where MCFP is the mean circulatory filling pressure.
By observing the formula, we can see clearly that if MCFP decreases due to blood loss, venous return remains normal as CVP also falls.
A reduction in CVP is therefore a warning sign, even if arterial pressure and heart rate remain normal. If blood loss continues and therefore the circulating mass, the venous return is reduced and along with it the stroke volume, but cardiac output remains normal thanks to an increase in heart rate. We therefore have three signs of a loss of circulating mass: decreased Htc, decreased CVP, and increased heart rate.
These are wonderful multiple compensation mechanisms that make it possible to overcome any oversight by the anesthetist for a certain amount of time, although he/she must also learn to recognize them early: reduced Htc, reduced daily diuresis (due to tubular reabsorption of H2O and Na), reduced CVP, and increased heart rate are all signs of a hypovolemic process that is compensated for but at risk of leading to hemodynamic deterioration which is not desirable.
If not equipped, cardiac output decreases along with pulse and EtCO2 (which are closely linked to output).
However, unless this is a substantial acute hemorrhage, this point is not reached quickly. In fact, the body can rely not only on interstitial fluids but also intracellular fluids (which make up the majority of TBW), which amount to 24 l in patients weighing 70 kg. The passage of water from cells to the interstitium when needed is immediate and follows osmotic logic.
The extracellular compartments (interstitium and circulation) have osmotic forces that are in balance with the intracellular compartment, which is made up of concentrations of cations and anions that ensure electroneutrality in various sectors. The most important cation in the extracellular compartment is Na+ followed by cations with less importance such as K+, Mg+, and Ca++. Electroneutrality is guaranteed by the presence of anions Cl− and HCO3 − and proteins (especially albumin).
In the intracellular compartment, the most important cation is K+, and the anion PO4 − ensures electroneutrality. Osmolarity is determined by the number of particles dissolved in a solution and is therefore fundamentally determined by Na+ in the plasma and interstitium and K+ in the cells.
These concepts, as far as expected, are very important since they regulate the distribution of water in the body. This can pass freely and rapidly from compartments with low osmolarity to others with greater osmolarity by maintaining identical osmolarity in various compartments.
Why is the intracellular water content much higher than the extracellular content?
This is simply because there is a K salt content in cells that is greater than the Na salt content in extracellular compartments. A change in osmolarity in one area triggers an immediate movement of water to restabilize the balance. This game of water movement applies to the balance between intracellular and extracellular interstitial spaces since the cell membrane is not permeable for ions, and therefore water movement is considerably linked to osmotic forces. In the relationship between capillaries and the interstitium, the mechanism is different since the capillary endothelium is permeable to ions which are therefore no longer part of the osmotic force that can lead to the movement of water. The passage of water is regulated by Starling’s law, and the forces at play are mainly the hydrostatic pressure and oncotic pressure of the capillary and interstitium.
Therefore, when a patient experiences rapid blood loss, the lost volume is quickly replaced by fluids from the interstitium. This results in the maintenance of the hemodynamic balance, and the phenomenon is revealed only due to a reduction in Htc, which is nevertheless moderate since autotransfusion of whole blood from the splanchnic reservoir also occurs at the same time.
The tolerance of fluid overloading is just as amazing as the negative effect of blood loss is marvelously “muted.” When there is an inappropriate overloading of crystalloids, a kidney response suddenly enters into play, and when the possibility of a diuretic renal response is overcome, the increased hydrostatic pressure in the capillary along with a reduction in oncotic pressure due to dilution thereof pushes excess fluid into the interstitial space, thus maintaining a normal hemodynamic pattern.
These compensation mechanisms (and we have only described some of them) are initially capable of covering up our carelessness.
We have described only some of the forms of compensation activated by the body in the event of volemic imbalance.
In reality we have described those that can most easily be discovered (decreased Htc, decreased CVP, decreased diuresis, increased heart rate). But there are other more complex mechanisms that require greater acuity and control to demonstrate. I am referring to the renin-angiotensin-aldosterone system (RAAS) and the action of ADH.
