Hematopoietic Cell Transplantation
Paul A. Carpenter
Marco Mielcarek
Ann E. Woolfrey
General Principles
Hematopoietic cell transplantation (HCT) typically is performed in patients with life-threatening disorders of the hematopoietic system. The procedure has considerable risks of transplant-related morbidity and mortality with a substantial proportion of patients requiring intensive medical care [1,2] (Fig. 188.1). Thus, knowledge of the basic principles of the transplant procedure and an understanding of potential complications including their differential diagnosis are important for improving the outcome of critically ill patients after transplantation.
HCT is potentially curative treatment for diseases including leukemia, lymphoma, myelodysplasia, multiple myeloma, aplastic anemia, hemoglobinopathies, and congenital immune deficiencies. In selected cases, HCT may also have a role in the treatment of solid tumors such as germ cell tumors, renal cell cancer, and breast cancer, and as a type of immunosuppression for patients with life-threatening autoimmune diseases (Table 188.1). In preparation for HCT, high-dose chemotherapy alone, or combined with irradiation therapy, is used to eradicate the underlying disease and to induce transient immunosuppression in the recipient to prevent graft rejection, a possible complication mediated by immunologic host-versus-graft reactions after allogeneic HCT. High-dose chemoradiation is followed by intravenous infusion of the graft, which contains hematopoietic stem cells (HSCs) that home to the bone marrow and reconstitute the hematopoietic system of the patient. In contrast to autologous HCT, allogeneic HCT requires prophylactic immunosuppressive therapy after transplant to prevent or mitigate graft-versus-host disease (GVHD), an inflammatory syndrome that primarily affects the skin, gastrointestinal (GI) tract, and liver.
Classification
HCT can be categorized according to the source of stem cells, the type of donor, or the intensity of the preparative regimen. The type of HCT used in an individual patient is a complex decision based on the patient’s age, diagnosis, disease stage, prior treatments, donor availability, and presence of comorbidities.
Stem Cell Source
HSCs capable of reconstituting hematopoiesis in recipients given myeloablative therapy can be obtained from bone marrow, peripheral blood, or umbilical cord blood (UCB). The stem cell products obtained from each of these sources are characterized by distinct kinetics of engraftment and recovery of immune function after transplantation. These features may affect the risks of developing infectious complications and GVHD during the posttransplant period.
Bone Marrow
Bone marrow was historically the most common source of stem cells for HCT but is now used very infrequently for autologous HCT. Bone marrow is harvested from the iliac crest under general anesthesia, from appropriate volunteer donors. Engraftment after bone marrow transplant is evidenced by rising neutrophil and platelet counts and occurs between 3 and 4 weeks after transplant.
“Mobilized” Peripheral Blood
Growth factor–mobilized peripheral blood stem cells (PBSC) are the predominant source of HSC for allogeneic HCT in adults and are almost always used as HSC rescue for autologous HCT [3]. PBSCs are recognized on the basis of their expression of the CD34 surface marker and can be collected from the blood by a semiautomated procedure called leukapheresis. To promote peripheral blood mobilization of PBSC for autologous HCT, patients typically receive chemotherapy followed by administration of G-CSF, which has the benefit of chemotherapy-mediated tumor debulking prior to stem cell collection [4]. For allogeneic HCT, PBSCs are mobilized from healthy donors using growth factor alone.
Engraftment after PBSC transplantation occurs approximately 1 week earlier compared with bone marrow transplantation, which is likely related to the greater proliferative potential of stem and progenitor cells in PBSC. PBSC allografts contain approximately 10 times more T cells than marrow, which influences the development of GVHD, graft rejection, and rate of relapse for malignancies after HCT [5]. Randomized studies of allografts donated from HLA-matched siblings have shown a higher risk for relapse and lower risk for chronic GVHD among recipients of marrow compared with PBSC [3,6].
Umbilical Cord Blood
UCB contains HSC sufficient for reconstitution of hematopoiesis, which can be collected from the placenta and umbilical cord immediately after delivery of a baby. UCB banking has increased the likelihood of donor availability for patients with rare HLA haplotypes. T cells contained in UCB are immunologically naive, which allows for less stringent HLA matching between donor and recipient. The number of HSC contained in a typical UCB unit is several orders of
magnitude lower compared with typical bone marrow or PBSC harvests. The smaller number of HSC may result in delayed engraftment, increased risk for graft rejection, and infection [7,8]. Recent studies have shown that infusion of two UCB units increases the total number of HSC, which seems to decrease the risk of graft rejection, thus giving adults as well as children the option of UCB transplantation [9].
magnitude lower compared with typical bone marrow or PBSC harvests. The smaller number of HSC may result in delayed engraftment, increased risk for graft rejection, and infection [7,8]. Recent studies have shown that infusion of two UCB units increases the total number of HSC, which seems to decrease the risk of graft rejection, thus giving adults as well as children the option of UCB transplantation [9].
