Inherited Coagulation Disorders
Hemophilia
The hemophilias are a group of X-linked recessive bleeding disorders characterized by insufficient production of coagulation factor VIII (hemophilia A) or factor IX (hemophilia B). Formation of the platelet plug (primary hemostasis) is normal; however, stabilization of the plug by fibrin (secondary hemostasis) is defective because an insufficient amount of factor VIII or IX results in ineffective thrombin generation.
Hemophilia A results from a deficiency of factor VIII and occurs in approximately 1 in 5000 males. Hemophilia B results from a deficiency of factor IX and occurs in 1 in 30,000 males. Approximately 20% to 30% of female carriers produce low factor concentrations within the hemophilia range with associated bleeding tendencies. Clinically, adequate clotting usually occurs with 40% of normal factor levels.
The diagnosis of hemophilia is confirmed by demonstration of a prolonged partial thromboplastin time (PTT) plus low functional concentrations of either factor VIII or factor IX. Genetic testing is available for both hemophilia A and B. Approximately 98% of patients with either form of hemophilia will have an identifiable pathogenic variant.
The clinical manifestations of hemophilia A and B are indistinguishable. The severity is determined by the patient’s pathogenic variant and their baseline factor level and is categorized as severe (<1%), moderate (1%–5%) or mild (>5 to <40%). Mild hemophilia may go undiagnosed for many years, whereas severe hemophilia may manifest during early infancy as a result of a traumatic delivery (intracranial hemorrhage), bleeding after circumcision, or intramuscular injections. If bleeding does not occur in early infancy, the remainder of children with severe hemophilia are typically asymptomatic until they begin to crawl or walk. Severe hemophilia is characterized by spontaneous or traumatic hemorrhages, which can be subcutaneous (palpable bruising), intramuscular, or within joints (hemarthroses). Acute hemarthroses are exquisitely painful and if repeated over time will result in progressive joint damage and subsequent disability.
Hemophilia is treated by IV administration of the appropriate coagulation factor. These factors are produced by either plasma-derived or recombinant technology. Advances have been made in prolonging the half-life of factor products through the addition of different moieties (e.g., Fc protein fragment, albumin, or PEGylation) to the factor VIII or IX molecule.
A novel antibody therapy was approved for prophylaxis in patients with factor VIII deficiency with and without an inhibitor. Emicizumab (Hemlibra) is a recombinant monoclonal antibody that bridges activated factor IX and factor X to replace the function of the missing activated factor VIII. Emicizumab improves but does not normalize baseline hemostasis. The estimated hemostatic effect is thought to be similar to a patient with mild hemophilia (equivalent factor VIII level of 10%–20%). Emicizumab has a long half-life of 28 days and takes approximately 1 month to reach steady state with initiation of therapy. Factor VIII concentrate or bypassing therapy with recombinant factor VIIa can be used concurrently with emicizumab therapy.
Emicizumab will shorten the activated prothrombin complex concentrate (aPTT) and activitated clotting time ACT. Therefore, these tests cannot be used intraoperatively to monitor a patient’s ongoing coagulation status. This drug effect may last for up to 6 months after stopping the medication. In addition, any one-stage aPTT-based factor assays are also unreliable.
Factor-Replacement Therapy
Factor-replacement dosing is based on the type of product and individual pharmacokinetics. The plasma half-life of unmodified and plasma derived factor VIII is approximately 12 hours, and the plasma half-life for unmodified and plasma derived factor IX is approximately 24 hours. The prolonged factor VIII products have an estimated extended half-life of approximately 18 hours and the prolonged factor IX products have an extended half-life of 85 to 90 hours. Peak plasma factor concentration is reached 30 minutes to 1 hour after an intravenous infusion. Table 7.1 provides formulas for factor correction.
|
a Dosing can vary based on the patient’s individual pharmacokinetics.
