INDICATIONS FOR PULMONARY ARTERY CATHETER INSERTION
Indications for PAC insertion (Table 21.1) have been broadly categorized to (a) precautionary measures in high-risk patients, (b) shock states, (c) pulmonary problems, and (d) cardiac dysfunction.
Preoperative intervention of high-risk surgical patients using PACs remains a controversial area and recommendations are vague in the American College of Cardiology/American Heart Association (ACC/AHA) guidelines (2,29). The key points are as follows: insert the PAC with enough time to achieve the hemodynamic goals (usually the day before), communicate with the anesthesiologist regarding the information obtained while in the intensive care unit (ICU), and monitor the patient in the ICU beyond 24 hours to allow for fluid shifts to occur.
The goals of preoperative invasive monitoring are to (a) optimize preload (plot the Starling curve, see below); (b) optimize CI and stroke volume index (SVI) by adjusting preload, afterload, and contractility (possibly by using inotropes); (c) maintain DO2 to perfuse the rest of the body and prevent MSOF; (d) perfuse the coronaries by maintaining coronary perfusion pressure (CPP) and DO2; (e) decrease myocardial work and myocardial oxygen consumption (MVO2) by keeping systolic blood pressure (SBP) and HR normal; and (f) prevent myocardial infarct by avoiding significant changes (>15%) in HR and BP (19,30,31). Further details in utilization of PACs for treatment of high-risk patients, shock, and cardiopulmonary failure are covered in other chapters.
The PAC is inserted to obtain information beyond the physical examination. Clinical predictors of hemodynamic status in the critically ill patient, such as chest radiograph, jugulovenous distention, and urine output, are inaccurate (1,32). Physicians are correctly able to predict PAOP and CI only 30% to 70% of the time. The PAC provides the following information to guide therapy.
- Central pressures in relationship to the right ventricle (RV) (i.e., central venous pressure [CVP] or right atrial [RA] pressure); central pressures in relationship to the left ventricle (i.e., PAOP to estimate left ventricular end-diastolic pressure [LVEDP])
- Cardiac function measured as CO and presented as CI
- SvO2
- Intrapulmonary shunt (Qs/Qt)
General Considerations
The technical aspects of PAC insertion are presented in “Vascular Cannulation.” It is essential that the catheter be positioned and transduced properly, and a knowledgeable clinician must be able to reliably interpret the data (33–35). Physicians should understand the basic physical principles involved in catheterization, know the design of the catheter, and be able to recognize and remedy technical errors.
Although the modern PAC has features that were not available when it was introduced, the general principles of placement have not changed. If the PAC has fiberoptic bundles at the tip for continuous SvO2 monitoring, external in vitro calibration is done prior to removing the catheter tip from the casing. The PAC is then flushed to assess the patency of its lumens and to fill the catheter with a noncompressible column of fluid capable of transmitting pressures. There is a distal port for monitoring the pulmonary artery pressures (PAPs) and a central port approximately 30 cm from the tip that will lie in the right atrium in the average, adult heart. For CO monitoring, a thermistor is located proximal to the tip to measure temperature changes (see Cardiac Output section). The catheter is placed through the protective sheath and the balloon is checked for integrity prior to insertion. The transducer is placed at the level of the patient’s midaxillary line and zeroed to atmospheric pressure (phlebostatic point). If the transducer elevates above the patient level, the readings will be falsely low. Conversely, if the transducer falls below the patient level, the readings will be falsely high. This is typically noted in beds that are designed to rotate patients.
PRESSURE MEASUREMENTS
Normal hemodynamic values are presented in Table 21.2.
Pressure changes in the heart or vessels cause movement of the catheter, which is then converted to an electrical signal by a transducer. Electrical noise is filtered and the signal is amplified and displayed as a tracing on a bedside monitor. Before insertion, the function of the system is checked by shaking the catheter and seeing good waveforms on the monitor. If the waveform is dampened, the system should be flushed to rid the catheter and tubing of all air bubbles, and all connections should be tightened. After inserting the PAC 15 to 20 cm into the introducer sheath, the balloon is inflated and the catheter is gently advanced. The natural flow of blood from the vena cava through the heart and to the lungs guides the catheter to the pulmonary vasculature (36). While passing from the vena cava to a branch of the pulmonary artery, characteristic waveforms are displayed on the monitor (Fig. 21.1). Once the catheter is advanced to the “wedged” position (PAOP), the balloon is deflated and the catheter adjusted until 1.25 to 1.5 mL of inflation is needed to produce the PAOP tracing. The balloon should only be inflated long enough to record a measurement in order to avoid rupture of the artery or infarction of the downstream segment of lung. The balloon should always be deflated when withdrawing the catheter to avoid vascular and valvular injury. A chest radiograph is performed to assess for pneumothorax and may help to confirm proper position, the catheter should curve gently in the RV and sit in a larger branch of the pulmonary artery (Fig. 21.2). If there is not an obvious PAOP tracing, blood may be sampled from the distal port with the balloon inflated. The sample should have a higher PaO2 and pH with a lower PaCO2 than blood aspirated without occlusion (37). Proper placement is also indicated by the SvO2 signal quality if using a fiberoptic catheter with continuous measurement. The quality of the signal may be altered by (1) fibrin clot at the tip of the catheter, (2) the tip situated against a vessel wall, (3) inserting the catheter too far, or (4) a hypovolemic with collapse of the vessel wall around the catheter tip. If the catheter is in too far, the PAOP tracing will continue to elevate and is called “overwedging” (Fig. 21.3). If this occurs, the balloon should be deflated and the catheter pulled back (≅1 cm) and the balloon inflated again until a good tracing is seen with 1.25 to 1.5 mL of balloon inflation.
TABLE 21.2 Normal Hemodynamic Values | |
![]() |