Table 188.1 Indications for Allogeneic or Autologous Transplants | ||||
---|---|---|---|---|
|
Donor Type
Autologous
Transplantation of HSC donated by the patient is termed autologous HCT. Most commonly, autologous PBSC are cryopreserved and then thawed and reinfused once the high-dose preparative therapy has been completed. High-dose chemoradiation is given to kill tumor cells that may not be susceptible to conventional-dose cytotoxic therapy. The success of the autologous transplant procedures relies exclusively on the tumor-eradicating potential of the preparative regimen [10]. The effect the conditioning regimen has on extrahematopoietic tissues determines the dose-limiting toxicity of the procedure. Relapse after autologous HCT may occur from tumor cells that have survived the conditioning therapy or from those that contaminated the graft, although the former mechanism appears to be more important.
Syngeneic
Transplantation of HSCs donated from identical (monozygotic) twins is termed syngeneic HCT. When there is no genetic disparity between donor and recipient, the biology of the transplant is similar to autologous HCT. Compared with allogeneic HCT from HLA-matched related or unrelated donors, relapse rates are higher after syngeneic HCT, which has been attributed to the absence of malignancy-eradicating graft-versus-host reactions.
Allogeneic
Transplantation of HSCs cells donated by another individual is termed allogeneic HCT. Allogeneic HCT requires
availability of an HLA-compatible related or unrelated donor. Because of the inheritance pattern of HLA haplotypes, the statistical likelihood of two siblings being genotypically HLA identical is 25%. Donor-recipient HLA genotypic identity is associated with the lowest risks for immunologically mediated complications such as graft rejection and GVHD [11]. For approximately 70% of patients who do not have an HLA-identical sibling donor, a search for a suitable unrelated donor can be considered. HCT from HLA-matched unrelated donors, however, has traditionally been associated with higher risks of transplant-related morbidity and mortality compared with HCT from HLA-identical related donors. Use of unrelated donors who are matched using molecular HLA typing methods can improve outcomes considerably, and, for some diseases, survival of patients with unrelated grafts has approached that with HLA-identical sibling grafts [12,13].
availability of an HLA-compatible related or unrelated donor. Because of the inheritance pattern of HLA haplotypes, the statistical likelihood of two siblings being genotypically HLA identical is 25%. Donor-recipient HLA genotypic identity is associated with the lowest risks for immunologically mediated complications such as graft rejection and GVHD [11]. For approximately 70% of patients who do not have an HLA-identical sibling donor, a search for a suitable unrelated donor can be considered. HCT from HLA-matched unrelated donors, however, has traditionally been associated with higher risks of transplant-related morbidity and mortality compared with HCT from HLA-identical related donors. Use of unrelated donors who are matched using molecular HLA typing methods can improve outcomes considerably, and, for some diseases, survival of patients with unrelated grafts has approached that with HLA-identical sibling grafts [12,13].
The worldwide development of donor registries has increased the number of available HLA-matched unrelated donors and umbilical cord blood units for patients without suitable related donors. Another alternative source of HSC is a haploidentical relative, such as a parent, defined by the inheritance of one identical haplotype and mismatching of one or more HLA loci with the noninherited haplotype. Over the past decade, technological advances have improved the outcome for recipients of HLA-disparate grafts. When more than a single HLA antigen disparity is present, depletion of T cells from the graft is necessary to prevent life-threatening GVHD. Depletion of T cells from the marrow may be accomplished ex vivo by using immunologic or physical methods to target T cells for removal. Because T cells play an important role in establishment of the graft, early immune reconstitution, and tumor control, T-cell depletion has been associated with higher rates of graft failure, opportunistic infections, and relapse. Strategies to selectively deplete alloreactive T cells remain an active area of research.
Intensity of the Preparative Regimen
Myeloablative
In myeloablative HCT, the preparative regimen ablates the hematopoietic system of the patient and leads to transient but profound myelosuppression with pancytopenia. The transplanted hematopoietic cells reconstitute the ablated hematopoietic system in the recipient. High-dose chemotherapy regimens, with or without doses of total body irradiation (TBI) that exceed 6 Gy, combine different drug combinations that have nonadditive toxicities with radiation. The aim of high-dose therapy is to overcome the genetic heterogeneity of tumors by employing agents with different mechanisms of action. Although the myeloablative regimens used for autologous HCT typically consist of drugs that provide maximum tumor eradication with tolerable toxicity to the patient, regimens used for allogeneic HCT also must provide sufficient recipient immunosuppression to prevent graft rejection. Myeloablative preparative regimens are associated with substantial risks of transplant-related toxicity and mortality, particularly among older or medically ill patients [14].
Nonmyeloablative
Nonmyeloablative preparative regimens for allogeneic HCT are mainly immunosuppressive and aimed at preventing graft rejection. The underlying malignancy is eliminated through the ensuing immunologic graft-versus-tumor effects, provided the tumor expresses antigens that make it a target for immune attack. Compared with myeloablative allogeneic HCT, the extrahematopoietic toxicity from nonmyeloablative preparative regimens is considerably milder, an important consideration for older patients or those with comorbidities [15,16]. Typical post-HCT complications such as GVHD and infections, however, are not prevented by nonmyeloablative conditioning but may have a delayed onset.
