Jared Sheppard, MD, Christopher S. Nelson, MD, and Stephen L. Barnes, MD Division of Acute Care Surgery, Department of Surgery, University of Missouri, Columbia, MO, USA Which of the above values is most concerning for shock in this patient? The patient above clearly has significant blood loss from his GSW, such that he is in physiologic shock as a result of inadequate tissue perfusion. This can cause decreased GCS, and is consistent with his hypotension and tachycardia. Lactic acid is normally produced in excess by about 20 mmol/kg/day, which enters the bloodstream. It is then metabolized mostly by the liver and the kidney. Some tissues can use lactate as a substrate and oxidize it to carbon dioxide (CO2) and water, but only the liver and kidney have the necessary enzymes to utilize lactate for the process of gluconeogenesis. In general, elevated lactate can be the result of increased production, decreased clearance, or both. The tissues which normally produce excess lactic acid include the skin, red cells, brain tissue, muscle, and the gastrointestinal (GI) tract. During heavy exercise, it is the skeletal muscles which produce the most excess circulating lactate, which normalizes in the absence of impaired hepatic metabolism. Inadequate perfusion causes inadequate oxygen delivery to the tissue beds, which prevents normal aerobic respiration on the cellular level. As a result, anaerobic respiration begins, which results in net 2ATP per molecule of glucose (compared with 32 net ATP with aerobic respiration), and produces lactate as a byproduct. This elevation in lactate is responsible for the changes in pH and subsequent base deficit exhibited by this patient. Shock and severe lactic acidosis (pH less than 7.2) are often comorbid, and this carries a mortality rate of about 50%. Survival is rare for severe lactic acidosis with shock when the pH had fallen under 7.0. The base deficit is also an indicator of severe shock; however, this choice is base excess. Answer: E Mayer K, Trzeciak S, Puri NK. Assessment of the adequacy of oxygen delivery. Curr Opin Crit Care. 2016; 22(5):437–43. doi: 10.1097/MCC.0000000000000336. PMID: 27467272. Bonanno FG. Hemorrhagic shock: The “physiology approach”. J Emerg Trauma Shock. 2012; 5(4):285–95. doi: 10.4103/0974‐2700.102357. PMID: 23248495; PMCID: PMC3519039. Tissues attempt to extract from blood the amount of oxygen required to maintain aerobic metabolism, thus mixed‐venous O2 tension falls when O2 delivery (the product of cardiac output and arterial O2 content) becomes insufficient for tissue needs. As a primary determinant of O2 delivery, cardiac output measurements often prove helpful during selection of the appropriate ventilator settings (especially PEEP) for the patient with life‐threatening hypoxemia. Depression of venous return may nullify any beneficial effect of improved pulmonary gas exchange in tissue oxygen delivery. A rational goal of resuscitative therapy in severe sepsis and shock is to restore balance between O2 delivery and demand, and boosting cardiac output is fundamental to such an approach. Aggressive goal‐oriented resuscitation in the earliest phase of management appears to improve mortality in septic patients. The oxygen delivery equation (below) expresses oxygen delivery in terms of cardiac output multiplied by the oxygen‐carrying capacity of the blood. In order to calculate the oxygen delivery (DO2) of a patient, cardiac output, hemoglobin, SaO2, and PaO2 