Hepatic Dysfunction
Mauricio Lisker-Melman
Gowri Kularatna
Liver function abnormalities are detected in about 50% of intensive care unit (ICU) patients, often leading to hepatology consultation [1]. The presentation of hepatic dysfunction range from simple abnormalities in biochemical tests with little impact on a patient’s clinical course to complex manifestations of liver failure that require prompt intervention and have high morbidity and mortality. Etiologies of hepatic dysfunction are many and variable in this setting. Sometimes, a detailed clinical history is sufficient to establish the cause of the derangement; however, a combination of clinical experience and judicious use of supplemental testing is required to establish a specific diagnosis and suggest a therapeutic course of action. Understanding the anatomic interactions between the liver and other organs and the physiologic principles that determine hepatic function is essential for establishing a rational therapeutic approach for each disorder. In this chapter, we outline aspects of liver physiology that are altered in critically ill patients and review common disorders of hepatic dysfunction seen in this setting.
Physiologic Considerations
Blood Flow
In resting conditions, the liver receives 25% of the cardiac output and 10% to 15% of the total body blood volume. About 25% of the liver volume consists of blood (capacitance function). The human liver has dual blood supply. Approximately one third of the hepatic blood flow is supplied by the hepatic artery (low-flow, high-pressure system, well-oxygenated blood) and two thirds by the portal vein (high-flow, low-pressure system, poorly oxygenated blood). The hepatic artery supplies the capsule of the liver and bile ducts. The portal vein is formed by the splenic vein, superior mesenteric vein, and inferior mesenteric vein (it drains into the splenic vein). The left gastric vein branches from the portal vein and plays a fundamental role in the formation of esophageal varices in patients with portal hypertension. The portal vein provides venous drainage for
several abdominal organs: pancreas, stomach, intestine, and spleen. Once within the liver, arterial and venous bloods mix in the hepatic sinusoids [2]. The sinusoids are involved in the exchange between the blood, space of Disse, and the hepatocytes. The blood flowing through the sinusoids is collected in the central veins and then into the hepatic veins and inferior vena cava.
several abdominal organs: pancreas, stomach, intestine, and spleen. Once within the liver, arterial and venous bloods mix in the hepatic sinusoids [2]. The sinusoids are involved in the exchange between the blood, space of Disse, and the hepatocytes. The blood flowing through the sinusoids is collected in the central veins and then into the hepatic veins and inferior vena cava.
The sinusoids are composed of fenestrated endothelial cells, Kupffer cells, stellate cells, and natural killer (NK) cells. Endothelial cells represent 50% of the sinusoidal cells. They contain numerous fenestrae (not uniform in size or distribution) with dynamic structure and function. Endothelial cells have several functions including endocytosis, secretion (interleukins, interferon, eicosanoids, endothelin, and nitric oxide), and expression of adhesion molecules. Kupffer cells are phagocytic and remove infective, toxic, and foreign substances from the portal blood. They also release substances involved in the immune response by the liver. Stellate cells, also known as lipocytes, fat storing cells, or Ito cells, store fat vacuoles (major storage sites of retinoids). They have contractile activity regulating the blood flow through the sinusoids. The NK cells, also known as Pit cells are liver-associated lymphocytes with azurophilic granules with lysosomal activity [3,4].
Blood flow through the liver varies considerably under different physiologic conditions. In the normal state, the liver extracts less than half of its supplied oxygen (4.6 mg per minute per 100 g liver). Thus, in most conditions of increased oxygen demand, the liver can increase oxygen extraction without an alteration in blood flow [5]. Regulation of total hepatic blood flow occurs primarily at the level of the hepatic artery. If portal venous inflow decreases, compensation is accomplished through vasodilatation of the hepatic artery. However, reductions in mean arterial pressure below 50 mm Hg exceed the capacity of the autoregulatory mechanisms to maintain adequate liver perfusion [6].
Bilirubin Metabolism
The majority of bilirubin (80%) is generated from the breakdown of heme released by senescent red blood cells. Unconjugated bilirubin (indirect bilirubin) circulates bound to albumin before entering the hepatocyte through an active process mediated by transporter proteins. Once inside the hepatocyte, unconjugated bilirubin is transferred to the smooth endoplasmic reticulum, where it is conjugated with glucuronic acid (conjugated or direct bilirubin) and, in turn, secreted into the biliary canaliculi by a pump called multidrug resistance protein 2. Once in the gastrointestinal tract, bilirubin is deconjugated by gut bacteria enzymes and oxidized to stercobilin and eliminated in the stool. Stercobilin is also reabsorbed in the small intestine, passes the liver, and is reexcreted through the kidney (urobilirubin) in the so-called enterohepatic circulation [7].