Epidemiology
Current estimates of annual numbers of HCT are 45,000 to 50,000 worldwide. During 2006, 16,000 transplants were registered with the Center for International Blood and Marrow Transplant Research (CIBMTR), of which one-half were allogeneic. Allogeneic HCT is most commonly performed in adults using PBSC grafts. In contrast, children now predominantly receive cord blood or marrow grafts (NMDP Web site: http://www.marrow.org/). PBSC is less used in children because of the difficulties harvesting PBSC from young children and because of the increased risk of chronic GVHD.
Risk Factors for Transplant-Related Morbidity and Mortality
The likelihood of developing transplant-related complications depends on patient’s age, the intensity of the preparative regimen, the type and stage of the underlying disease, and the presence of comorbidities. Prognosis is most heavily influenced by the underlying disorder. Patients with chronic malignancies and nonmalignant disorders, such as aplastic anemia, have a higher likelihood of survival compared to those with aggressive malignancies, who have a greater tendency to relapse following HCT. Mortality caused by the transplant procedure, and not from disease relapse, termed transplant-related mortality, ranges from 15% to 40% for allogeneic HCT recipients compared to 5% to 10% for autologous HCT recipients. HLA disparity between donor and recipient increases the risk of transplant-related mortality owing to the greater likelihood of developing GVHD and graft rejection. The risk for mortality increases significantly with age, although improvements in supportive care and donor selection and the introduction of nonmyeloablative preparative regimens have increased the proportion of patients older than 60 years who benefit from allogeneic HCT. Recent studies have demonstrated that pretransplant assessment of comorbidities using simple but transplant-specific comorbidity scoring systems has improved the ability to predict subsequent transplant-related mortality and survival [14,17].
Transplant-Related Complications
Transplanted-related complications include infections, regimen-related toxicity (RRT), and complications associated with alloreactivity. More intense conditioning regimens and higher degrees of donor-recipient HLA disparity are associated with greater risk for infection. Regimen-related toxicities include profound cytopenias and organ damage that follow myeloablative conditioning. The complications seen after allogeneic HCT that may occur irrespective of the intensity of the conditioning regimen include rejection, GVHD, and hemolysis.
Regimen-Related Pancytopenia
Reconstitution of hematopoiesis after HCT occurs in an orderly pattern; in general, neutrophil recovery occurs first, followed by recovery of platelets and red blood cells. The tempo of hematopoietic reconstitution varies according to the type
of HSC product, being earlier after PBSC grafts and later after UCB grafts, compared with marrow grafts. Transfusions of platelets and red blood cells often are needed until there is marrow recovery. Transfusion of red blood cells should be determined by the clinical condition of the patient, including hemodynamic stability and presence of active hemorrhage. Red blood cell transfusions generally are indicated when the hemoglobin falls below 8 g per dL. Platelet transfusions are indicated when the platelet count falls below 10,000 cells per μL to minimize the risk for spontaneous bleeding [18,19]. Transfusions thresholds should be increased before invasive procedures or in patients with bleeding to a level appropriate for any other intensive care unit (ICU) patient [18]. Platelet consumption may be increased in patients with fever, disseminated intravascular coagulation (DIC), or splenomegaly. Patients who have become alloimmunized to platelet antigens demonstrate poor response to platelet transfusions and may achieve higher platelet counts by limiting the number of donor exposures, controlling fever or DIC, using platelet products that are less than 48 hours old, or use of nonpooled (single-donor) or HLA-matched platelets [20,21].
of HSC product, being earlier after PBSC grafts and later after UCB grafts, compared with marrow grafts. Transfusions of platelets and red blood cells often are needed until there is marrow recovery. Transfusion of red blood cells should be determined by the clinical condition of the patient, including hemodynamic stability and presence of active hemorrhage. Red blood cell transfusions generally are indicated when the hemoglobin falls below 8 g per dL. Platelet transfusions are indicated when the platelet count falls below 10,000 cells per μL to minimize the risk for spontaneous bleeding [18,19]. Transfusions thresholds should be increased before invasive procedures or in patients with bleeding to a level appropriate for any other intensive care unit (ICU) patient [18]. Platelet consumption may be increased in patients with fever, disseminated intravascular coagulation (DIC), or splenomegaly. Patients who have become alloimmunized to platelet antigens demonstrate poor response to platelet transfusions and may achieve higher platelet counts by limiting the number of donor exposures, controlling fever or DIC, using platelet products that are less than 48 hours old, or use of nonpooled (single-donor) or HLA-matched platelets [20,21].
Precautions should be taken in preparation of blood products for transfusion into HCT patients because passenger lymphocytes pose a risk for generating GVHD and latent viruses may be transferred through leukocytes. Except for the stem cell graft, all other components should be irradiated at a dose of 1,500 to 3,000 cGy to inactivate or eliminate contaminating lymphocytes. Depletion of leukocytes or use of blood components that test seronegative for cytomegalovirus (CMV) is effective for prevention of CMV transmission to CMV-seronegative recipients [21]. Removal of white blood cells from platelet and red blood cell products also decreases the risk for alloimmunization of the patient [22].