must be known. Answer: C Mayer K, Trzeciak S, Puri NK. Assessment of the adequacy of oxygen delivery. Curr Opin Crit Care. 2016; 22(5):437–43. doi: 10.1097/MCC.0000000000000336. PMID: 27467272. The oxygen delivery equation (below) is a function of cardiac output (HR × SV), Hgb concentration, percent hemoglobin saturation with oxygen measured by blood gas (SaO2), and percent of oxygen dissolved in the plasma itself, such that: However, this dissolved fraction of oxygen (PaO2) factors only minimally into the overall oxygen delivery formula, as denoted by the 0.003 coefficient (Choice D). As such, while the PaO2 is useful for determining various aspects of care (such as a P:F ratio in ARDS), it is essentially inconsequential in determining oxygen delivery. Typically, SaO2 (oxygen saturation from blood gas) is used to calculate CaO2. SPO2 is oxygen saturation by pulse oximeter. Regarding goals of oxygen delivery, an indexed DO2 (DO2 divided by body surface area, denoted as DO2i) of 400–600 mL/min/m2 has been shown to be associated with increased survival. The most effective way to increase oxygen delivery is by increasing hemoglobin. When going from a hemoglobin of 6–8 would result in an increase of oxygen delivery by 33%. Increasing preload may increase cardiac output by increasing stroke‐volume. It would be difficult to increase cardiac output easily by 33%. Increasing preload can often decrease PaO2 (Choice B). Increasing heart rate is typically not a strategy used to increase cardiac output (Choice C). Increasing PaO2 from 60 to 100 would only increase the saturation by 10% at the most as the saturation would only go from 90 to 100% (Choice E). Answer: A Lenkin AI, Kirov MY, Kuzkov VV, Paromov KV, Smetkin AA, Lie M, Bjertnæs LJ Comparison of goal‐directed hemodynamic optimization using pulmonary artery catheter and transpulmonary thermodilution in combined valve repair: a randomized clinical trial. Crit Care Res Pract. 2012; 2012:821218. doi: 10.1155/2012/821218. Mayer K, Trzeciak S, Puri NK. Assessment of the adequacy of oxygen delivery. Curr Opin Crit Care. 2016; 22(5):437–43. doi: 10.1097/MCC.0000000000000336. PMID: 27467272. Source: Data from: Mutschler A, Nienaber U, Brockamp T, et al. A critical reappraisal of the ATLS classification of hypovolaemic shock: does it really reflect clinical reality? Resuscitation 2013,84:309–313. The patient above has a narrowed pulse pressure (but is not yet hypotensive) and is tachycardic. He does not have any signs of overt shock (he is oriented and cooperative indicating adequate cerebral perfusion). Because of his tachycardia and narrowed pulse pressure with increased diastolic pressure, this would place him in class II shock, which is defined at 15–30% loss of total blood volume. By comparison, class I hemorrhagic shock is defined by less than 15% total blood volume loss, and typically does not have any significant vital sign abnormalities except for mild tachycardia. Class III hemorrhagic shock is achieved with 30–40% total blood volume loss, and will present with tachycardia, narrowed pulse pressure, hypotension, anxiety, and confusion. Therefore, a hypotensive patient from hemorrhagic shock has lost approximately 1.5–2 L of blood. Class IV hemorrhagic shock will have greater than 40% blood volume loss and will appear similar to class III but patients are typically lethargic instead of anxious. The astute clinician should recognize hemorrhagic shock long before the onset of hypotension, as by this point significant blood loss has already occurred. Instead, narrowed pulse pressure and tachycardia should be the first vital sign changes to point toward hemorrhage. Answer: B Bonanno FG. Hemorrhagic shock: The “physiology approach”. J Emerg Trauma Shock. 