Unconjugated hyperbilirubinemia results from increased bilirubin production (e.g., ineffective erythropoiesis, hemolytic disorders), impaired uptake (e.g., Gilbert’s syndrome, use of certain drugs such as rifampin), or defective conjugation (e.g., Gilbert’s syndrome, Crigler–Najjar syndrome types I and II). In the physiological jaundice of the newborn, the bilirubin metabolism is affected at various levels (increased disruption of hepatocytes, reduced hepatocyte uptake, intracellular transport and conjugation, and increased enterohepatic circulation) resulting in unconjugated hyperbilirubinemia. Breast milk jaundice is another form of unconjugated hyperbilirubinemia attributed to the ingestion of breast milk components that affect the enzyme that conjugates bilirubin [8]. Conjugated hyperbilirubinemia results from a wide spectrum of familial (Dubin Johnson syndrome and Rotor syndrome), hepatocyte, and biliary disorders that are associated with a benign course, acute or chronic liver cell damage, cholestatic injury, or biliary tree obstruction (intra- or extrahepatic) [9].
Drug Metabolism
The liver is positioned strategically between the digestive tract and the systemic circulation. Only those substances absorbed by the oral mucosa and the rectum bypass the liver, reaching the systemic circulation directly. Several diverse pathways participate in the metabolism of drugs and toxins by the liver. Three main enzymatic pathways participate in drug metabolism: oxidation, hydrolysis, and reduction, catalyzed by oxidoreductases, hydrolases, and transferases. The oxidoreductases and hydrolases catalyze phase I reactions that increase polarity (or water solubility) of substances and potentially generate toxic metabolites. The transferases catalyze phase II reactions through conjugation and produce less toxic and biologically less active products when compared with the parent compound. The most important drug-oxidation system is the P450 system (the electron-transport chain associated with the microsomal system). The central protein in this system is cytochrome P450, a hemoprotein [10]. The primary reactions, biochemical or physiologic changes, and toxic consequences to the liver of drug and toxin exposure may be variable and in part dependent on the interaction with the host [11,12].
Drug-induced liver injury (DILI) has a difficult to calculate incidence, ranging from asymptomatic liver chemistry abnormalities with a small potential impact to life or function to fulminant liver failure with high morbidity and mortality. A thorough medication history obtained from the patient, relatives, friends, caregivers, and pharmacy records is essential to identify and substantiate this challenging clinical problem. Factors to be considered when assessing patients with suspected DILI include clinical presentation and timeline of symptoms, timeline of drug ingestion, concurrent liver disease and other potential etiologies of liver injury, concomitant medications, herbal and substance abuse, biochemical pattern of liver injury, histologic findings, and response to rechallenge [13].
Hemostatic Function
The liver is the primary site of synthesis of most of the coagulation factors and the major inhibitors of the activated coagulation cascade. The synthesis of procoagulant factors II, VII, IX, and X and anticoagulant factor proteins C and S depends on the presence of vitamin K. The adequacy of hepatic synthetic function can be estimated by the prothrombin time or international normalized ratio (INR) [14]. In the presence of liver disease, there is reduced synthesis of clotting factors and inhibitors of coagulation. The synthesis of abnormal clotting proteins with anticoagulant activity leads to disseminated intravascular coagulation (DIC) and enhanced fibrinolytic activity [15,16]. In addition, thrombocytopenia, associated with portal hypertension and hypersplenism, and thrombocytopathy are usually identified in patients with end-stage liver disease. Consequently, most patients with liver disease have some measurable defect in hemostasis involving the coagulation system, fibrinolytic system, platelets, and reticuloendothelial system (Kupffer cells). The resultant clinical impact of this bleeding diathesis, however, is of variable importance. When and how the physician institutes therapeutic or prophylactic hemostatic interventions is debated and must be individually assessed for each patient.