Regimen-Related Toxicity
High-dose cytotoxic chemotherapy with or without doses of TBI exceeding 6 Gy may severely disrupt mucosal integrity and has the potential to cause RRT in the skin, GI tract, liver, bladder, lung, heart, kidney, and nervous system. RRT occurs predominantly within the first 3 to 4 weeks after conditioning [23] and is more common after myeloablative than nonmyeloablative conditioning. RRT increases the risk for opportunistic infection, which is already high because of concomitant profound immunosuppression and regimen-related cytopenias. This section will focus on the noninfectious complications of individual organs specifically attributable to conditioning toxicity. Opportunistic infection or, after allografting, GVHD must strongly be considered as etiologies for organ dysfunction in the differential diagnosis of RRT. These alternative diagnoses are covered elsewhere under the appropriate subsection.
Skin
Generalized skin erythema is common after doses of TBI exceeding 12 Gy but is self-limiting and rarely associated with skin breakdown. Regimens that contain cytosine arabinoside (Ara-C), thiotepa, busulfan, etoposide, and carmustine may also cause erythema. Hyperpigmentation typically follows the inflammatory dermatitis, with skin folds often being particularly noticeable. Skin biopsies during the first 3 weeks after transplant often show nonspecific inflammatory changes irrespective of cause, making them frequently unhelpful in distinguishing between RRT, drug allergies, or acute GVHD [24].
Gastrointestinal Tract
Mucositis
Most patients who receive high-dose conditioning regimens develop mucositis. Symptoms include inflammation, desquamation, and edema of the oral and pharyngeal epithelial tissue that typically presents within the first several days after HCT and usually resolves by the third week. Anorexia, nausea, or other intestinal symptoms that persist after day 21 are more likely to be caused by GVHD or infection. Severe mucositis places patients at risk for aspiration and occasionally airway compromise, indicating the need for endotracheal intubation. Damage to the mucosa of the lower GI tract results in secretory diarrhea, cramping abdominal pain, and anorexia, and it facilitates translocation of intestinal bacteria with sepsis [23,25].
Mucositis is treated supportively with total parenteral nutrition, administration of intravenous fluids, and intravenous narcotics for pain control. It is important to recognize an iatrogenic narcotic bowel syndrome, characterized by abdominal pain and bowel dilatation, which occasionally may be a side effect of efforts to control painful symptoms of mucositis or sinusoidal-obstruction syndrome [26].
Acute Upper Esophageal Bleeding
The combination of mucositis, thrombocytopenia, and severe retching may result in a Mallory–Weiss tear, or esophageal hematoma [27]. The latter condition may have associated symptoms of dysphagia and retrosternal pain, and can be diagnosed by computed tomography (CT) scan. These conditions are treated supportively with transfusions to maintain platelet counts of greater than 50,000 per μL and optimal management of nausea and vomiting.
Liver
Sinusoidal Obstruction Syndrome
Sinusoidal obstruction syndrome (SOS; formerly referred to as veno-occlusive disease) develops in 10% to 60% of patients and is a clinical diagnosis based on the triad of tender hepatomegaly, jaundice, and unexplained weight gain usually within 30 days after HCT and in the absence of other explanations for these symptoms and signs [28,29]. It is more likely to be severe in patients with cirrhosis or fibrosis of the liver, or those with a history of hepatitis or liver irradiation (greater than 12 Gy), or chemotherapy-induced SOS [29,30].
Elevations of total serum bilirubin and serum transaminases are sensitive but nonspecific markers for SOS, and urinary sodium levels are typically low. A hepatobiliary ultrasound may show hepatomegaly, ascites, and dilatation of the hepatic vein or biliary system [31]. Doppler ultrasonography may show attenuation, or diagnostic, reversal of hepatic venous flow, but absence of this pattern does not exclude SOS [32]. If the diagnosis remains unclear, a transvenous liver biopsy may be helpful, and simultaneous measurement of hepatic venous pressure showing a gradient of greater than 10 mm Hg is highly specific for SOS [33].
Other causes of jaundice after HCT seldom lead to renal sodium avidity, rapid weight gain, or hepatomegaly. Cyclosporine, methotrexate, and total parenteral nutrition are iatrogenic causes of hyperbilirubinemia, although rarely cause levels greater than 4 mg per dL [34]. Combinations of illnesses that may mimic SOS are cholangitis lenta (cholestatic effects of endotoxin [35], especially when combined with renal insufficiency); cholestatic liver disease with hemolysis and congestive heart failure; GVHD and sepsis syndrome.