2012; 5(4):285–95. doi: 10.4103/0974‐2700.102357. PMID: 23248495; PMCID: PMC3519039. Table 6.1 Classes of hemorrhagic shock * Base excess is the quantity of base (HCO3−, in mEq/L) that is above or below the normal range in the body. A negative number is called a base deficit and indicates metabolic acidosis. Lawton LD, Roncal S, Leonard E, Stack A, Dinh MM, Byrne CM, Petchell J. The utility of Advanced Trauma Life Support (ATLS) clinical shock grading in assessment of trauma. Emerg Med J. 2014; 31(5):384–9. doi: 10.1136/emermed‐2012‐201813. Epub 2013 Mar 19. PMID: 23513233. Bedside pulse oximetry is a cornerstone of hemodynamic monitoring in the intensive care unit due to its noninvasive nature and accuracy. However, there are multiple situations in which readings can be erroneous. Once such example is sickle cell disease, which causes deformation of hemoglobin and decreases flow through the micro circulation, and thus causes an overestimation of readings. The clinical significance of these readings is often downplayed in studies. Acute blood loss anemia, by itself, seems to have no effect on oximetry readings (Choice A). Most peripheral sensors use two wavelengths of light: those associated with oxygenated and deoxygenated hemoglobin. These light waves are in the near‐infrared spectrum as these light waves can easily pass through bone and tissue. Thus, the light looks red as red light can passed through bone and tissue as well. The presence of carboxyhemoglobin and methemoglobin, which have differing specific wavelengths, causes an overestimation of oxygen saturation (Choice B). Cyanide toxicity would cause an inability to use oxygen as an electron acceptor in aerobic metabolism but would not change the SpO2 (Choice C). Motion artifact has long been a reason for inaccuracy with peripheral sensors, and despite several attempts at correction, this remains a common cause of alarm that is not associated with actual patient decline (Choice D). Other causes of pulse oximetry inaccuracy include methylene blue, indocyanine green, indigo carmine, and black, blue, or green nail polish (Choice E). Answer: B Chan ED, Chan MM, Chan MM. Pulse oximetry: understanding its basic principles facilitates appreciation of its limitations. Respir Med. 2013; 107(6):789–99. doi: 10.1016/j.rmed.2013.02.004. Epub 2013 Mar 13. PMID: 23490227. Stroke volume variability is a naturally occurring phenomenon in which the arterial pulse pressure falls during inspiration and rises during expiration due to changes in the intrathoracic pressure secondary to negative pressure ventilation (i.e., spontaneous breathing). Variations over 10 mmHg have been referred to as pulses paradoxus. Reverse pulsus paradoxus is a same phenomenon with controlled mechanical ventilation. Stroke volume variability (SVV) is calculated from the percent variability in stroke volume between inspiratory and expiratory portions of the ventilator cycle. The differences in intrathoracic pressure from the ventilator directly affect the amount of venous return to the right ventricle, which determines the subsequent stroke volume (increased intrathoracic pressure decreases the amount of venous return, subsequently