Clinical Disorders
Ischemic Hepatitis
Liver ischemia is the consequence of hypoxic liver insult and presents in the setting of reduced liver blood flow, persistent hypotension, or severe hypoxemia. Common synonyms for ischemic hepatitis include acute hepatic infarction, shock liver, and hypoxic hepatitis [17,18]. The hepatic insult is diffuse, noninflammatory in nature, and results in variable degrees of central vein (zone 3) necrosis and collapse. The syndrome typically is recognized through detailed clinical history and biochemical evaluation, as the precipitating factor may not be apparent. Dehydration, heat stroke, hemorrhage, cardiogenic shock, acute decline in cardiac output in the absence of hypotension, traumatic shock, respiratory failure, aortic dissection, pulmonary embolus, and extensive burns have been associated with ischemic hepatitis [19,20,21,22]. In patients with congestive heart failure (left ventricular failure) and chronic passive liver congestion (right ventricular failure), even minor, additional insults may precipitate liver ischemia [23]. It is difficult to estimate the prevalence of ischemic hepatitis, but approximately 1% of patients in the ICU develop severe hypotension, cardiac failure, or respiratory failure and are susceptible to developing this condition [24].
The clinical presentation is highly variable. Biochemically, there is a characteristic rapid rise in serum aminotransferases, reaching 10 to 40 times the upper limits of normal. The lactate dehydrogenase also increases dramatically, whereas abnormalities in serum bilirubin, alkaline phosphatase, and prothrombin time are less striking. Peak elevations occur in the first 72 hours. In situations in which the triggering factor resolves, normalization of laboratory tests may occur over 7 to 10 days. Other chemistry abnormalities include renal failure with increased blood urea nitrogen and serum creatinine. Patients may present with hepatic encephalopathy, mild jaundice, weakness, or general malaise. More typically, the dominant clinical features are those of the disorder that triggered the ischemic insult [25]. The differential diagnosis includes other disorders associated with significant, rapid increases in liver enzymes, such as acute viral hepatitis, alcoholic hepatitis, and drug-induced hepatotoxicity. In only rare instances is a liver biopsy necessary for diagnosis [24].
Treatment of ischemic hepatitis is directed at correction of the underlying disease or factor that initiated the liver damage. The aim of treatment is to improve cardiac output, optimize liver and peripheral organ perfusion, and improve tissue oxygenation. The specific intervention depends on the precipitating factor and varies from case to case [26,27]. Ischemic hepatitis frequently is self-limiting, and recovery is associated with normalization of the hepatic architecture. From the liver standpoint, the prognosis depends on the presence of a normal or previously damaged liver and on the etiology of the underlying disorder [24,25,28].
Congestive Hepatopathy
Congestive hepatopathy and passive hepatic congestion are interchangeable terms used to refer to the outcome of increased hepatic vein pressure from a variety of causes. The increased pressure is transmitted through the hepatic veins and venules to the hepatocytes resulting in initial damage to cells in zone 3 [29]. Additional liver damage is thought to occur from decreased hepatic flow and decreased arterial oxygen saturation [30]. The most common causes are ischemic cardiomyopathy, heart failure, valvular heart disease, restrictive lung disease, and pericardial disease. Right-sided heart failure of any etiology (constrictive pericarditis, tricuspid regurgitation, mitral valve stenosis, or cardiomyopathy) increases the pressure of the inferior vena cava and the hepatic veins and ultimately produces liver congestion [31,32,33]. Although the clinical presentation of hepatic vein thrombosis (Budd–Chiari syndrome), primary thrombosis limited to hepatic venules, sinusoidal obstructive syndrome (formerly known as venoocclusive disease), and inferior vena cava thrombosis at its hepatic portion (obliterative hepatocavopathy) may be similar to congestive hepatopathy, accurate differential diagnosis is imperative. The workup of these conditions includes various imaging modalities, such as ultrasound with Doppler flow, fluoroscopic cavography, and magnetic resonance venography; at times, liver biopsy is needed for a definitive diagnosis. These medical conditions have different etiologic factors and treatment approaches [34,35,36,37].
The patient with liver congestion may present with signs and symptoms of right-sided heart failure and only subtle abnormalities in liver chemistries. The aminotransferases may be mildly elevated, reflecting limited degree of liver cell necrosis. Mild elevations of the alkaline phosphatase and total bilirubin are also common. In more severe presentations, the patient may be deeply jaundiced, suggesting extrahepatic biliary obstruction. Congestive hepatopathy can eventually lead to development of hepatocellular necrosis, broad fibrous septa deposition, regenerative nodule formation, architectural derangement, and frank cirrhosis, previously termed cardiac cirrhosis. Congestion produces tender hepatomegaly, and a pulsatile liver can occur with tricuspid regurgitation. Hepatojugular reflux may be elicited, and ascites is a frequent finding. In these patients, the ascitic fluid albumin is high (> 2 g per dL); in contrast, in noncardiac cirrhosis, the ascitic fluid albumin is typically lower (< 2 g per dL) [33,38]. The serum albumin to ascites albumin gradient (SAAG) is more than 1.1 g per dL in both conditions. Diagnosis rests on a combination of a high index of suspicion and studies that confirm the presence of cardiopulmonary disease. Pressure measurements through cardiac catheterization, transjugular hepatic venous pressure gradients, and cardiac imaging studies are diagnostic.