At this point it is essential to refer to effective circulating volume (ECV): this is the volume of blood distributed in the arterial system intended for perfusion of the tissues. It is not a measurable parameter and is regulated by baroreceptors in three areas:
1.
The carotid sinuses that regulate sympathetic activity and, to a lesser extent, the release of ADH. One of the main functions of this apparatus is the maintenance of cerebral perfusion.
2.
The juxtaglomerular apparatus that regulates the activity of the RAAS and therefore glomerular perfusion and glomerular filtration.
3.
The atria and ventricles that release natriuretic peptides in response to increases in pressure.
If cardiac output decreases, the regulation systems enter into play to restore it. For example, in a patient with cardiac insufficiency, upon reduction of output the carotid sensors stimulate sympathetic activity, and those of the juxtaglomerular apparatus activate the RAAS. These responses promote an increase in heart rate and sodium retention and therefore an increase in circulating blood volume that causes greater distension of the cardiac chambers and, according to Starling’s law, an increase in cardiac output, which is also promoted by the increased heart rate.
The response of these regulation systems is therefore initially favorable, but, if cardiac insufficiency and the Na-sparing mechanism continue, the blood volume increases later on even if the heart is no longer able to empty the venous return: this leads to blocked circulation, edemas, dyspnea, and pulmonary edemas.
In the case of advanced-phase cardiac sufficiency, the blood volume increases, while the ECV decreases.
It is confirmation of the fact that volemia and ECV are two values that are not necessarily correlated.
We have highlighted the concept of ECV and described the example of chronic cardiac insufficiency to draw attention to the fact that volemic overloading may coexist even if ECV is reduced.
The aim of this article is to attract the attention of anesthetists to apparently normal situations which in reality are the result of compensation mechanisms, but providing advice for better diagnosis and management.
6.1 Fluid Dynamics in the Capillary
6.1.1 The Importance of the Glycocalyx
The mechanism that regulates fluid movement in the capillaries was described by Starling in 1896 and is still largely applicable. Fluids are maintained within the capillaries thanks to oncotic pressure from plasma components. Oncotic pressure counters hydrostatic pressure which pushes fluids into the capillaries and therefore the interstitium. In the interstitium both hydrostatic and oncotic pressure are lower than in the endocapillary plasma. The net result is a constant moderate passage of fluids from capillaries to the interstitium where any excess fluid is immediately removed from the lymph nodes and rechanneled towards the heart. The endothelial barrier is permeable for water and small molecules such as sodium, potassium, chlorine, and glucose, which pass freely via specialized pathways.
The macromolecules can be transported via larger pores or vesicles. The movement of fluid across the capillaries can be distinguished as two types: Type 1 (physiological) occurs continuously and, as mentioned above, is removed from the lymph nodes and Type 2 (pathological) is seen when the barrier is damaged or does not function properly: this results in the formation of edemas.
If it is true that the physiological basis that regulations of fluid movement across the capillaries are still the old Starling’s law, we must, however, include much more recent information that currently provides us with particularly useful data on the perioperative management of fluids.
The new information essentially relates to demonstrating the presence of the glycocalyx system at the endothelial level.
The endothelium is made up of a wafer-thin barrier formed of a single layer of cells (endothelial cells). In the part facing the lumen, the endothelium is covered by a thin, fragile membrane, the glycocalyx, which is the first barrier regulating the transport of macromolecules and cells.
This barrier, which is made up of glycoproteins and proteoglycans and contains glycosaminoglycans, traps red blood cells and protein-rich plasma. We must therefore today consider the intravascular fluid volume as having two distinct parts: that which is noncirculating and is trapped in the glycocalyx system and that which is circulating.
The endothelial wall is therefore made up of endothelial cells and the glycocalyx and is 0.4–1.2 μm in thickness; it is in continuous dynamic balance with the circulating plasma.