FIGURE 21.1 Waveforms seen during pulmonary artery catheter insertion.

FIGURE 21.2 Proper position of the pulmonary artery catheter. RA, right atrium; RV, right ventricle; PA, pulmonary artery; LV, left ventricle; AO, aorta.
Because there is a tendency for materials to oscillate at their natural frequencies, the pressure signal may be distorted (38). This effect may be reduced by using noncompliant, short (<4 ft) tubing in the setup of the catheter and monitoring system. The loss of transmitted signal is referred to as damping, and catheters may be over- or underdamped. The degree of damping can be determined by a “fast-flush” device while demonstrating an optimal waveform (Fig. 21.4A). When the catheter is rapidly flushed, a square wave is produced, followed by a series of oscillations before the tracing returns to the baseline pressure reading (39). The appearance of the oscillations demonstrates the degree of damping. Underdamping, which occurs more frequently, is identified by several sharp oscillations and produces higher systolic pressure readings (Fig. 21.4B). Overdamping results in a rounded oscillation and results in lower readings, and may be due to clots, air bubbles, or kinking in the catheter (Fig. 21.4C). Another factor that may interfere with the signal is catheter whip, which results from contraction of the heart. The tracing will show high-frequency distortion and may be minimized by a high-frequency filter built into the transducer system (38).
Whether the patient is on mechanical ventilation (positive intrathoracic pressure at end inspiration) or spontaneously breathing (negative intrathoracic pressure at end inspiration), all pressure measurements should occur at end expiration when the intrathoracic pressure is closest to atmospheric pressure (Fig. 21.5). This point can be determined by watching the patient’s respiratory movements or displaying the airway pressure tracing on the same monitor where the PAP is displayed. If respiratory variation is so pronounced that there is no flat end expiration, then it is best NOT to record a number (Fig. 21.6). In this situation, patients may need to be sedated, or if getting a PAOP is crucial, even paralyzed. Note that the approximation to atmospheric pressure is clearly affected by patients on extreme PEEP. It is ill advised to guess the PAOP; no information is better than wrong information.

FIGURE 21.3 Overwedged tracing. PA, pulmonary artery; PAOP, pulmonary artery occlusion pressure.

FIGURE 21.4 Checking transducer reliability by the “fast-flush square wave testing.”