A transjugular liver biopsy, ideally obtained at the time of pressure measurements, can be helpful in difficult cases. Classic biopsy findings include centrilobular parenchymal atrophy, sinusoidal and terminal hepatic venular distention, and red blood cell congestion and extravasation into the space of Disse. In addition, perisinusoidal collagen deposition is seen in chronic congestion. Treatment is focused on management of the underlying pulmonary, cardiac, or pericardial disease. Ascites is managed with diuretics, low-salt diet, or large-volume paracentesis. Transjugular intrahepatic portosystemic shunts (TIPSs) are contraindicated in this condition, as the resulting marked increase in the right heart and pulmonary arterial pressures may precipitate severe heart failure [25].
Total Parenteral Nutrition
Total parenteral nutrition (TPN) remains a vital medical intervention, and its use has become routine to provide nutrition to those who are unable to eat or tolerate enteral nutrition (short gut syndrome, Crohn’s disease, radiation enteritis, severe pancreatitis, post-op periods, etc.). Hepatobiliary dysfunction is recognized as a major adverse effect of short-term and long-term TPN use [39,40]. Variable degrees of liver dysfunction, ranging from subtle laboratory abnormalities to clinically apparent liver disease, develop in 40% to 60% of infants and 15% to 40% of adults who require long-term TPN [41,42,43]. The wide prevalence ranges reflect the difficulty in ascribing liver dysfunction to TPN, particularly in the ICU where the etiology of liver abnormalities may be multifactorial. The spectrum
of hepatobiliary complications attributable to TPN includes hepatic steatosis, intrahepatic cholestasis, biliary sludge, and cholelithiasis [44]. TPN-related complications are more commonly seen after prolonged periods of parenteral nutrition. Cholestasis predominates in infants (occurring in 40% to 60%), hepatic steatosis and steatohepatitis predominate in adults (occurring in up to 40% to 55%), while biliary sludge and cholelithiasis affect both groups [43]. Progression to cirrhosis and portal hypertension is rare and occurs more frequently in infants and neonates than in adults [43].
of hepatobiliary complications attributable to TPN includes hepatic steatosis, intrahepatic cholestasis, biliary sludge, and cholelithiasis [44]. TPN-related complications are more commonly seen after prolonged periods of parenteral nutrition. Cholestasis predominates in infants (occurring in 40% to 60%), hepatic steatosis and steatohepatitis predominate in adults (occurring in up to 40% to 55%), while biliary sludge and cholelithiasis affect both groups [43]. Progression to cirrhosis and portal hypertension is rare and occurs more frequently in infants and neonates than in adults [43].
Most patients with hepatic steatosis are asymptomatic. Mild elevations of aminotransferases, alkaline phosphatase, and total bilirubin may be identified in up to 70% of patients [45]. Enzyme levels usually peak within 1 to 4 weeks of TPN initiation. The elevation is often transient and complete resolution may occur spontaneously despite continued use of TPN. Hepatic steatosis is most likely a direct consequence of a high carbohydrate load and defective secretion of triglycerides by the liver. Glucose or dextrose overfeeding (> 50 kcal per kg per day) should be avoided [46]. With the development of currently accepted protocols for caloric intake (25 to 40 kcal per kg per day), including lipids as an alternative calorie source, the prevalence of liver steatosis has declined significantly [47,48]. In a prospective study, a 53% versus 17% reduction in hepatic steatosis was reported in patients who received only dextrose infusion when compared with mixed dextrose and lipid emulsion [49]. The use of fish oil–based lipid emulsions (instead of safflower or soybean oil) appears to be “hepatoprotective” in children receiving TPN, but this strategy has not been studied in adults [50].