decreasing the stroke volume). This effect is magnified in states of volume deficiency, as the lower circulating volume is more subjected to the changes in intrathoracic pressure. A SVV of >13% has been shown to correlate well to a deficit in overall intravascular volume, and thus predicts an increase in stroke volume in response to fluid administration. Stroke volume variability can be measured from the arterial line using a variety of monitoring devices. Answer: D Pinsky MR. Functional hemodynamic monitoring. Crit Care Clin. 2015; 31(1):89–111. doi: 10.1016/j.ccc.2014.08.005. PMID: 25435480; PMCID: PMC4250574. Shi R, Monnet X, Teboul JL. Parameters of fluid responsiveness. Curr Opin Crit Care. 2020; 26(3):319–26. doi: 10.1097/MCC.0000000000000723. PMID: 32332283. Aortic pulse pressure is directly proportional to left heart stroke volume and aortic compliance. The variation in the pulse pressure (pulse pressure variation or PPV) should thus reflect stoke volume changes as a result of the respiratory cycle. With more intravascular volume, the respiratory influence on preload should diminish, and the variation in pulse pressure should similarly decrease. Several studies showed that PPV accurately predicts fluid responsiveness when patients are under controlled mechanical ventilation. Nevertheless, in many conditions encountered in the ICU, the interpretation of PPV is unreliable (spontaneous breathing, cardiac arrhythmias) or doubtful (low VT). However, there are certain instances in which the respiratory cycle does not significantly alter preload. If the patient has ARDS, he should be on low tidal volume lung‐protective ventilation. Per ARDSNET protocol, a goal tidal volume of 6 mL/kg ideal body weight should be utilized in patients with ARDS. However, low tidal volumes have a less pronounced influence on preload, and several studies have shown that tidal volumes of at least 8 mL/kg are necessary for a reliable variation due to hypovolemia (Choice A). Atrial fibrillation (Choice B) likewise will alter pulse pressure due to changes in length between beats, thus changing the amount of time available for atrial/ventricular filling. This will result in intrinsic pulse pressure variation independent of volume status. In true abdominal compartment syndrome (Choice C), the increased abdominal pressure will prevent an adequate respiratory cycle, and similar to a patient with a set low tidal volume, this will result in significantly decreased effect on preload variation from hypovolemia. Increased respiratory rates (Choice D) can result in low PPV even in cases of sufficient intravascular volume, and thus PPV is not reliable when the heart rate/respiratory rate ratio is less than 3.6. The final patient (Choice E) may have altered hemodynamics secondary to neurogenic shock; however, this will not change the effect of respiratory cycle on ventricular filling. In medical critically ill patients, although no randomized controlled trial has compared PPV‐based fluid management with standard care, the Surviving Sepsis Campaign guidelines recommend using fluid responsiveness indices, including PPV, whenever applicable. In conclusion, PPV is useful for managing fluid therapy under specific conditions where it is reliable. The kinetics of PPV during diagnostic or therapeutic tests is also helpful for fluid management. Answer: E Yang X, Du B. Does pulse pressure variation predict fluid responsiveness in critically ill patients? A