FIGURE 21.5 Reading of pulmonary artery occlusion pressure (PAOP) at end expiration. During spontaneous breaths, PAOP dips down during peak inspiration due to negative intrathoracic pressure. During mechanical ventilation, PAOP goes up during peak inspiration due to positive pressure ventilation and intrathoracic pressure. In both situations, PAOP should be read at end expiration.

FIGURE 21.6 Excessive variation in pulmonary artery occlusion pressure with forced inspiratory and expiratory efforts precluding accurate measurement due to absence of a stable end-expiratory point.
The first characteristic waveform seen when inserting a PAC is the right atrium (RA) tracing (Fig. 21.7). The tracing can be seen while inserting the catheter or by transducing the right atrial pressure once the PAC is in position. There are two main positive pressure deflections, called the “a” and “v” waves. The “a” wave follows the P wave of the electrocardiogram (ECG) and is due to the pressure increase during atrial systole (Figs. 21.7 and 21.8). The “v” wave results from atrial filling against a closed tricuspid valve during ventricular systole. Between these two positive deflections is a small “c” wave due to tricuspid closure. Two negative deflections called the x and y descents occur when pressure in the atrium decreases. The x descent occurs during atrial relaxation. The y descent is seen when the tricuspid valve opens and blood flows from the atrium to the ventricle.

FIGURE 21.7 Pressure tracings from the right atrial port in normal and pathologic conditions (refer to text).
The best estimate of CVP and PAOP is at end diastole when the atrium contracts. For CVP, where the “c” wave emerges from the “a” wave (also called the z-point) is the optimum reading point. If this point is not clear, read the pressure at the middle of the x descent. Certain patterns in the RA tracing may be seen in disease states (Fig. 21.7). For example,
- “a” waves may not be seen in patients with atrial fibrillation.
- Sawtoothed “a” waves will be present during atrial flutter.
- Large “a,” or “cannon” waves occur during atrioventricular (AV) dissociation when the atrium contracts against a closed valve, or during complete heart block.
- A steep y descent is seen in tricuspid regurgitation and the x descent is not apparent.
- Both descents are prominent in RV infarction.
- Cardiac tamponade tends to cause loss of the y descent due to impairment of ventricular filling.
- In pericarditis, sharp a and v waves are followed by steep x and y descents.
- Large v waves are seen in mitral regurgitation, congestive heart failure, and ventricular septal defect due to the increase in atrial pressure.
Recognizing these patterns may suggest a diagnosis before a confirmatory echocardiogram is obtained.
The pressures observed in the right atrium range from 0 to 8 mmHg. Higher pressures may not necessarily mean fluid overload, but may reflect the volume of the right heart and the ability of the ventricle to eject that volume. There is little relationship between CVP and PAOP or left heart pressures in patients with valvular or coronary artery disease or when PAPs are elevated (40,41). Monitoring only the CVP can be misleading in patients with right heart failure, severe pulmonary disease, and most critically ill patients.
The next waveform seen is that of the RV (Fig. 21.1). The pressures here are higher with a wider difference between systolic and diastolic. If no RV waveform is seen after inserting the catheter 30 cm from the internal jugular or subclavian vein entry site, the catheter may be curling in the atrium or passing into the inferior vena cava. The catheter should be expeditiously advanced through the ventricle both to avoid dysrhythmias and to keep the catheter from warming and softening. The RV systolic pressures generally range from 15 to 30 mmHg and diastolic pressures from 4 to 12 mmHg. In right heart failure, the RV diastolic pressures may be high enough that the waveform mimics the PA. Low RV pressures will be seen in hypovolemic shock and they will also be close to PA pressures. One concern at this point of insertion is causing a right bundle branch block (RBBB), or even complete heart block in patients with pre-existing left bundle branch block (LBBB) (42). However, the incidence of complete heart block appears to be no greater in patients with LBBB than without (43).