Once SOS is established, mathematical models can be used to predict prognosis, based on rates of increase in serum bilirubin and weight according to the elapsed time after transplantation [29,36]. The treatment for the 70% to 85% of patients who are predicted to have a mild or moderate course is largely supportive, with attention to management of sodium and water balance to avoid fluid overload [29]. Diuretics must be used judiciously to avoid depletion of intravascular volume and renal
hypoperfusion. Paracentesis is indicated if the degree of ascites threatens respiratory function. There is no universally effective therapy for severe SOS. However, multiple studies, including a recent large international multicenter phase II clinical trial, have demonstrated 30% to 60% complete remission rates with defibrotide, even among patients with severe SOS [37]. There is no support for insertion of peritoneovenous shunts and limited support for use of portosystemic shunts to reduce ascites [38]. Liver transplantation has been successful in a small number of patients [39].
hypoperfusion. Paracentesis is indicated if the degree of ascites threatens respiratory function. There is no universally effective therapy for severe SOS. However, multiple studies, including a recent large international multicenter phase II clinical trial, have demonstrated 30% to 60% complete remission rates with defibrotide, even among patients with severe SOS [37]. There is no support for insertion of peritoneovenous shunts and limited support for use of portosystemic shunts to reduce ascites [38]. Liver transplantation has been successful in a small number of patients [39].
Lung
Pulmonary complications occur in 40% to 60% of patients after HCT [40,41]. Noninfectious pulmonary problems that may occur within 30 days from the transplant include idiopathic pneumonia syndrome (IPS), diffuse alveolar hemorrhage, pulmonary edema [42] due to excessive sodium and fluid administration or associated with SOS, or acute cardiomyopathy induced by cyclophosphamide, and sepsis with adult respiratory distress syndrome (ARDS) [43]. These complications occur more frequently in older patients, those who receive higher-dose conditioning regimens, and those with allogeneic donors, particularly HLA-disparate donors [44]. Although the incidence of life-threatening pulmonary infections has decreased over the past decade due to the introduction of routine antimicrobial prophylaxis, pulmonary complications continue to be a leading cause of death.
Idiopathic Pneumonia Syndrome
IPS is defined as a noninfectious inflammatory lung process that may be triggered by TBI and chemotherapies such as carmustine or busulfan. IPS has been reported in 5% to 10% of patients and occurs with a median onset of 2 to 3 weeks after myeloablative HCT [44,45]. Contributing factors to IPS lung injury may be release of inflammatory cytokines due to alloreactivity or sepsis. The clinical symptoms cannot be distinguished from infection, and may include fever, nonproductive cough, and tachypnea. Hemoptysis is infrequent and more likely related to indicate invasive fungal disease or diffuse alveolar hemorrhage. Radiographic imaging shows diffuse interstitial or multifocal intra-alveolar infiltrates. Arterial blood gases show hypoxemia and the alveolar–arterial oxygen gradient is increased. In the occasional patient who is not too ill to attempt lung function studies, a new restrictive pattern or a reduced diffusing capacity is characteristic. Measurements of pulmonary artery occlusion pressure or echocardiography may be useful to rule out cardiogenic pulmonary edema. Bronchoalveolar lavage or lung biopsy is necessary to exclude bacterial, fungal, or viral infection because IPS is a diagnosis of exclusion. Multifocal bronchiolitis obliterans with organizing pneumonia (BOOP) may mimic late-onset IPS and has been more commonly associated with chronic GVHD.
Management of IPS is mainly supportive, including judicious diuresis to decrease pulmonary edema, transfusions of blood components to reverse bleeding diathesis, support of oxygenation, and administration of antibiotics to prevent superinfection with mold and bacteria, particularly in patients receiving high-dose glucocorticoids. Effective therapy for idiopathic pneumonia has not been demonstrated. High-dose glucocorticoids (1 to 2 mg per kg) have been reported to have an adjunctive role in treatment of diffuse alveolar hemorrhage or idiopathic pneumonia, but their efficacy has not been validated in controlled studies [46]. In a recent study of 15 patients who had IPS after allogeneic HCT, combination treatment with soluble tumor necrosis factor receptor (etanercept) and glucocorticoids resulted in an encouraging day-28 survival rate of 73% [47]. More than half of the patients included in this study had required mechanical ventilation at therapy onset. Long-term survival, however, did not appear to be superior compared with historic controls.
The mortality associated with IPS after myeloablative HCT is 50% to 70% [45,48]. Aggressive management, including initiation of mechanical ventilation to identify and treat reversible causes of respiratory failure, is a reasonable approach for most HCT recipients with diffuse or multifocal pulmonary infiltrates. When hemodynamic instability or sustained hepatic and renal failure develop, survival is extremely unlikely. Withdrawal of mechanical ventilation may be appropriate in specific situations.
Acute Respiratory Distress Syndrome
An ARDS-like syndrome also has been described as a presenting feature of acute GVHD, typically early-onset (hyperacute) GVHD. ARDS has an extremely high mortality rate in the transplant population; recovery depends on aggressive treatment of associated infections and support of respiratory and cardiac function [49,50]. The diagnosis of ARDS often is complicated by presence of other illnesses, such as SOS, hemorrhage, or disseminated intravascular hemolysis, which can cause difficulties in fluid management and indicate the need for pulmonary artery catheterization.
Diffuse Alveolar Hemorrhage
Diffuse alveolar hemorrhage may be a manifestation of diffuse alveolar damage. However, the erosion of blood vessels by fungal organisms always needs to be considered [51]. Hemorrhage occurs more frequently in older patients and those with malignancy, severe mucositis, or renal failure [52]. Bloody bronchoalveolar lavage (BAL) fluid with hemosiderin-laden macrophages is characteristic of diffuse alveolar hemorrhage.