To function normally, this barrier requires a normal level of albumin. In hypoalbuminemic states, which are very common in our patients, the barrier does not work properly, and, even with a reduction in oncotic pressure, large quantities of fluid pass from the capillary to the interstitium, and when the lymph nodes are no longer able to empty them, edemas occur.
When we administer iso-oncotic colloids to our patients, we increase capillary hydrostatic pressure, but oncotic pressure remains constant: this situation encourages a more moderate passage of fluids towards the interstitium. When, however, we administer crystalloids, hydrostatic pressure increases, as in the case of colloids, but oncotic pressure falls: this leads to the passage of more fluids from the capillary to the interstitium.
The endothelium and the glycocalyx systems are not only a barrier between intravascular liquids and the interstitium, but play an important role in hemostasis, platelet aggregation, leukocyte adhesion, and permeability of the barrier. In perioperative phases, therefore, it is mandatory to protect the barrier from events that may damage it.
Negative factors are processes of ischemia/reperfusion, hypoxemia/reoxygenation, proinflammatory cytokine, and BNP. In particular we must draw readers’ attention to BNP since it is of great significance in anesthesiological practice.
In the case of fluid overloading, whether iatrogenic (excessive administration) or resulting from pathological states (chronic cardiac insufficiency), distention of the cardiac chambers leads to increased BNP release, which has a harmful effect on the glycocalyx system [1].
Fluid overloading therefore does not only increase the hydrostatic pressure of the capillary but damages the barrier, transforming Type 1 transcapillary fluid movement into Type 2 movement and thus promoting the formation of edemas.
6.1.2 The Distribution of Crystalloids and Colloids
The use of a glycosylated solution to expand the circulating volume is destined to be unsuccessful. In fact, glucose is rapidly metabolized by the liver, and therefore administering a glycosylated solution is equal to administering free water.
Water passes through all barriers freely and rapidly, and therefore 1,000 ml of an infused glycosylated solution on 7 % (the equivalent of 70 ml) remains in the circulation, adding to the other 4 l of intravascular water. The remaining water is distributed by balancing itself with other compartments where water totals 24 l and/or is eliminated via diuresis.
The use of 1,000 ml of a 0.9 % saline solution remains confined in the extracellular space (interstitium + circulation) since sodium cannot enter the larger intracellular space. Therefore a greater proportion of the solution compared to the glycosylated solution remains in the bloodstream (approx. 20 % or 200 ml out of 1,000 ml, compared with 7 % or 70 ml out of 1,000 ml for the glycosylated solution).
Colloids – hydroxyethylamides, gelatin, or albumin solutions – remain longer in the bloodstream compared with crystalloids since the dimensions of their molecules do not facilitate transcapillary movement.
This passage nevertheless occurs. It has been shown that a colloid administered in normal volemic situations, whether 6 % hydroxyethylamide or a 5 % albumin solution, enters into the interstitia in a few minutes at a rate of 68 %. If, however, these plasma expanders are administered to a hypovolemic patient, leading to normovolemic hemodilution, the amount of fluid remaining in the circulation in this period is roughly 90 % [4].
This observation is important for clinical practice. If we administer a plasma expander to a patient who needs it, and who is hypovolemic, we have greater persistence in the circulation and less passage into the interstitia. If, however, we administer it to a patient who does not require it, it rapidly enters the interstitia: this mechanism protects the circulation from an excessive rise in hydrostatic pressure but also has negative consequences that trigger pruritus and edema.
Recently a comparison has been made between 0.9 % saline, gelatin, and 6 % hydroxyethylamide in healthy young subjects. After 1 h of infusions, 68 %, 21 %, and 16 % of infused liquids, respectively, left the circulation [5].
The difference between the two colloids used was therefore moderate, but their molecular weight varied greatly: 30 KDa for gelatin and 130 KDa for hydroxyethylamide.