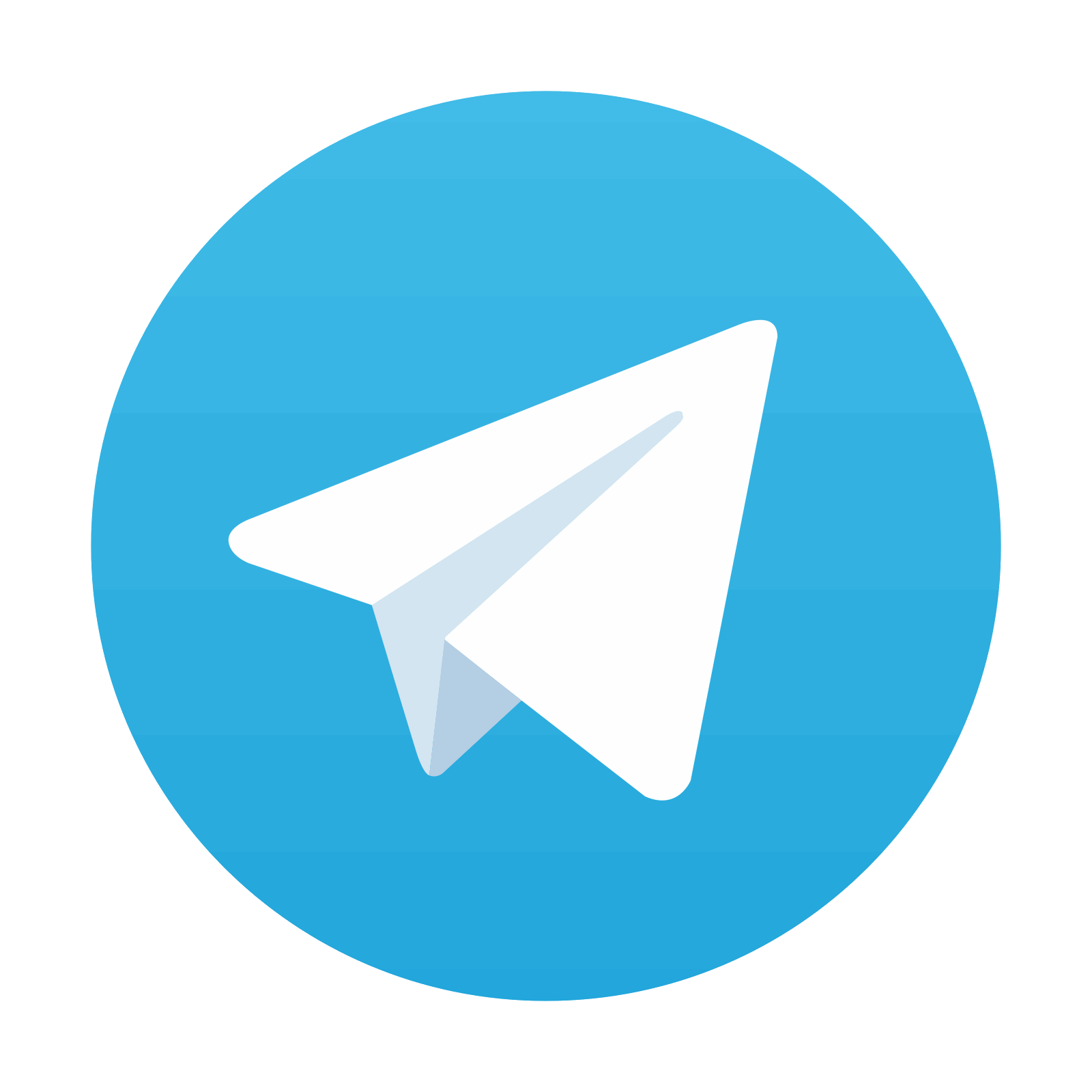
Stay updated, free articles. Join our Telegram channel

Full access? Get Clinical Tree
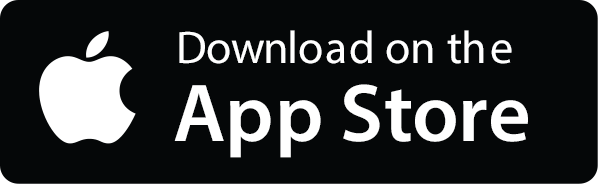
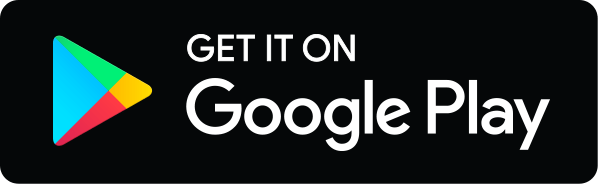