In the United States, the majority of children with severe hemophilia are treated with prophylactic therapy, which consists of either IV factor administration at regular intervals to prevent spontaneous bleeding or subcutaneous injections of emicizumab (see Table 7.1 ). Patients with mild or moderate hemophilia are treated as needed to prevent bleeding before surgical procedures. For nonlife-threatening bleeding episodes, such as hemarthroses, coagulation factor activity should be raised to 40% to 50% of normal. For life-threatening bleeding, coagulation factor activity is raised to 80% to 100% of normal.
The goal of treatment in a patient with hemophilia undergoing a surgical procedure is to obtain a factor level of 0.8 to 1.0 units/mL (80%–100%) before the procedure. Factor replacement is continued into the postoperative period to prevent excessive bleeding and promote adequate healing. Factor replacement is usually continued for 2 to 3 days after a minor procedure and 10 to 14 days after major procedures. Intermittent dosing or continuous infusion factor replacement can be used to accomplish this goal. Preoperative evaluation for elective procedures in a patient with hemophilia should include measurement of an inhibitor titer and assessment of factor recovery and half-life.
Adjuvant hemostatic therapies include antifibrinolytics, such as aminocaproic acid and tranexamic acid, that inhibit clot lysis. Antifibrinolytic agents are particularly beneficial for surgeries that involve the oral mucosa (i.e., adenotonsillar procedures or dental extractions) because of a high concentration of fibrinolytic enzymes in saliva. Aminocaproic acid can be administered orally or IV, 100 mg/kg (maximum 6 grams) every 6 hours. Tranexamic acid is administered IV 10 mg/kg every 6 to 8 hours. A range of dosing has been reported for oral tranexamic from 10 to 25 mg/kg orally every 6 to 8 hours (maximum of 3900–6000 mg/day) has been reported.
An alternative hemostatic option for some patients with mild hemophilia A is desmopressin acetate (DDAVP). DDAVP is a synthetic vasopressin analog that causes release of factor VIII and von Willebrand factor from endothelial cells. In patients with mild hemophilia A, there is a significant variation in individual response to DDAVP, and it should not be used in severe or moderate hemophilia A. A trial administration to demonstrate an adequate rise in factor VIII levels is recommended before using DDAVP in the operative setting. (See von Willebrand disease section for more detailed information regarding DDAVP.)
Up to 30% of patients with severe hemophilia A and 3% to 5% of patients with hemophilia B develop an immune response to administered coagulation factors, with the development of IgG antibodies against factor VIII or IX. These antibodies are called inhibitors , and their development is the most significant treatment complication in hemophilia. High titer inhibitors interfere with the infused factor concentrates rendering them ineffective and necessitating the use of costlier and less effective alternative hemostatic agents. Alternative hemostatic agents for patients with an inhibitor include an aPCC or recombinant factor VIIa. The efficacy of these bypassing agents has significant interpatient variation and treatment is complicated with a lack of a clinical dose response relationship and ineffective laboratory methods to monitor efficacy. Because of these limitations, surgical interventions in patients with hemophilia and a high titer inhibitor should be limited to only those procedures deemed medically necessary.
For patients without an inhibitor who are receiving emicizumab prophylaxis, concurrent factor can be used in the setting of surgery to ensure adequate hemostasis. Minor procedures can be completed without additional therapy. Patients with inhibitors who are on emicizumab for prophylaxis and are having surgery should receive rFVIIa. The use of aPCC in combination with emicizumab has been associated with thrombotic events (venous and arterial) and thrombotic microangiopathy. This is hypothesized to be secondary to excessive FIXa from the aPCC that promotes abnormal clotting. In general, aPCC should not be used with emicizumab. If there is a poor clincial response to rFVIIa then aPCC can be used cautiously with recommended dosing <100 U/kg/24 hours.
Ideally, a hematologist should be involved in the perioperative management of patients with hemophilia to help develop an individualized hemostatic plan, consultation for intraoperative hemorrhage complications, and postoperative management.
Von Willebrand Disease
von Willebrand disease (vWD) is the most common hereditary bleeding disorder, present in up to 1% of the population. It results from a quantitative and/or qualitative defect of von Willebrand factor (vWF), a glycoprotein synthesized by endothelial cells and megakaryocytes. In the process of primary hemostasis, vWF acts as an adhesive bridge between the platelets and damaged subendothelium at the site of vascular injury. In the process of secondary hemostasis, it functions as the carrier protein for factor VIII.