systematic review and meta‐analysis. Crit Care. 2014; 18(6):650. doi: 10.1186/s13054‐014‐0650‐6. PMID: 25427970; PMCID: PMC4258282. Teboul JL, Monet X, Chemla D, Michard F. Arterial pulse pressure variation with mechanical ventilation. Am J Res Crit Care Med. 2019; 199(1):22–32. doi: 10.1164/rccm.201801‐0088CI. While IVC collapsibility or distensibility throughout the respiratory cycle (caval index) has been used for years as an adjunct to determine volume responsiveness in critically ill patients, the practice has demonstrated fairly poor reliability. In a systematic review and meta‐analysis performed by Orso et al., 26 studies were evaluated that investigated the predictiveness of the caval index in both adult and pediatric intubated and non‐intubated patients. While the caval index in intubated patients was more predictive than in non‐intubated patients, there was not sufficient correlation among studies to show that IVC diameter throughout the respiratory cycle was a reliable method of predicting volume responsiveness. Answer: E Long E, Oakley E, Duke T, Babl FE ; Paediatric Research in Emergency Departments International Collaborative (PREDICT). Does respiratory variation in inferior vena cava diameter predict fluid responsiveness: A systematic review and meta‐analysis. Shock. 2017; 47(5):550–9. Orso D, Paoli I, Piani T, Cilenti FL, Cristiani L, Guglielmo N. Accuracy of ultrasonographic measurements of inferior vena cava to determine fluid responsiveness: A systematic review and meta‐analysis. J Intensive Care Med. 2020; 35(4):354–363. doi: 10.1177/0885066617752308. Epub 2018 Jan 17. PMID: 29343170. An end‐expiratory occlusion test is done by increasing intrathoracic pressure during inspiration while on mechanical ventilation. The ventilation is stopped at end‐expiration, at the level of positive end‐expiratory pressure (PEEP). If done long enough, this increase in pressure results in decreased preload to the left ventricle and subsequently a reduced cardiac output. By performing end‐expiratory occlusion (pausing mechanical ventilation at end‐expiration for 15 seconds), the artificially reduced preload is eliminated, and so a preload increase is simulated. If there is significant change in stroke volume on bedside monitoring with inspiratory hold, this predicts that fluid administration would also increase stroke volume. A 2009 study by Monnet et al. evaluated EEO, passive leg raise, and a 500cc fluid bolus administration, and found that an increase in arterial pulse pressure > 5% during EEO was predictive of an increase in CO following volume administration. The other maneuvers listed would all decrease the left‐sided preload and have the opposite effect. Answer: A Jalil BA, Cavallazzi R. Predicting fluid responsiveness: A review of literature and a guide for the clinician. Am J Emerg Med. 2018; 36(11):2093–2102. doi: 10.1016/j.ajem.2018.08.037. Epub 2018 Aug 14. PMID: 30122506. Monnet X, Osman D, Ridel C, Lamia B, Richard C, Teboul JL. Predicting volume responsiveness by using the end‐expiratory occlusion in mechanically ventilated intensive care unit patients. Crit Care Med. 2009; 37(3):951–6. doi: 10.1097/CCM.0b013e3181968fe1. PMID: 19237902. Although routinely used, traditional methods for confirming the correct positioning of an endotracheal tube have limited reliability. These include stethoscopic audibility and symmetry of breath sounds and chest rise, direct visualization of the cords, ease of insufflation