FIGURE 21.8 Reading of central venous pressure (CVP) and pulmonary artery occlusion pressure (PAOP) in relationship to the cardiac cycle (electrocardiogram).
Once the catheter enters the pulmonary artery, the waveform shows an increase in diastolic pressure while the systolic pressure remains similar to the ventricle, sometimes referred to as the “step up” (Fig. 21.1). This transition may be difficult to discern when there is hypovolemia, tamponade, RV failure, or catheter whip. If there is no change in waveform after inserting the catheter 50 cm, it may be coiling in the ventricle and is at risk of knotting. A chest radiograph will discern the problem and fluoroscopy may be used to guide placement. Normal PA pressures range from 15 to 25 mmHg systolic over 8 to 15 mmHg diastolic. The beginning of diastole is marked by a dicrotic notch on the PA tracing, corresponding to the closure of the pulmonic valve (44). This incisura distinguishes the PA from the RV when RV diastolic pressures are elevated. As blood flows through the lungs to the left atrium, the PA pressure drops until it reaches a nadir at the end of diastole. Since the pulmonary circulation has low resistance, the diastolic pressure is able to decrease until it is just higher than PAOP. The highest PA systolic pressure occurs during the T wave of the corresponding ECG. The pulmonary circulation is very dynamic and is affected by acidosis, hypoxia, sepsis, and vasoactive drugs (38). An increase in CO may also paradoxically lower the PA pressures by a reflexive decrease in pulmonary vascular resistance (PVR) with fluid resuscitation and decreased sympathetic nervous system discharge (45).
The transition to the wedge position is noted by a drop in mean pressure from the PA. The PAOP usually ranges from 6 to 12 mmHg in normal states. PAOP most closely reflects LVEDP after atrial contraction and before ventricular contraction (Fig. 21.8). There are often no clear “a,” “c,” or “v” waves. The point 0.05 seconds after onset of QRS of the ECG is where the pressure best estimates LVEDP (46). When “v” waves are prominent such as in mitral insufficiency, the bottom of the “v” wave or the “a” wave may be used to measure the PAOP (Fig. 21.9). A prominent “v” wave may fool the novice into thinking that the catheter is not wedging. It is important to note the change in waveform from PA to v-wave tracing (although the two waves may look remarkably similar). One way of differentiation is that the “v” wave occurs later in the ECG cycle after the T wave while the PA wave occurs right after QRS (Fig. 21.9). There may be large “a” waves secondary to a decrease in left ventricle compliance (47); the point 0.05 seconds after initiation of QRS again best reflects LVEDP. Even though the measurements are correlated with the ECG and are done during end expiration, the PAOP may be exaggerated by respiratory muscle activity, especially during active or labored exhalation. Once the patient is adequately sedated, a short-acting paralyzing agent may be necessary to eliminate this effect (48) (Fig. 21.6).
Principles of Measuring Pulmonary Artery Occlusion Pressure
When the balloon is inflated, the blood flow in that segment of the pulmonary artery is occluded and the PAOP is measured. The pressure between the occluded pulmonary artery segment and the left atrium will equalize upon flow cessation (Fig. 21.10) which is analogous to closing off a pipe with pressures equalizing between the two ends (49). With the closed pipe analogy, there is a host of assumptions: PAOP ≅ PcP ≅ LAP ≅ LVEDP ≅ LVEDV, where PcP is pulmonary capillary pressure, LAP is left atrial pressure, and LVEDV is left ventricular end-diastolic volume. As long as there is no obstruction in this conduit, the relationship between PAOP and LVEDP may hold. The final assumption is equating pressure to volume by estimating LVEDV or “preload” with LVEDP. We will now assess the pitfalls with each of these assumptions.
- PAOP ≅ PcP ≅ LAP. In the “closed pipe” analogy, the column of blood between the catheter tip and the left atrium should be patent and not narrowed by alveolar pressures. This occurs in the dependent areas of the lung, where the pressures from blood flow in the right atrium and pulmonary artery are greater than the alveolar pressure, or zone 3 in the West classification (50). In other areas of the lung (zone 1 or 2), the pulmonary arteries may collapse from higher alveolar pressures and the wedge pressure may partially reflect alveolar pressure (Fig. 21.11). Because the PAC is directed by blood flow, it is more likely to pass into zone 3, where pulmonary arterial and venous pressures exceed alveolar pressures. This is especially true when the patient is supine, since there is greater volume of lung located in a dependent position (51). When pulmonary artery diastolic (PAD) pressure is lower than the PAOP, this implies incorrect positioning of the PAC (i.e., blood cannot flow in reverse direction), and may be due to transmission of alveolar pressures on the PAOP in non–zone 3 catheter position. Other factors that may cause errors in estimation of PAOP to LAP are pulmonary venous obstruction and respiratory variation as well as high ventilator support (PAOP reads higher than LAP).