Heart
Cardiac complications occur in 5% to 10% of patients after HCT, but death from cardiac failure is uncommon [53,54]. Cardiac injury with hemorrhagic myocardial necrosis is a rare but known adverse effect of high-dose cyclophosphamide, one of the most commonly used chemotherapy agents in conditioning regimens. Acute cardiac failure due to cyclophosphamide has a case mortality rate exceeding 50%. Risk factors for cyclophosphamide cardiotoxicity include use of doses equal to or greater than 120 mg per kg, an underlying diagnosis of lymphoma, prior radiation to the mediastinum or left chest wall, older age, and prior abnormal cardiac ejection fraction [54,55]. Patients who had prior cumulative anthracycline exposures of 550 mg per m2 doxorubicin equivalents are at an increased risk for developing heart failure. Signs and symptoms of congestive heart failure may occur within a few days of receiving cyclophosphamide, while anthracycline-related cardiomyopathy may have a delayed onset. The electrocardiogram (ECG) may show voltage loss or arrhythmia, and echocardiography may reveal systolic dysfunction, pericardial effusion or tamponade [56]. Older age and a history of abnormal ejection fraction are other factors that predispose to cardiac toxicity [54]. Management includes attention to fluid and sodium balance, afterload reduction, and inotropes.
Kidney and Bladder
Acute Renal Failure
Acute renal failure (ARF), defined by doubling of baseline serum creatinine, occurs in 30% to 50% of all patients during the first 100 days after HCT, and most often during the first 10 to 30 days [57,58]. Occasionally, ARF develops during conditioning or infusion of HSC, as a consequence of tumor or red-cell lysis. ARF occurs most frequently in the setting of SOS and is characterized by low urinary sodium concentration and high blood urea nitrogen to creatinine ratio, similar to the hepatorenal syndrome. Renal hypoperfusion, caused by
acute hemorrhage, sepsis, or high-volume diarrhea, may result in ARF. Nephrotoxic drugs like cyclosporine, tacrolimus, all amphotericin products, and aminoglycosides frequently cause renal insufficiency.
acute hemorrhage, sepsis, or high-volume diarrhea, may result in ARF. Nephrotoxic drugs like cyclosporine, tacrolimus, all amphotericin products, and aminoglycosides frequently cause renal insufficiency.
Thrombotic microangiopathy (TMA), endothelial damage caused by chemoradiotherapy, cyclosporine, tacrolimus, or sirolimus, occurs in 5% to 20% of patients, more frequently in allograft recipients [59]. The hallmark of thrombotic microangiopathy is red blood cell (RBC) fragmentation (schistocytes) associated with increased RBC turnover (increased reticulocytes; elevations of serum lactate dehydrogenase and indirect bilirubin) without evidence for immune-mediated hemolysis or disseminated intravascular coagulation. The syndrome ranges from subclinical hemolysis to a life-threatening hemolytic syndrome, the latter being seen more frequently when sirolimus therapy is combined with cyclosporine or tacrolimus (calcineurin inhibitors, CNIs) and immediately following conditioning with busulfan and cyclophosphamide. High-therapeutic or supratherapeutic serum levels of CNIs or sirolimus are more prone to be associated with TMA [60]. Management involves careful assessment of volume status and discontinuation or adjustment of the drug levels of the offending agent(s). The use of plasma exchange has been associated with high mortality rates in most series [61] with recent exceptions [62], and may be skewed by selection bias because only the sickest patients are likely to receive the treatment. For this reason, determination of any survival benefit attributable to plasma exchange in the absence of a controlled study is impossible.
Hypertension
Hypertension develops in approximately 60% of patients after HCT, more often in patients given CNIs for GVHD prophylaxis. Glucocorticoid therapy also contributes to the development of hypertension. Uncontrolled hypertension may lead to fatal intracerebral bleeding in thrombocytopenic patients. Therefore, hypertension should be anticipated and controlled medically. Most patients respond to conventional antihypertensive therapy, such as a calcium channel blocker, angiotensin-converting enzyme inhibitor, or beta-blocker. Correction of hypomagnesemia, which often confounds CNI therapy, may improve control of hypertension [63].
Hemorrhagic Cystitis
High-dose cyclophosphamide is commonly used for conditioning, and one of its toxic metabolites, acrolein, accumulates in the urine and may cause a hemorrhagic chemical cystitis during the conditioning regimen or later after HCT [64,65]. Measures to prevent hemorrhagic cystitis include aggressive fluid hydration to increase urine volume that dilutes and minimizes contact of acrolein with the mucosa, and administration of the drug mesna, which provides free thiol groups to detoxify acrolein. Viral infections, particularly adenovirus and BK virus, also have been implicated in the development of hemorrhagic cystitis [66] and the diagnosis is established by viral culture or polymerase chain reaction (PCR) test of a urine sample [66]. Unless there is evidence of disseminated infection, viral cystitis is managed with supportive therapy, including aggressive hydration and platelet transfusions. Intravesicular infusions of ε-aminocaproic acid or prostaglandins have been reported to improve outcome of severe hemorrhagic cystitis [67]. Severe hemorrhagic cystitis caused by BK virus that proves refractory to supportive therapy may respond to therapy with cidofovir [68].