and recovery of tidal volume, tidal fogging and clearing of the endotracheal tube, palpation of the tube in the larynx, loss of voice, coughing and expulsion of airway secretions (Choice C), expansion of upper chest, and failure of the abdomen to progressively distend during gas delivery. To improve reliability and speed of placement, the physical detection of CO2 during expiration by capnography and capnometry can be used (Choices A, E). CO2 detection and measurement by these methods can occasionally and transiently be misleading. Minimal CO2 is evolved or expelled during shock or circulatory arrest and some CO2 may be liberated initially after esophageal intubation from gas trapped in the gastric pouch. However, this concentration falls rapidly as serial tidal volumes are delivered. When compressed, a large‐capacity squeeze bulb affixed to the endotracheal tube will fail to fill easily if the tube is in the collapsible esophagus. Several studies have also established the efficacy of confirming endotracheal tube placement by direct trans‐tracheal visualization with bedside ultrasound (Choice B). Although not always easily available, flexible bronchoscopy with visualization of the trachea would be confirmatory (Choice D). Answer: C Gottlieb M, Holladay D, Peksa GD. Ultrasonography for the confirmation of endotracheal tube intubation: A systematic review and meta‐analysis. Ann Emerg Med. 2018; 72(6):627–36. doi: 10.1016/j.annemergmed.2018.06.024. Epub 2018 Aug 14. PMID: 30119943. Littlewood K, Durbin CG Jr. Evidenced‐based airway management. Respir Care. 2001; 46(12):1392–405; discussion 1406‐7. PMID: 11728299. When the catheter is inserted via the right internal jugular vein, the balloon is inflated 15 cm from the point of neck entry. From the RIJ approach, the RA is entered at approximately 25 cm, the RV at approximately 30 cm, and the PA at approximately 40 cm; the PCWP can be identified at approximately 45 cm. As a rule of thumb, the catheter tip should not require advancement of more than 20 cm beyond its current position before encountering the next vascular compartment. Coiling within the right ventricle and misdirection of the catheter should be suspected after reaching 45–50 cm and the appropriate PA waveform is not encountered. Fluoroscopy can be a helpful adjunct in difficult cases and is especially worthwhile to consider before attempting an insertion from the femoral site. When advancing the catheter from the RA to the RV, the systolic pressure will climb significantly owing to a functional tricuspid valve. During diastole, the valve is open, allowing for the diastolic pressure to remain the same between the RA and RV. Answer: B Whitener S, Konoske R, Mark JB. Pulmonary artery catheter. Best Pract Res Clin Anaesthesiol 2014; 28(4):323–35. doi: 10.1016/j.bpa.2014.08.003. Epub 2014 Sep 8. PMID: 25480764. Scheeren TWL, Ramsay MAE. New developments in hemodynamic monitoring. J Cardiothorac Vasc Anesth. 