FIGURE 21.9 Regurgitant mitral valve generating a v wave seen during wedging. PA, pulmonary artery.

FIGURE 21.10 Closed pipe analogy: blocking of flow by the balloon with theoretical equalization of pressure in the conduit. RA, right atrium; RV, right ventricle; PAOP, pulmonary artery occlusion pressure; Pc, pulmonary capillary; LAP, left atrial pressure; LA, left atrium; LV, left ventricle.
The PAOP usually closely approximates the pulmonary capillary hydrostatic pressure (Pc). When there is an increase in PVR, the wedge pressure underestimates Pc. A difference of 2 to 3 mmHg between the PAOP and PAD pressure is a clue that there may be a discrepancy between PAOP and Pc (34). Hydrostatic pulmonary edema may therefore occur at lower wedge pressures. A method of calculating the Pc has been described by recording the rapid drop in pressure decline when the catheter balloon is inflated in the wedge position (52). The point where the rapid decline transitions to a more gradual slope before reaching the PAOP is the Pc (Fig. 21.12).
Increased intrathoracic pressure secondary to respiratory failure and the addition of PEEP in ventilated patients affects pulmonary vascular pressures. Up to about 15 cm H2O, PAOP closely correlates with LAP (53). During higher PEEP states, the PAOP may not reflect the true filling pressure of the heart (i.e., pressure outside minus pressure inside the heart). Although the heart is seeing the high PEEP support at all times, on-PEEP PAOP is not giving the information that we need from the PAOP, which is the cardiac filling pressure. In general, 5 cm H2O of PEEP is said to raise the measured PAOP by 1 mmHg, but a greater effect is seen in hypovolemic patients or when the catheter is not in West zone 3 (54). High PEEP may also turn zone 3 status to zone 2 or 1 by compressing the pulmonary artery and/or pulmonary vein. Another formula predicts that 50% of PEEP is transmitted to the pleural space (51). However, in noncompliant lungs, such as in the acute respiratory distress syndrome, the alveolar pressure is not effectively transmitted to the vasculature. Also, pulmonary disease is not homogeneous. In complicated cases, it is best to avoid formulas or assumptions. In order to more accurately correct for the effect of pressure transmitted during high PEEP ventilation, the intrapleural pressure may be measured directly with a catheter in the pleural space or distal esophagus and then subtracted from the PAOP. However, this is cumbersome and not often done.

FIGURE 21.11 West lung zones. Zone 1: PAP < PalvP > PvP (there is no blood flow across the collapsed pulmonary capillary bed). Zone 2: PAP > PalvP > PvP (there is some flow since PAP is greater than PalvP). Zone 3: PAP > PalvP < PvP (pulmonary arteries are patent). PAP, pulmonary arterial pressure; PalvP, pulmonary alveolar pressure; PvP, pulmonary venous pressure.