In the same study the response of the RAAS was assessed for the three infusions: all reduced it by depriving the response of renin and aldosterone and by promoting the elimination of H2O and Na. This observation confirms that it had already been demonstrated in the past that the elimination of H2O and Na in acute hypervolemia depends essentially on suppression of the RAAS. Even BNP increases iatrogenic hypervolemia, but its diuretic effect in this situation is weaker. As mentioned above, however, the harmful effect of BNP on the glycocalyx is very important.
It is usually thought that the amount of crystalloids to be infused in the case of acute hypovolemia is three to four times that of colloids, which are, however, equal in terms of estimated blood loss.
A systematic review of the issue seems to result in the recommendation of a 2:1 ratio [6].
What is certain is that the administration of colloids enables greater linear recovery of cardiac filling and therefore CO in all patients, whether or not they have sepsis, for saline solutions.
We must not, however, ignore the study recently published in the New England Journal of Medicine which examines the use of hydroxyethylamide in septic patients in whom the use of this drug compared with saline solutions would be greatly burdened by adverse events and mortality [7].
In cases of multiple trauma, however, the use of hydroxyethylamide seems to enable faster clearance of lactate and a lower incidence of renal damage compared with the use of saline solutions [8].
In concluding this short section, it appears possible to say that, in the presence of a perioperative hypovolemic state, it would be preferable to use colloids rather than crystalloids, which would be used exclusively to rebalance the physiological losses (perspiration and diuresis), which are abundantly and rapidly compensated for by fluid movements from extravascular compartments, whether intracellular or extracellular.
6.2 Perioperative Management of Fluids: Comparison of Liberal, Restrictive, and Goal-Directed Strategies
The goal of maintaining good organ perfusion is still too often pursued in operating rooms with high levels of crystalloid administration. This approach is supported by the conviction that surgery patients who have fasted for at least 12 h are often prepared for intestinal surgery with enema and have lost fluids through perspiration and diuresis or as a result of hypovolemia. The belief that during surgery an irrelevant quantity of body fluids escapes towards a “third space,” which has never been determined, is just as widespread.
This so-called third space has been discussed since the 1960s [9] when research with techniques based on the use of a tracer in patients undergoing major abdominal surgery demonstrated that in these patients a loss of water from the extracellular compartment is confirmed even without blood loss.
It is believed that an amount of fluid is discharged into a compartment that is then called a “third space” and that it is identified in the gastrointestinal apparatus and tissues that have been damaged during surgery. Based on this assumption, which is fantastical and minimally supported by research, the liberal use of crystalloids has become the standard, not only to compensate for diuresis and perspiration, but also for the amount of fluids discharged into the “third space” and reduced from the intravascular amount. As a result of this perioperative fluid management strategy, a consistent increase in weight (of up to 10 kg!) in patients in the immediate postoperative period has become very common in recent years.
A systematic review of the studies in which the behavior of perioperative extracellular volume has been controlled has very recently discredited the theory of the “third space” [10], and therefore currently in the perioperative phase, it is justified to compensate with crystalloids only during diuresis and perspiration.
Perspiration, among other things, is considered less important than in the past, and forty years ago it was shown by Lamke et al. [11] that it can amount to 0.5–1 ml/kg/h during major abdominal surgery.
Therefore, during a classical intervention of this kind, such as hemicolectomy in a patient weighing 70 kg (lasting 3 h), fluid loss via perspiration fluctuates between 100 and 200 ml. If diuresis occurs during the same period at a rate of 150 ml/h, it can immediately be understood that in a patient like the one described above, replacement with 500 ml crystalloids is justified. Higher doses can lead to episodes of hypervolemia which, even if transitory, cause the release of BNP, which, as we have seen, damages the glycocalyx system leading to the transcapillary passage of fluids into the interstitia with a resulting increase in weight, as described above.
In becoming aware of this reality, there is naturally a comparison with a different perioperative fluid management strategy that has been labeled “restrictive.” This strategy has been rapidly embraced in thoracic surgery, in which the advantage in terms of oxygenation and the prevention of respiratory complications is immediately clear.