There are different types of vWD:
- •
Type 1 (>85% of cases) is associated with quantitative reductions in all multimeric sizes of vWF. There is a wide variation of clinical manifestations, even for members of the same family. Children with type 1 vWD may be asymptomatic, or may have a history of frequent nosebleeds, mucosal bleeding, and easy bruising. A history of excessive bleeding during menses, or after mucosal surgery such as tonsillectomy or wisdom tooth extraction, is common. These patients are usually responsive to treatment with DDAVP.
- •
Type 2 is associated with quantitative and qualitative abnormalities of vWF and accounts for about 10% of cases. There are four subtypes of type 2 vWD: 2 A, 2 B, 2 M, and 2 N. Type 2 A includes genetic defects that impact multimer formation or processing. Type 2 B includes gain of function variants with vWF platelet binding and can be associated with mild thrombocytopenia. Type 2 M includes variants that result in decreased binding to platelets. Type 2 N results from pathogenic variants in the vWF FVIII binding region and results in low FVIII levels and can be mistaken for hemophilia A. Many patients with type 2 variants will not be responsive to DDAVP therapy, and require vWF replacement.
- •
Type 3 is the rarest and severest form, and results in a severe quantitative vWF deficiency associated with low factor VIII levels. It is associated with major bleeding requiring treatment with vWF and FVIII-containing concentrates.
Laboratory screening in patients with vWD may reveal a PTT, but many children with vWD have normal coagulation screening tests. More directed tests for vWD include a quantitative assay for vWF antigen, vWF (ristocetin cofactor) activity, plasma factor VIII activity, determination of vWF structure (vWF multimers), and a platelet count (decreased in type 2B vWD).
Treatment of vWD can be accomplished by using either DDAVP to stimulate the release of endogenous stores of vWF and factor VIII or through the use of either plasma-derived factor VIII-vWF concentrates (e.g., Humate-P) or recombinant vWF (e.g., Vonvendi). Treatment decisions are based on a patient’s type of vWD and their individual response to DDAVP.
Most commonly, children with type 1 vWD are responsive to DDAVP and are pretreated 30 minutes before surgery with IV DDAVP, 0.3 μg/kg. Additional doses can be administered every 12 to 24 hours postoperatively, but the response diminishes with repeated treatments as a result of tachyphylaxis. In general, DDAVP can be repeated for up to three to four consecutive doses. DDAVP has a known antidiuretic effect with resultant free water retention. Excessive fluid intake after DDAVP administration can result in hyponatremia and seizures. To minimize the risk for hyponatremia, fluid intake should be limited to two-thirds maintenance for 24 hours after DDAVP is given. This fluid limit includes the intraoperative period and should direct your anesthetic of choice and use of fluids. Even the use of isotonic fluids in the OR can still result in significant hyponatremia.
DDAVP is not used in patients who are nonresponders, type 3 vWD and some type 2 variants. In these patients, plasma-derived factor VIII concentrate with vWF or recombinant vWF is administered pre- and postoperatively. Plasma-derived vWF products should be dosed using ristocetin units and the half-life is approximately 12 hours. Recombinant vWF has a half-life of approximately 20 hours and contains ultra large multimers that do not exist in plasma-derived products.
Dosage formulae for recombinant and plasma derived FVIII vWF products (Humate P or Alphanate):
Dose (ristocetin units) = % Desired Rise × Weight × 0.5
As in hemophilia, antifibrinolytics can be used as a hemostatic adjuvant therapy in mucosal surgeries.
Hemoglobinopathies
The understanding of hemoglobinopathies requires knowledge of the structure and function of the hemoglobin molecule. All normal forms of hemoglobin contain a tetramer consisting of two alpha-polypeptide (globin) chains and two non-alpha chains. The non-alpha chains vary by the stage of maturation. For example, fetal hemoglobin (hemoglobin F) is composed of two alpha chains and two gamma chains, whereas adult hemoglobin (hemoglobin A) consists of two alpha and two beta chains. Each globin chain binds one heme group. A heme group consists of a porphyrin ring and a ferrous atom that binds to an oxygen molecule.
