High-altitude illness (HAI) often affects young and otherwise healthy individuals. This disease progresses from acute mountain sickness (AMS) to potentially life-threatening high-altitude pulmonary edema (HAPE) and high-altitude cerebral edema (HACE).
Acclimatization and slow ascent are the best way of avoiding HAI.
Acetazolamide has been shown to be very effective for acclimatization when staging is not possible in individuals who are at an increased risk of HAI.
Truncal ataxia is the cardinal sign of HACE, and immediate definitive treatment is descent. High-flow oxygen is indicated as soon as symptoms are recognized and dexamethasone, at an initial dose of 1 to 2 mg/kg orally or intramuscularly, can produce dramatic improvement.
HAPE, with a cardinal sign of severe dyspnea at rest, is the leading cause of high-altitude death other than trauma.
High-altitude illness (HAI) has been documented for thousands of years, and with the increasing popularity of various recreational activities individuals tend to ascend to greater altitudes, raising the incidence of HAI. Examples of activities that can put individuals at risk include hiking, mountain climbing, biking, skiing, snowboarding, hot air balloons, and gliding. With the ease and access to modern travel, the incidence of HAI can be expected to continue rising, putting more children and adults at increased risk.
HAI results from the decrease in barometric pressure, and the individual’s response to hypoxia and can affect individuals of any age, even young, healthy individuals.1,2 HAI seems to affect children and adults equally.3 However, the diagnosis may be delayed in young children who cannot describe their symptoms.
HAI encompasses a broad spectrum of disease. The continuum ranges from acute mountain sickness (AMS), the mildest form of HAI, to the potentially life-threatening high-altitude cerebral edema (HACE) and high-altitude pulmonary edema (HAPE). Symptoms of HAI may develop within hours or days after ascent. In contrast, hypoxemia occurs within minutes to hours of arrival at altitude and results in the initiation of the cascade of physiologic events that lead to AMS, HAPE, and HACE (Table 141-1).
Acute Mountain Sickness (AMS) | High-Altitude Cerebral Edema (HACE) | High-Altitude Pulmonary Edema (HAPE) | |
---|---|---|---|
Altitude | Rare below 8000 ft Affects nearly everyone who rapidly ascends to 11,000 ft | Rare below 12,000 ft | Rare below 8000 ft More commonly associated with altitudes >14,500 ft |
Onset | Within 4–8 h of a rapid ascent but can be as long as 4 d Peaks within 24–48 h Usually resolves by the third or fourth day | Most often within 1–3 d after ascent to altitude | Usually within 1–4 d after ascent to altitude Most common during the second night at altitude |
Symptoms | Most common: headache, sleep disturbance, fatigue, shortness of breath, dizziness, anorexia, nausea, vomiting, oliguria Other symptoms: mild peripheral edema, weakness, lassitude, malaise, irritability, decreased concentration, poor judgment, palpitations, deep inner chill, dull pain in the posterolateral chest wall | Severe headaches, nausea, vomiting, altered mental status Cardinal sign: truncal ataxia Will proceed to include confusion, slurred speech, diplopia, hallucinations, seizures, impaired judgment, cranial nerve palsies (third and sixth), abnormal reflexes, paresthesias, decreased level of consciousness, coma, death | Initial symptoms: dry cough, fatigue, dyspnea on exertion, few rales Symptoms of AMS may be present As symptoms progress: productive clear cough, orthopnea, weakness, altered mental status, tachycardia, tachypnea, fever, increased rales, cyanosis Cardinal sign: severe dyspnea at rest |
Treatment | Rest Increase fluids No higher ascent until symptoms have resolved Proceed to a lower altitude if no improvement within 1–2 d or if symptoms worsen Supplemental oxygen, if available Symptomatic relief for headache, nausea Immediate descent is indicated for ataxia, decreased level of consciousness, confusion, dyspnea at rest, rales, or cyanosis Acetazolamide: adult, 125 mg bid; pediatric, 2.5 mg/kg bid | Immediate descent High-flow oxygen Dexamethasone 0.15 mg/kg (maximum dose of 4 mg) every 4–6 h Rest Hyperbaric therapy if descent is not possible | Mild-to-moderate HAPE: bed rest, supplemental oxygen Observe closely Severe HAPE: Immediate descent, minimal exertion High-flow oxygen Nifedipine 0.5 mg/kg (maximum 20 mg) q8h Hyperbaric therapy if descent is not possible |
Prevention | Acclimatization: slow, graded ascent; first night’s sleep should be at an altitude no greater than 8000 ft and the first day should be spent at rest; daily climb should then be limited to 1000 ft if the altitude is between 10,000 and 14,000 ft; beyond 14,000 climbers should take 2 d for each 1000-ft ascent. Avoid alcohol, sedatives, smoking Acetazolamide: start 48 h before ascent and continue for at least 48 h after arriving at the highest altitude (same as treatment dosage) |
Acetazolamide or dexamethasone (same as treatment dosage) |
Nifedipine (same as treatment dosage) |
There are a number of factors that influence the incidence, onset, and severity of HAI. Risk factors common to the pediatric and adult populations are rate of ascent, altitude achieved, length of stay, and physical exertion. The severity of HAI varies when any of these factors or a combination of them exceeds the individual’s ability to adapt to the new environment. The highest incidence of AMS occurs between the ages of 1 and 20 years, and the severity of symptoms decreases with increasing age.