In abdominal surgery, where the advantages are not particularly evident or resounding, the “restrictive” strategy of fluid therapy has not had such an immediate success.
Even in 2003 it was necessary to conduct a multicenter study, published by Brandstrup et al. in Ann. Surg. [12], to draw attention to the risks of liberal fluid management in the perioperative phase. The authors compared two groups of patients who underwent colorectal surgery: one group was treated liberally with crystalloids, receiving on average 5.4 l of fluids, and the other group, which underwent a restrictive strategy, received 2.7 l.
The postoperative complications were much more common in patients treated with high doses of crystalloids (51 % vs. 33 %), including liver infections, failure of intestinal sutures, and cardiovascular and respiratory damage.
The much-feared renal damage, however, as a result of fluid restriction, was not found. It is sufficient to notice that patients undergoing the restrictive strategy received more colloids, as a percentage, and those with the liberal strategy more crystalloids, which confirms the comparison between the two different theories: one (the restrictive) that used crystalloids mainly to compensate for diuresis and perspiration and/or as a vehicle for drugs, and colloids to compensate for blood loss, and one that uses crystalloids much more liberally, even to treat any type of hypotension if confirmed during the perioperative phase.
It should be pointed out that hypotension during anesthesia is mostly due to vasodilation and could be treated by reducing anesthesia and/or using vasoconstrictors, which, among other things, are much more effective in such cases.
The liberal use of crystalloids, as we have seen, is also based on the old concept of underlying hypovolemia in surgery patients as a result of fasting and, in the case of intestinal surgery, of enema preparations. This concept has been discredited. In 2008 Jacob et al. published a study in Acta Anesth. Scand. which demonstrated the absolute normality of the circulating volume in surgical patients after nocturnal fasting [13].
The considerations made up to now point towards a constantly restrictive use of fluids in major surgery. In reality things are not as simple as this. Alongside a study that is favorable to this type of strategy, there are others that do not show differences in outcome between liberal and restrictive strategies.
Among other things, it is not easy to compare the various strategies used by different working groups, since a universally accepted restrictive strategy has not yet been codified.
Colorectal surgical interventions with miniscule administration of fluids (800 ml) have been reported, and others, such as the multicenter study by Brandstrup mentioned above, in which the average fluid administration was 2,700 ml.
We therefore have before us studies that demonstrate that liberal fluid administration can be harmful, but it seems we can confirm that a valid restrictive strategy for all patients cannot currently be defined with certainty.
Faced with this uncertainty it is even more beneficial to agree on a personalized perioperative fluid management strategy, the so-called goal-directed fluid therapy (GDT), in order to avoid both hypovolemia leading to organ damage due to hypoperfusion and hypervolemia with cardiac overloading, O2 desaturation, and damage to the glycocalyx system.
GDT has been stressed for more than 20 years, but in order to carry it out in the 1980s, a Swan-Ganz catheter was required, and DO2I was recommended at above-normal doses (600 ml/m2/min) to ensure perfusion of the organs even in critical moments.