FIGURE 21.12 Estimation of pulmonary capillary pressure. PA, pulmonary artery pressure; Pc, pulmonary capillary pressure; PAOP, pulmonary artery occlusion pressure.
Another method is to measure an “off-PEEP” wedge pressure by temporarily disconnecting the patient from the ventilator circuit and recording the nadir of the tracing (55). This nadir pressure better reflects LAP than the on-PEEP PAOP. The discontinuation should be brief (<1 second) so that a decrease in PaO2 from derecruitment of alveoli does not occur (56). The brief off-PEEP state will not change physiologic conditions such as venous return and cardiac function. The procedure should be coordinated and done by trained personnel only when the PAOP is needed to make a clinical decision. The balloon is inflated first to ensure good position, then deflated. The FiO2 may be increased temporarily for patient safety, the balloon reinflated, and at end expiration, the patient is disconnected from the ventilator for 1 second and then reconnected while the PAOP tracing is being recorded. The drop in PAOP upon ventilator disconnection is the off-PEEP PAOP (55). When done properly, it is extremely rare to cause hypoxia.
- LAP ≅ LVEDP. LAP (and thus PAOP) will overestimate LVEDP if there is an obstruction between the left atrium and the left ventricle such as a myxoma or mitral stenosis. Mitral valve regurgitation also causes the PAOP to read higher than the true LVEDP because of the additional pressure of the retrograde flow of blood across the valve resulting in a large v wave (see Pressure Measurements Section). LAP (and thus PAOP) will underestimate LVEDP when severe aortic regurgitation causes premature closure of the mitral valve when the left ventricle is still filling. LAP (and PAOP) is higher when there is a left atrial kick in a failing heart and decreased ventricle compliance such as in ischemic states, left ventricular hypertrophy, and restrictive cardiomyopathies (57). This is especially true when LVEDP is greater than 25 mmHg.
- LVEDP ≅ LVEDV. The pressure–volume relationship depends on the compliance of the ventricle and the transmural ventricular distending force. The compliance of the ventricles will change with ischemia, infarct, and hypertrophy. A stiff heart (myocardial hypertrophy) will need higher pressures to obtain the same amount of volume as a normal heart (Fig. 21.13). The transmural ventricular distending force (intracavitary pressure minus juxtacardiac pressure) will depend on the pressure inside and outside the heart. External forces elevating juxtacardiac pressures may be high ventilator support or pericardial tamponade, which may cause elevation of PAOP but may not reflect ventricular filling pressure.

FIGURE 21.13 The same pulmonary artery occlusion pressure of 20 mmHg reflecting three different clinical conditions. A: Distended hypervolemic ventricle in a normal heart. B: Normal volume in a noncompliant heart (ventricular hypertrophy). C: Low volume in a normal ventricle with high juxtacardiac pressures such as high positive end-expiratory pressure.
Clinical Use of the Pulmonary Artery Occlusion Pressure
As long as the previously mentioned assumptions regarding the relationship between PAOP, LAP, and LVEDP have been evaluated, the PAOP may be used as an estimate of LAP with reasonable correlation (49,53). The optimum wedge pressure depends on the patient, but has been defined as the pressure where there is minimal increase in stroke volume or left ventricular stroke work. Although the normal PAOP values may be 10 to 14 mmHg (58,59), some patients require a high pressure to reach the optimum stroke volume (Fig. 21.14). Such optimization can be graphically illustrated using a Starling curve plotting SVI to PAOP (as an estimate of LVEDP). If vasoactive agents are started, the heart may now be on a different curve requiring new assessment of the optimum PAOP. The optimum PAOP varies not only from patient to patient, but also temporally within the same patient as the clinical condition changes (such as vasoactive agents, myocardial compliance, and external forces around the heart). No set of values is broadly appropriate for all patients; in practice, each patient must be assessed repeatedly, further stressing the importance of judgment and expertise of the end user.