Central Nervous System
Noninfectious complications include cerebrovascular events and encephalopathies due to metabolic, toxic, and immune-mediated causes. Focal symptoms are more indicative of infectious or cerebrovascular mechanisms, while diffuse symptoms such as delirium or coma may have metabolic causes. Fever is not necessarily associated with central nervous system (CNS) infections. Infection should be considered as the cause of any neurologic symptom and should prompt evaluation, including obtaining CT or magnetic resonance imaging (MRI) scans of the head and a sample of cerebrospinal fluid for appropriate cultures, cytochemistry stains, and PCR tests should be undertaken.
Cerebrovascular Events
Thrombocytopenia poses a risk for intracranial hemorrhage, which usually presents as abrupt onset of focal neurologic deficit or mental status changes [69]. Patients with sickle cell disease have a predisposition to CNS hemorrhage after HCT and should be managed carefully by ensuring sufficient platelet and magnesium levels and strict control of hypertension [70]. Ischemic stroke is an unusual complication after HCT but has been reported in patients with Aspergillus infections, hypercoagulable states, or TMA [59,71].
Toxic Encephalopathies
Conditioning with high-dose busulfan or carmustine may cause encephalopathy and seizure prophylaxis with phenytoin is usual. High-dose cytarabine may cause cerebellar dysfunction, encephalopathy, and seizures. High-dose cyclophosphamide can be associated with the syndrome of inappropriate antidiuretic hormone (SIADH), rarely causing acute decline in the serum sodium that may prompt seizures. Fludarabine, used frequently in nonmyeloablative conditioning, may cause an encephalopathy.
A rare syndrome of encephalopathy and hyperammonemia without other chemical evidence of liver failure has been reported after HCT [72]. Contributing factors may include hypercatabolism induced by conditioning, glucocorticoids, or sepsis, and high nitrogen loads associated with parenteral nutrition or intestinal hemorrhage. The syndrome is difficult to reverse and has a high mortality rate. Treatment involves hemodialysis and administration of ammonia-trapping agents, such as sodium benzoate or sodium phenylacetate.
Related to a tendency to accumulate in nervous tissues due to their lipophilic characteristics, CNIs can cause a range of neurologic toxicities [73]. Tremor develops in most patients. Seizures have been reported in up to 6% of patients and may present in association with headaches, tremor, or visual disturbances [74]. Seizures should be managed with anticonvulsant therapy and cessation of the drug. When CNIs are essential for management of GVHD, substitution of one agent for the other, or reinstitution of the offending agent at a lower dose, may be feasible [75]. A unique and usually reversible syndrome of cortical blindness has been reported as a complication of cyclosporine treatment; hypertension and hypomagnesemia are thought to be predisposing factors [76]. Toxicity due to calcineurin inhibitor therapy may occur with “therapeutic” drug levels, and clinical suspicion is often confirmed by MRI scans that show multifocal areas of signal hyperintensity on T2 (time for 63% of transverse relaxation) and fluid-attenuated inversion recovery (FLAIR) sequences, most often in the occipital lobe white matter.
Glucocorticoid therapy may be associated with psychosis, mania, or delirium in a dose-dependent fashion. Seizures or altered sensorium may be associated with the use of sedative-hypnotic drugs and have been reported as adverse side effects of many of the commonly used antibiotics and antiviral agents. Metabolic encephalopathy may be associated with Gram-negative sepsis, hypoxic encephalopathy with IPS, and hepatic encephalopathy due to SOS or GVHD.
Treatment of metabolic encephalopathies should be directed at the underlying problem, and offending drugs have to be discontinued. In patients with CNI neurotoxicity, temporary discontinuation of the CNI and the restarting at a lower dose is usually successful. Short-term phenytoin for seizure prophylaxis may be indicated.
Infection
Conditioning regimens and GVHD severely impair host defense mechanisms, and the process of immune reconstitution necessarily requires many months for completion. Together these factors place patients at high risk for acquisition of severe infections. Proper medical care of patients after HCT includes measures to monitor and prevent infection, as it is a leading cause of death.
Prevention of infection is of vital importance to the success of HCT procedures. Hospitalized patients should be housed in single rooms that have positive-pressure airflow and ventilation systems with rapid air exchange and high-efficiency particulate air filtration [77]. Strict visitation, hand washing, and isolation policies should be instituted to prevent introduction or spread of communicable disease. A daily program of skin and oral care should include bathing all skin surfaces with mild soap, brushing teeth with a soft brush, frequent rinsing of the oral cavity with saline, and good perineal hygiene. The diet should exclude foods known to contain bacteria or fungi, and patients should avoid exposure to dried or fresh plants or flowers. Caregivers should be trained in the proper handling of central venous catheters.
Immunologic reconstitution after HCT can broadly be categorized into three phases, which are characterized by a spectrum of opportunistic infections. Advances in management of antimicrobial prevention of opportunistic infections after HCT are outlined in Table 188.2.