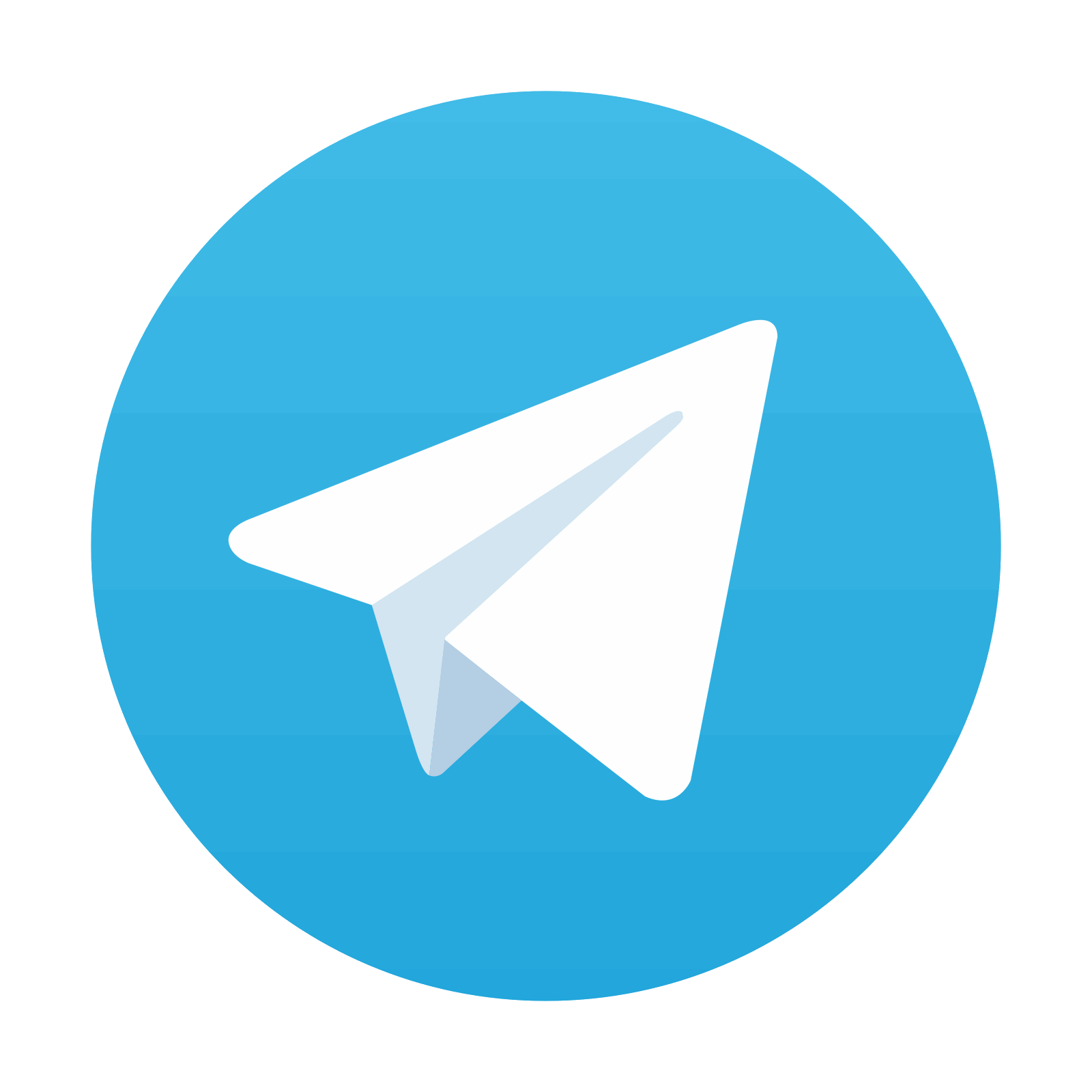
Stay updated, free articles. Join our Telegram channel

Full access? Get Clinical Tree
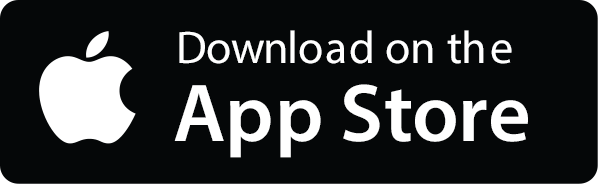
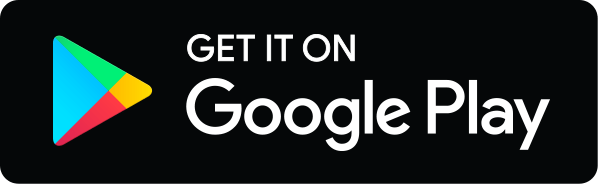