FIGURE 21.14 Frank–Starling curves (family of curves) showing the relationship between left ventricular end-diastolic pressure (LVEDP) and stroke volume (SV, mL/beat). Augmenting preload increases LVEDP with a concomitant increase in SV (up to a certain point). The effects of manipulating preload, afterload, and contractility and shifting to another curve can be seen.
Elevated wedge pressures may help differentiate hydrostatic pulmonary edema from that caused by increased permeability. A PAOP of 24 mmHg or higher is associated with a tendency for hydrostatic edema (60). Lower wedge pressures may imply increase capillary permeability and traditionally, a PAOP of <18 mmHg has implied a pulmonary (or noncardiogenic) cause of lung edema. When there is an increase in PVR, the wedge pressure underestimates Pc and hydrostatic pulmonary edema may therefore occur at lower wedge pressures (see Fig. 21.12).
Volumetric PACs are designed with the ability to measure right ventricular ejection fraction (RVEF), from which the right ventricular end-diastolic volume indexed (RVEDVI) to body surface area (BSA) is calculated. Traditionally, the right heart function was deemed unimportant and thought to merely act as a conduit to funnel blood to the left ventricle. However, right heart dysfunction with septal deviation may impact LV compliance and contractility, and the function of RV is important when PAPs are elevated. The volumetric PACs have two additional electrodes that provide continuous measurement of the ECG and a thermistor with a rapid response. From beat-to-beat change in temperature, the ejection fraction (EF) is calculated. EF (%) = SVI/EDVI × 100, where SVI is stroke volume indexed and EDVI is end-diastolic volume indexed to BSA. CI/HR = EDVI – ESVI, where ESVI is end-systolic volume indexed to BSA (61). RVEDVI has been shown to be a more accurate measure of cardiac preload than pressure measurements in certain patient populations (62,63). The measurement of RVEF has been validated by comparisons with transesophageal echocardiography (64). The RVEDVI in healthy individuals falls between 60 and 100 mL/m2. The information obtained from the volumetric catheter has been used to predict response to fluid challenge when the values are relatively low (<90 mL/m2) (62). Much like other measurements of fluid responsiveness, the validity of the RVEF measurement is compromised by tachycardia (pulse >120 beats/min) and atrial fibrillation (irregular HR) (65).
CARDIAC OUTPUT
One’s ability to meet increasing tissue oxygen demand by improving cardiac function is perhaps the single most important determinant in DO2 and tissue perfusion. The evolution of CO measurement is fascinating in its simplicity and started with Adolf Fick, who in the 1870s proposed that uptake or release of a substance by an organ is the product of blood flow through that organ and the difference between arterial and venous values of that substance. The original “dye” was oxygen and the organ studied was the lung. Fick’s equation stated: CO = VO2/(CaO2 – CvO2), where VO2 is oxygen consumption, CaO2 is arterial content of O2, and CvO2 is mixed venous content of O2.
This principle is widely accepted as an accurate though invasive assessment of CO since a PAC must be placed to obtain accurate mixed venous oxygen content. Additionally, its practical use is limited by the cumbersome measurement of VO2. Stewart (1897) and Hamilton (1932) utilized Fick’s principles but used a known amount of dye injected into central circulation followed by serial peripheral arterial measurements of dye concentration (i.e., change in dye concentration over time), and calculated the flow. The area under the curve after plotting time (x axis) versus dye concentration (y axis) reflected the CO using the following equation: Cardiac output = Amount of dye injected/integral (dye concentration × function of time). The next revolutionary step in CO measurement was using temperature as the dye. Crystalloid solution (usually 10 mL, but 5 mL may be used in volume-restricted patients) is injected into the RA port at similar parts of the respiratory cycle (end expiration), within 4 seconds in a smooth manner (66). The thermistor near the tip of the PAC detects the change in temperature, and the change in blood temperature over time is proportional to the blood flow from the ventricle. Several measurements (three to five) should be taken and the average of the values (within 10% of each other) used. Principles of the modified Stewart–Hamilton equation calculate the CO (Fig. 21.15).