Unique anatomical and physiologic differences among infants and children may increase the risk of ventilation–perfusion mismatch resulting in hypoxia.
Contributing factors4 include:
Increased rib cage compliance.
Increased airway reactivity in response to hypoxia.
Fewer alveoli are present in early infancy.
Reduced surfactant in preterm infants.
Reduced upper and lower internal diameters of the airways.
Increased proportion of the pulmonary vascular bed with muscular arterioles in early infancy.
Tendency for a paradoxical inhibition of increased respiratory drive (up to 1–2 months of age) to hypoxia.
Presence of fetal hemoglobin until 4 to 6 months of age.
High altitude is generally considered to be 5000 to 11,500 ft (1500–3500 m), and at this altitude arterial oxygen saturation falls below 90% (PaO2 60 mmHG). Acclimatization is necessary to prevent illness. Although severe altitude illness is uncommon below 8000 ft, individuals with pre-existing medical conditions may become symptomatic with abrupt ascent. Very high altitude (11,500–18,000 ft; 3500–5500 m) is the most common range for altitude illness and at this range arterial oxygen falls between 75% to 85%. At extreme altitude, generally greater than 18,000 ft (5500 m) acclimatization is not possible and altitude illness is inevitable.
An understanding of the physical gas laws along with the composition of the atmosphere explains the occurrence of HAI. The atmosphere is comprised of multiple gases. The largest percentage is nitrogen (78%), followed by oxygen (21%). Forces exerted at any given altitude are represented as barometric pressure or atmospheric pressure. At different altitudes, while the percentage of each gas remains the same, the partial pressures of the gases will vary.
Boyle’s law states that the volume of a given mass of gas will vary inversely with its pressure. As an individual ascends, barometric pressure decreases and the volume of gas within an enclosed space expands. As the individual descends, the reverse is true.
Dalton’s law of partial pressure describes the pressure exerted by gases at various altitudes. The total pressure of a mixture of gases is the sum of the partial pressures of all the gases in the mixture. At sea level, barometric pressure is 760 mmHG, and the percentage of oxygen in the atmosphere is equal to 20.95%. Therefore, the partial pressure of oxygen (PO2) at sea level is 159.22 mmHG.
As altitude increases and pressure decreases, gas expansion causes the available oxygen to decrease. For example, at 10,000 ft, where the barometric pressure is 523 mmHG, the percentage of oxygen remains 20.95% but the partial pressure of oxygen will decrease to approximately 110 mmHG and the alveolar PO2 will drop to 60 mmHG.
Several different physiologic responses are seen at high altitude. The response to hypoxia is the most significant. Hypoxia causes increased cerebrospinal fluid (CSF) pressure, fluid retention, fluid shifts, and impaired gas exchange. Although no one is exempt from the effects of hypoxia, the onset and severity of symptoms varies among individuals. Factors that influence an individual’s threshold for hypoxia include physical activity, sleep, physical fitness, metabolic rate, diet, nutrition, emotions, and fatigue. Alcohol ingestion and smoking act as respiratory depressants and will exacerbate the effects of hypoxia. Exposure to temperature extremes will increase a person’s metabolic rate, increasing oxygen requirements and reducing the hypoxic threshold.
