult to quantify and provide relevant information on the peripheral (particularly skin, an organ with independent mechanisms of regulation) rather than the central microcirculation. Therefore these clinical methods, although useful for identifying patients at risk, have limited applications in daily clinical practice.
Videomicroscopy
Developed more than three decades ago, epi-illumination methods were introduced to observe the microcirculation in vivo without the need for transillumination. This approach eliminated one of the main technical issues that limited clinical utility. These methods were later incorporated into handheld microscopes, eventually giving rise to orthogonal polarization spectral (OPS) imaging developed by Slaaf and co-workers and incident dark-field (IDF) illumination developed by Sherman and co-workers. OPS and later sidestream dark-field (SDF, an application of IDF imaging ) are videomicroscopic imaging techniques based on similar general principles that filter surface reflections of incident illumination light to allow detection of subsurface microcirculatory structures. After a light source is applied on a surface, the light is reflected by the deeper layers of the tissue, transilluminating superficial tissue layers. Accordingly, this technique can be used only on organs or tissue surfaces covered by a thin epithelial layer because the penetration of the green light used is about 0.5 mm. The selected wavelength (530 nm) of illumination light is absorbed by the hemoglobin in the red blood cells irrespective of its oxygen content. Erythrocytes are seen as black-gray bodies flowing inside capillaries (absorbed light) over a white tissue background (reflected light). Thus, only functional capillaries (with red blood cell flow) would be observed in contrast to physiologic nonfunctional capillaries (without red blood cell flow), which would not be detected. Although the main focus of the technique is evaluation of red blood cell flow and the microvessel network, other microcirculatory elements such as leukocytes can also be identified.
In contrast to animal studies or patients undergoing surgery where several internal organs have been explored with videomicroscopy, in critically ill patients, this technique has been applied in more accessible surfaces, especially the sublingual mucosa. The sublingual area has been the most intensely investigated surface. In this region, different sized venules (25 to 50 μm) and capillaries (<25 μm) can be examined, whereas arterioles (50 to 100 μm) are normally not identified because they are located in deeper layers and the optics in early OPS and SDF devices limit visualization.
The early phase of severe sepsis and septic shock is characterized by a significant decrease in vessel density and in the proportion of perfused capillaries in sublingual videomicroscopy studies. In addition, these studies identified an increase in heterogeneity of vascular density and blood velocity between coexisting areas. These alterations were more severe in nonsurvivors, and the rapid resolution of microcirculatory changes after interventional therapy correlated with improved outcome, including mortality. Conversely, the persistence of microcirculatory alterations after the first 24 hours strongly and independently correlated with early mortality secondary to circulatory failure and with the development of multiorgan dysfunction in the late phase.
Quantification of microcirculatory alterations has been a challenge because these techniques are limited by the hardware and because different scoring systems have been developed. After the conclusions of an expert consensus conference, the ideal microcirculation analysis report should evaluate microvascular blood flow, vascular density, and perfusion heterogeneity. Microcirculatory perfusion is evaluated assessing microvascular flow index (MFI) and the proportion of perfused vessels (PPVs). Vascular density is evaluated by assessing total vessel density and perfused vessel density (PVD). Importantly, tissue perfusion is dependent on functional capillary density (reflected by PVD) and blood velocity (reflected by MFI). Vascular density is thought to be more important than blood velocity in ensuring tissue oxygenation because cells are able to regulate oxygen extraction. Accordingly, homogeneous low flow should be better tolerated than heterogeneous flow even when total blood flow is lower. On the other hand, the presence of very high blood flow may theoretically reduce the time needed for hemoglobin to unload oxygen to cells and also may induce capillary endothelial damage by shear stress. Finally, heterogeneity of perfusion is reflected by PPV in the investigated area and the heterogeneity index (Het Index) in the investigated organ. Assessing heterogeneity of perfusion is an essential factor for evaluating the shunt fraction in septic shock. Most of these variables are quantitative; flow-related parameters are semiquantitative but are sensitive enough to evaluate microcirculatory performance.
Routine clinical use of handheld microscopes has been sparse because the current first-generation (OPS) and second-generation (SDF) devices are technically limited and because automatic bedside image analysis is problematic. Thus, these approaches have been used primarily for research purposes. Recently, a third-generation handheld microscope with incident dark-field imaging (Cytocam–IDF imaging ) has become available. A computer-controlled high-resolution, high pixel–density digital camera permits instant analysis and quantification of images. With this advanced technology, physiologically relevant, functional microcirculatory parameters may be measured and directly related to the clinical setting. This development should allow direct implementation of quantitative microcirculatory imaging monitoring at the bedside and thus open the way for its use in clinical decision making such as titrating fluid resuscitation to achieve microcirculatory endpoints.