FIGURE 21.15 The modified Stewart–Hamilton equation for estimating cardiac output.
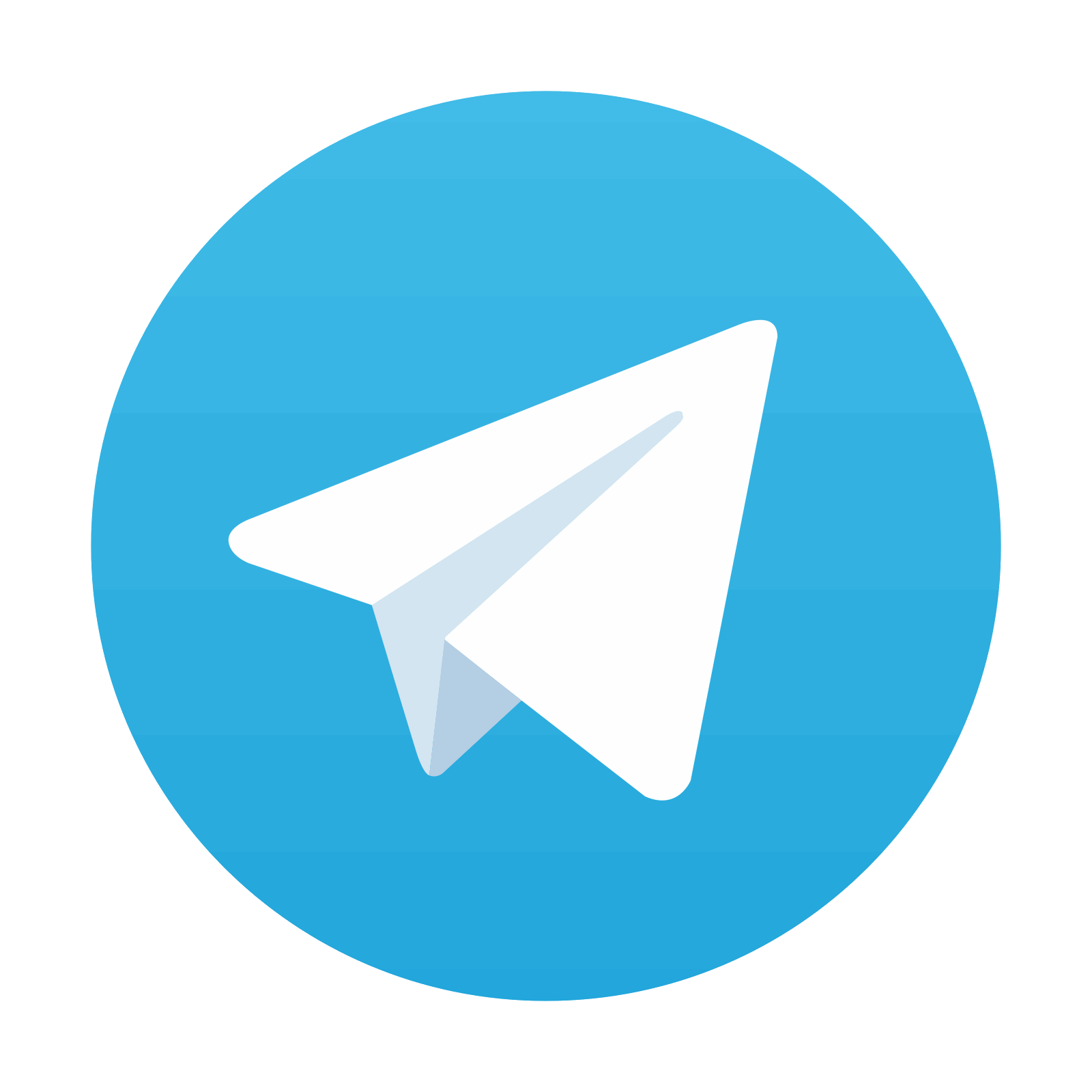
Stay updated, free articles. Join our Telegram channel

Full access? Get Clinical Tree
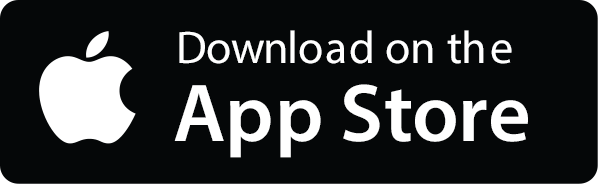
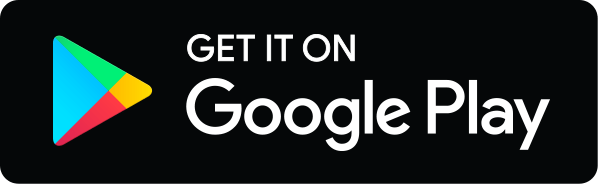