2019; 33(Suppl 1):S67–72. doi: 10.1053/j.jvca.2019.03.043. PMID: 31279355. Thermodilution is an indicator‐dilution method of measuring blood flow. This method is based on the premise that, when an indicator substance is added to circulating blood, the rate of blood flow is inversely proportional to the change in concentration of the indicator over time. This can be accomplished by using a temperature change as an indicator. Using a pulmonary artery catheter, cold fluid is mixed in the right heart chambers and the cooled blood is ejected into the pulmonary artery and flows past the thermistor on the distal end of the catheter. The thermistor records the change in blood temperature over time. This information is sent to an electronic device that records and displays a temperature time curve. The area under the curve is inversely proportional to the rate of blood flow in the pulmonary artery, and this flow is equivalent to the cardiac output. Tricuspid regurgitation causes the cold indicator fluid to be recycled back and forth across the tricuspid valve (Choice E). This produced a prolonged, low‐amplitude thermodilution curve, thus tricuspid regurgitation produces a falsely low thermodilution cardiac output. Aortic regurgitation, aortic stenosis, and mitral prolapse are left‐sided heart abnormalities that can affect cardiac output but will not affect the thermodilutional method (Choices A, B, C). Similarly, tricuspid stenosis will still allow a steady rate of flow across the valve and will not affect thermodilution (Choice D). Answer: E Scheeren TWL, Ramsay MAE. New developments in hemodynamic monitoring. J Cardiothorac Vasc Anesth. 2019; 33(Suppl 1):S67–72. doi: 10.1053/j.jvca.2019.03.043. PMID: 31279355. Kobe J, Mishra N, Arya VK, Al‐Moustadi W, Nates W, Kumar B. Cardiac output monitoring: Technology and choice. Ann Card Anaesth. 2019; 22(1):6–17. doi: 10.4103/aca.ACA_41_18. PMID: 30648673; PMCID: PMC6350438. Central line‐associated blood stream infections (CLABSI) are a major risk of an indwelling central venous access, and major progress has been made with systemic guidelines implemented in most hospital systems to reduce their incidence. Placement of central lines in the subclavian vein has shown decreased rates of infection; however, other locations may be necessary for placement based on the patient’s anatomy or injury pattern (Choice A). An increased number of passes before successful cannulation of the vein has been associated with increased risk of infection, and for this reason, ultrasound is recommended to achieve first‐attempt vascular access (Choice B). In addition, ultrasound in real time use during the procedure has become the standard of care to decrease complications. While theoretical risk of infection increases with the number of lumens in a central line, this has not been borne out in literature (Choice C). Chlorhexidine‐impregnated dressings significantly decrease the rate of infection, as do daily chlorhexidine baths of the line. While using a suture to secure a central line provides a more stable anchor, administering another wound (albeit small) to the skin from the needle also increases the risk of surrounding infection (Choice E). Answer: D Bell T, O’Grady NP. Prevention of central line‐associated bloodstream infections. Infect Dis Clin North Am. 2017; 31(3):551–9. doi: 10.1016/j.idc.2017.05.007. Epub 2017 Jul 5. PMID: 28687213; PMCID: PMC5666696. Parienti JJ, Mongardon N, Mégarbane B, Mira JP, Kalfon P, Gros A, Marqué S, Thuong M, Pottier V, Ramakers M, Savary B, Seguin A, Valette X, Terzi N, Sauneuf B, Cattoir V, Mermel LA, du Cheyron D ; 3SITES Study Group. Intravascular complications of central venous catheterization by insertion site. N Engl J Med. 2015; 373(13):1220–9. doi: 10.1056/NEJMoa1500964. PMID: 26398070. Pathogen distribution and antimicrobial resistance, as reported to the National Healthcare Safety Network from 2011‐2014 showed that among central line‐associated blood stream