Overall, videomicroscopy is considered to be the gold standard technique for assessing microcirculation at the bedside. In the near future, this technique may allow monitoring of the last frontier of tissue perfusion in daily clinical practice.
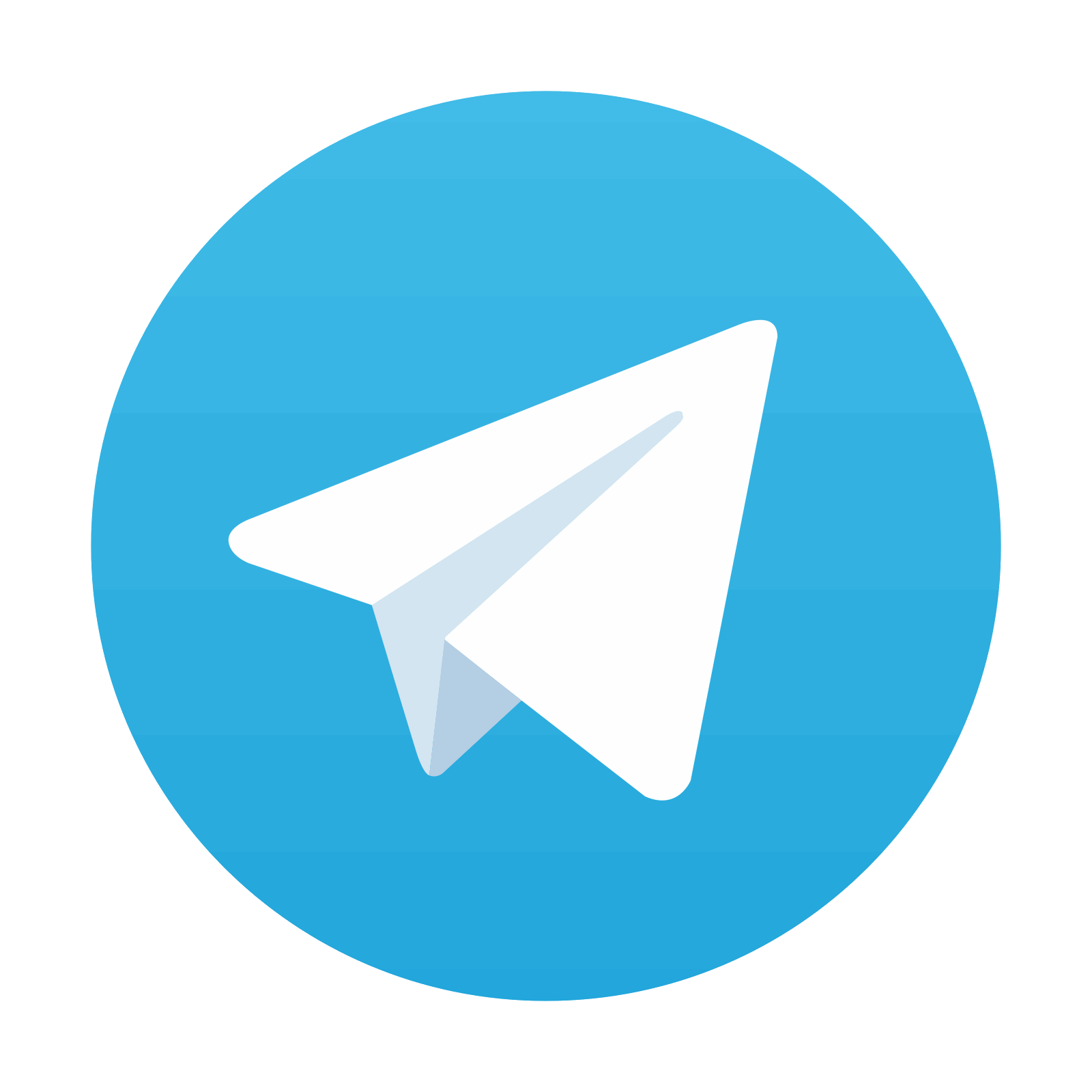
Stay updated, free articles. Join our Telegram channel

Full access? Get Clinical Tree
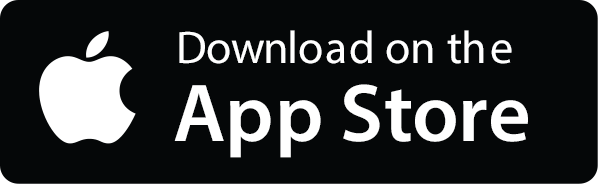
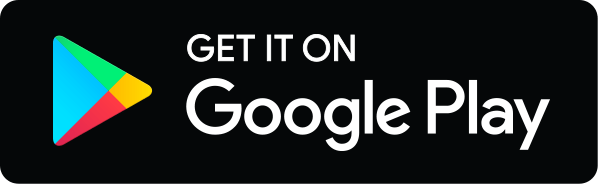