Sickle Cell Disease
Sickle cell disease (SCD) is an autosomal recessive disorder secondary to pathogenic variants in the beta-globin gene, and occurs in approximately 1 in 650 (0.15%) African Americans. Common forms of SCD include homozygous S or a combination of hemoglobin S with other hemoglobinopathies, commonly either hemoglobin C or beta thalassemia mutations. In all forms of SCD, the red cell containing the abnormal hemoglobin can assume an abnormal sickled shape, which predisposes the cells to impaired motility characteristics and hemolysis. Specifically, the sickled red cell membrane more easily adheres to the capillary endothelium resulting in occlusion of small capillaries and resultant reduction of perfusion to the peripheral tissues. The formation of sickled cells is promoted by hypoxemia, acidosis, hypothermia, dehydration, and hypotension.
The heterozygous state in which a child inherits a single affected beta globin gene is called sickle cell trait and is found in approximately 7% to 8% of African Americans. It does not cause anemia or red cell fragility. Rarely, an individual with sickle trait will exhibit painless hematuria and the inability to properly concentrate the urine (isosthenuria). They are also at increased risk for rhabdomyolosis from excessive exertion.
Clinical Syndromes in Sickle Cell Disease
Children with SCD exhibit a number of clinical syndromes, all of which are caused by aggregation of sickled red cells in small vessels, hemolysis, and decreased survival of abnormal red cells.
Painful vaso-occlusive events are the hallmarks of SCD. Pain results from vascular occlusion and resultant ischemia and can be seen in bony or soft tissues. Precipitating factors include infection, fever, cold exposure, dehydration, venous stasis, and acidosis. Dactylitis, or hand–foot syndrome, is painful swelling of the dorsal surface of the hands and feet caused by vaso-occlusion of the metacarpal and metatarsal bones. It is often the initial clinical manifestation of SCD in the first year of life. Older children typically develop pain crises in the long bones of the arms, legs, vertebral column, and sternum. Pain episodes typically last up to a week and may warrant hospitalization for administration of nonsteroidal antiinflammatory drugs (NSAIDs) and IV opioid analgesics.
Hemolytic anemia becomes apparent at approximately 4 to 6 months of age when the percentage of hemoglobin F diminishes and that of hemoglobin S increases. The anemia of SCD is chronic and well compensated. The chronic hemolysis is responsible for an increased risk for gallstone formation. In general, red cell transfusion is used during life-threatening complications such as aplastic crises, splenic sequestration, ACS, severe symptomatic anemia, or in preparation for major surgery. Chronic transfusion therapy is indicated for patients with severe SCD complications including stroke or recurrent ACS.
Splenic sequestration of sickled red blood cells leads to splenomegaly, acute exacerbation of anemia, and possibly hypovolemic shock. Splenic dysfunction occurs from repeated episodes of splenic infarction during childhood and results in susceptibility to sepsis from encapsulated organisms such as Streptococcus pneumoniae , Neisseria meningitidis , and Haemophilus influenzae . Children with SCD should receive vaccination against these organisms and daily penicillin prophylaxis and prompt evaluation for any fever >38.5°C.
Aplastic crises are typically caused by temporary cessation of red blood cell production in the bone marrow and are most often associated with parvovirus B-19 infection. Symptomatic anemia is treated with hospitalization and red cell transfusion. In addition, during an acute parvovirus infection, patients can have increase SCD complications including pain, splenic sequestration, and acute chest syndrome.
Acute chest syndrome (ACS) is a clinical diagnosis characterized by a new pulmonary infiltrate on chest radiograph associated with one or more clinical symptoms including fever, cough, tachypnea, dyspnea, or hypoxia. The pathophysiology of ACS in SCD is complex and multifactorial and includes infection (bacterial or viral), in situ vaso-occlusion, pulmonary edema, fat embolism, or thromboembolism. General anesthesia is a known risk factor for the development of ACS; therefore, preoperative transfusions are used to reduce this risk. Treatment of ACS includes supplemental oxygen, analgesia, antibiotics, and red cell transfusion to maximize respiratory function and minimize further pulmonary damage. Pulmonary complications account for a large proportion of morbidity and mortality in patients with SCD. During childhood, a substantial number of children with SCD will develop reactive airway disease and progressive pulmonary dysfunction. Up to 40% of adult patients develop moderate to severe pulmonary hypertension, which is associated with an increased mortality rate.