Table 188.2 Advances in Prevention of Opportunistic Infections after Allogeneic Hematopoietic Cell Transplantation | |||||||||||||||||||||||||||||||||
---|---|---|---|---|---|---|---|---|---|---|---|---|---|---|---|---|---|---|---|---|---|---|---|---|---|---|---|---|---|---|---|---|---|
|
Before Engraftment Period
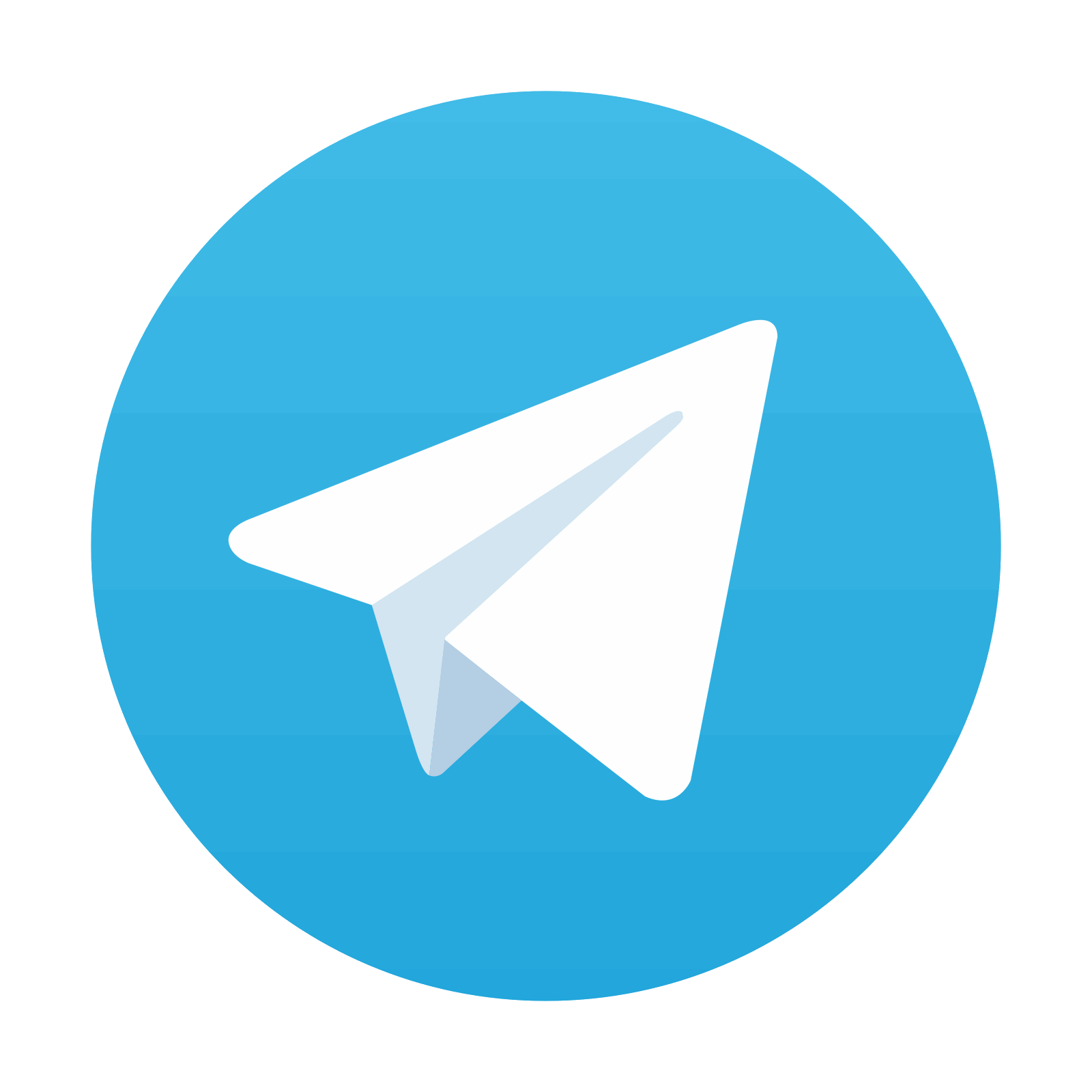
Stay updated, free articles. Join our Telegram channel

Full access? Get Clinical Tree
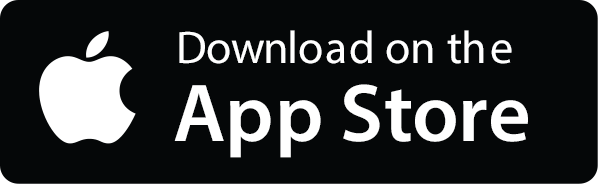
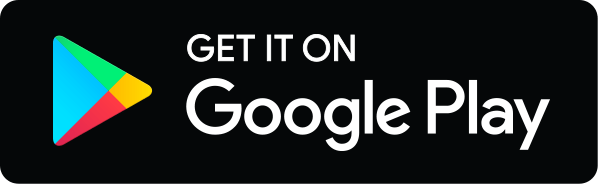