infection (CLABSI), the most common causative pathogens were (in order): coagulase‐negative staphylococci (20.9%), staphylococcus aureus (18.1%), Klebsiella spp (9.4%), Enterococcus faecalis (9.1%), Escherichia coli (7.4%), Enterobacter spp (5.5%), and Pseudomonas (3.4%). Multiple strategies have been studied and implemented to reduce the incidence of CLABSI, including antiseptic and antibiotic coating of the central lines; antiseptic/antibiotic impregnated catheters have been shown to result in a 2% absolute risk reduction of CLABSI. Another pillar of CLABSI prevention is catheter removal as soon as the catheter is no longer needed, as well as catheter exchange when there is clinical concern of bloodstream infection. Prophylactic or scheduled catheter exchange versus removal when clinically indicated showed no difference in CLABSI rates, although procedure‐related complications of catheter placement were more frequent in patients undergoing scheduled exchange. Answer: B Weiner‐Lastinger LM, Abner S, Edwards JR, Kallen AJ, Karlsson M, Magill SS, Pollock D, See I, Soe MM, Walters MS, Dudeck MA. Antimicrobial‐resistant pathogens associated with adult healthcare‐associated infections: Summary of data reported to the National Healthcare Safety Network, 2015‐2017. Infect Control Hosp Epidemiol. 2020; 41(1):1–18. doi: 10.1017/ice.2019.296. Epub 2019 Nov 26. PMID: 31767041. Bell T, O’Grady NP. Prevention of central line‐associated bloodstream infections. Infect Dis Clin North Am. 2017; 31(3):551–9. doi: 10.1016/j.idc.2017.05.007. Epub 2017 Jul 5. PMID: 28687213; PMCID: PMC5666696. 15 and 16: A 78‐year‐old stunt pilot is involved in a training accident while attempting to land his plane. He sustains a large right subdural hematoma, right 1–9th rib fractures, left 2–7th rib fractures, bilateral pulmonary contusions, and a grade III liver laceration. Once in the ICU, a pulmonary artery catheter is placed, revealing the following values: MAP 60 mm Hg, PAP 40/20 mm Hg, PCWP 18 mm Hg, CVP 10 mm Hg, CO 4 L/min. A major component of afterload is the resistance to ventricular outflow in the aorta and large, proximal arteries. The total hydraulic force that opposes pulsation flow is known as impedance. This force is a combination of 2 forces: a force that opposes the rate of change in flow (compliance), and a force that opposes mean or volumetric flow (resistance). Vascular resistance is derived by assuming that hydraulic resistance is analogous to electrical resistance. Ohm’s law predicts that resistance to flow of an electric current (R) is directly proportional to the voltage drop across a circuit (V), and inversely proportional to the flow of current (I): R = V/I. This relationship is applied to the systemic and pulmonary circulations, creating the following derivations:
6
Hemodynamic and Respiratory Monitoring
Parameter
Class I
Class II (mild)
Class III (moderate)
Class IV (severe)
Approximate blood loss
<15%
15–30%
31–40%
>40%
Heart rate
↔
↔/↑
↑
↑/↑↑
Blood pressure
↔
↔
↔/↓
↓
Pulse pressure
↔
↓
↓
↓
Respiratory rate
↔
↔
↔/↑
↑
Urine output
↔
↔
↓
↓↓
Glasgow Coma Scale score
↔
↔
↓
↓
Base deficit*
0 to −2 mEq/L
−2 to −6 mEq/L
−6 to −10 mEq/L
−10 mEq/L or less
Need for blood products
Monitor
Possible
Yes
Massive transfusion protocol
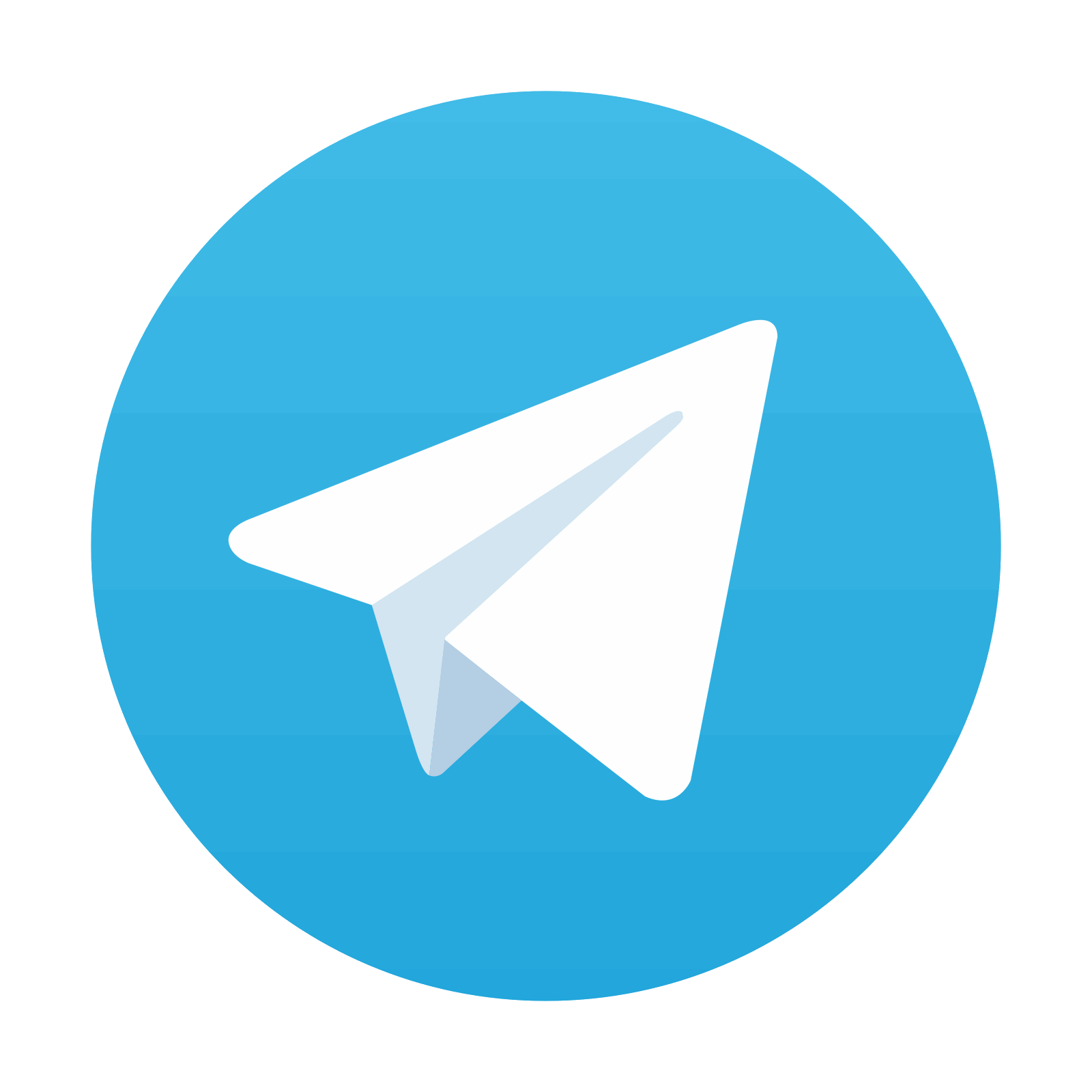
Stay updated, free articles. Join our Telegram channel

Full access? Get Clinical Tree
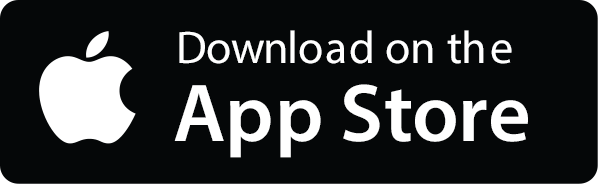
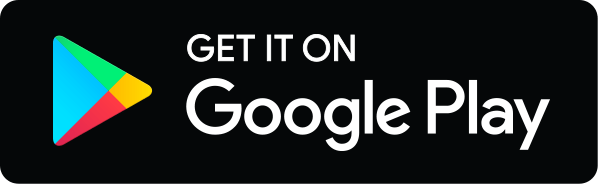