The body responds with both immediate and chronic physiologic adaptations to the hypoxic environment. Through acclimatization, a series of physiologic adjustments works to restore the tissue oxygen pressure to near its sea level value. Successful acclimatization varies between individuals and cannot be predicted by physical conditioning, examination, or testing.
A slow, graded ascent is the key to acclimatization; that is, staging, especially for individuals who have previously exhibited sensitivity to high-altitude changes.5 In the ideal setting, the first night’s sleep occurs at <8000 ft, with the first day spent at rest; then the daily ascent should be limited to 1000 ft. Beyond 14,000 ft, 2 days of rest should be taken for each 1000-ft ascent. Climbing to higher elevations during the day and descending to a lower altitude to sleep is another option to prevent AMS and facilitate acclimatization.
Pharmacologic agents may also be beneficial adjuncts to acclimatization. Acetazolamide has been shown to be effective when staging is not possible or with individuals who are at an increased risk of HAI.6 Dexamethasone may also be effective in preventing AMS, but it is generally reserved for treatment.
As an individual ascends, the hypoxic ventilatory response (HVR) will attempt to compensate for the decrease in arterial PO2 through an increase in the respiratory rate. An inadequate HVR, resulting in relative hypoventilation, has been suggested as the etiology for AMS and HAPE. Individuals with low tidal volumes and children are less able to respond to the hypoxic insult and therefore are more prone to AMS. HVR is genetically predetermined, but can be influenced by caffeine, alcohol, and various medications. Further, hypoxia from chronic heart or lung problems desensitizes this effect.
The threshold for the HVR is at approximately 4000- to 5000-ft elevation, with the maximum response occurring at 22,000 ft. At this elevation, the minute volume will be nearly doubled.
The initial maximal effect of the HVR occurs approximately 6 to 8 hours after arrival to altitude. A decline in the partial pressure of inspiratory oxygen (PIO2) results in an increase in ventilation, and decreases in the PACO2 accordingly. The falling PACO2 causes a mild respiratory alkalosis and a shift of the oxyhemoglobin dissociation curve to the left. The result is increased binding of oxygen with hemoglobin for transport to the tissues. The respiratory alkalosis then provides negative feedback to the medulla, restricting the HVR. Compensation for this respiratory alkalosis is dependent on the renal excretion of bicarbonate, usually occurring within 24 to 48 hours. Ventilation will slowly increase as the pH returns to normal. With no further ascent, this response can take 4 to 6 days. As an individual continues to higher elevation, subsequent acclimatization will be directed by the declining arterial PCO2.
Hypoxia and cold stressors act as significant vasoconstrictors of the pulmonary vascular bed, resulting in an elevation of the pulmonary arterial pressure and an increase in the workload on the right side of the heart. The degree of pulmonary hypertension in response to global hypoxia is an important contributor to the development of HAPE.
Compared to the respiratory and central nervous systems, the cardiovascular system is relatively resistant to hypoxia. The cardiovascular system responds to hypoxia in two phases. In the first phase, the heart rate begins to increase at an altitude of 4000 ft. The second phase occurs when the decrease in PAo2 releases an increased catecholamine surge causing selective vasoconstriction, resulting in a slight increase in blood pressure, thereby increasing the cardiac output. As acclimatization occurs, the heart rate will return to normal. If the individual fails to acclimatize, the heart rate will remain elevated, and any increase in cardiac activity will require more oxygen.
The hematopoietic response to high altitude is a critical element in an individual’s long-term ability to acclimatize. All changes within the hematopoietic system are geared toward increasing arterial oxygenation. Within hours of ascent, erythropoietin output is increased in response to the hypoxia. In approximately 4 to 5 days, there will be an increase in circulating red blood cells. This increase in red cell mass persists for 1 to 2 months after return to lower altitudes. At extreme altitudes, this hematopoietic response is detrimental due to the increased blood viscosity, hemoconcentration, and increased red blood cell mass impeding oxygen transport.
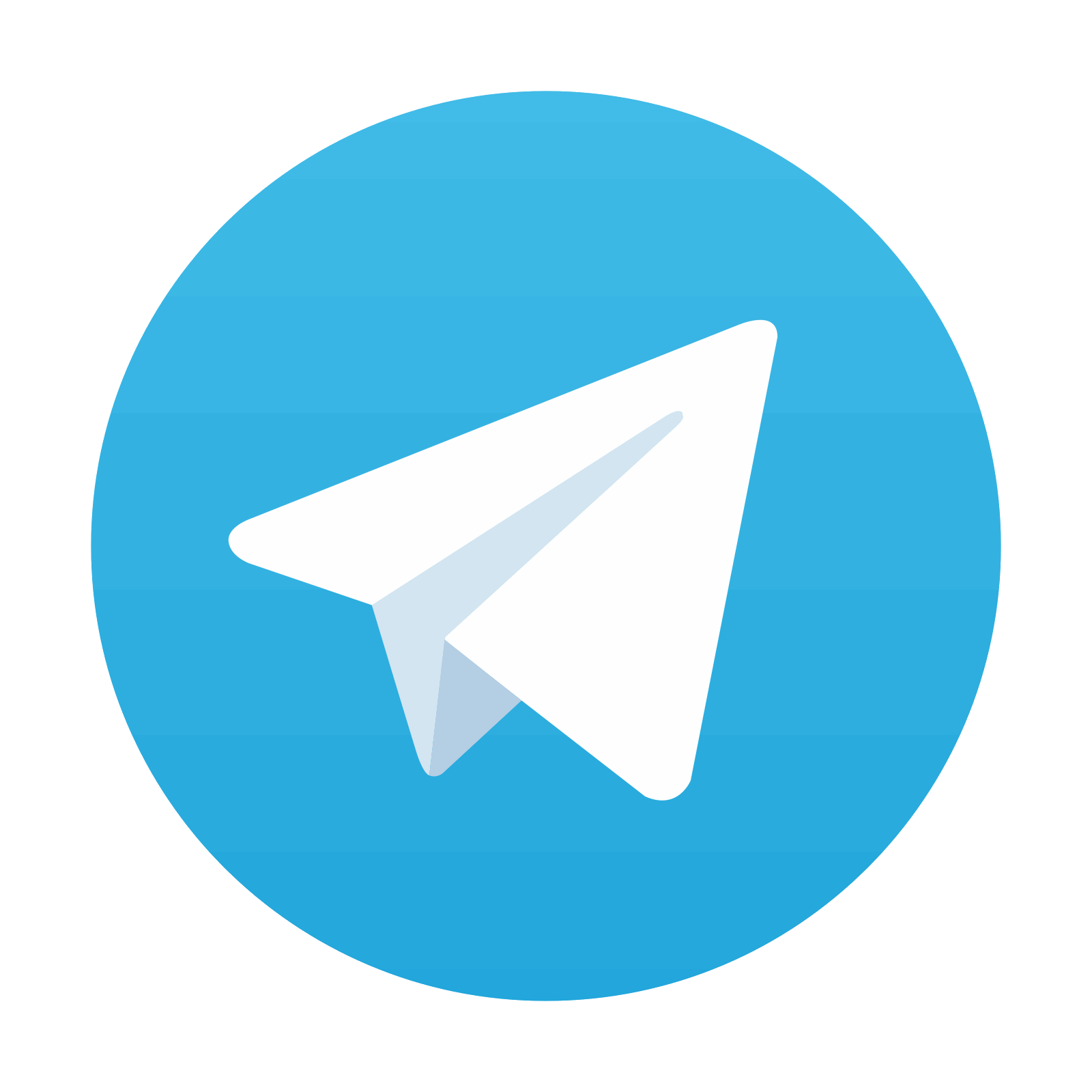
Stay updated, free articles. Join our Telegram channel

Full access? Get Clinical Tree
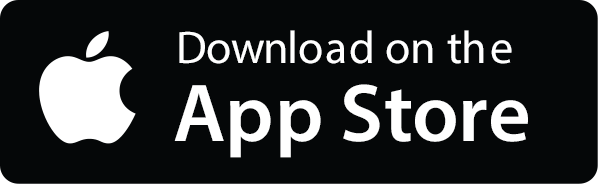
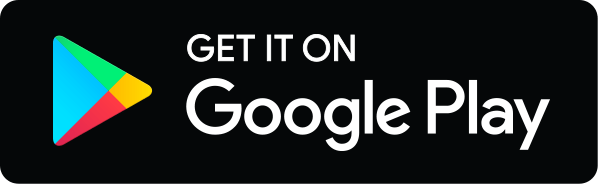