Stroke is one of the most disabling complications of SCD and occurs in approximately 11% of patients before the age of 20 years without screening interventions. The predominant etiology is a large vessel vasculopathy with proliferative intimal hyperplasia. Presenting signs and symptoms include mental status changes, seizures, and focal paralysis (e.g., hemiparesis). Stroke prevention is possible through routine transcranial Doppler imaging, which measures the velocity of cerebral blood flow and detects those children at increased risk for a first stroke. Children with persistently elevated transcranial Doppler measurements are placed on chronic prophylactic red cell transfusions. Children who have a stroke are also placed on a chronic red cell transfusion protocol to minimize the risk for future strokes.
Additional complications of SCD include priapism, retinopathy, leg ulcers, and progressive renal failure. By adolescence the effects of chronic myocardial microvascular obstruction and anemia result in ventricular hypertrophy. Microvascular obstruction of the intestinal circulation results in abdominal crises that manifest as signs and symptoms of an acute abdomen.
The treatment of sickle cell complications includes volume support, administration of antimicrobial agents, and prevention or reversal of anemia, hypothermia, hypoperfusion, acidosis, and pulmonary dysfunction. Hydration decreases blood viscosity and helps prevent capillary stasis. Red cell transfusion is an important aspect of sickle cell treatment because it increases the amount of hemoglobin A, while reducing the proportion of the patient’s own hemoglobin S, which is responsible for sickling. Red cell transfusions are targeted to a hemoglobin level of 10 to 11 g/dL; higher hemoglobin levels will unnecessarily increase blood viscosity. Exchange transfusion to reduce the hemoglobin S level to less than 30% is used in the context of life-threatening episodes such as stroke or severe ACS with pending respiratory failure that does not resolve with simple red cell transfusion.
Hydroxyurea is currently the main drug therapy that is used to prevent SCD complications. Hydroxyurea is thought to mitigate SCD complications through the induction of hemoglobin F production which stabilizes the red cell and prevents sickling. Hydroxyurea should be started at 9 to 12 months of age for all patients with SS or S-Beta-0-thalassemia. A monoclonal antibody (crizanlizumab tmca, Adakveo ) that functions as a p-selectin inhibitor may prevent recurrent pain in patients with all forms of SCD. In a clinical trial of 198 patients, crizanlizumab decreased the annual rate of pain crises by 45% (1.63 versus 2.98) and the duration of hospitalization by 42% (4 versus 6.87 days).
Perioperative Management of Children With Sickle Cell Disease
Historically, patients with SCD have not fared well in the perioperative period. Development of hypoxemia, hypotension, hypothermia, hypovolemia, and acidosis are associated with exacerbation of vaso-occlusive events, and should be prevented. All ongoing medical problems should be optimized before elective surgery. We follow the current National Institute of Health guidelines for perioperative management. Except for short and minor procedures, red cells should be administered to achieve a hemoglobin level of 10 g/dL. If the patient’s baseline hemoglobin is close to 10 g/dL exchange transfusion can be used. Patients with cerebral vasculopathy should have special consideration for anesthesia that maximizes cerebral perfusion. The use of a tourniquet during limb surgery is discouraged because of the possibility of precipitating distal limb hypoxia, hypothermia, and acidosis; however, poor outcomes after tourniquet use have not been reported. When appropriate, regional analgesic techniques are recommended for management of postoperative pain to reduce acute painful crises, to enhance respiratory function, and to minimize opioid use in the postoperative period. Postoperative mortality is often related to development of severe ACS.
Thalassemia
The thalassemias (from the Greek thalassa; thalassemia means blood as watery as the sea) encompass a group of inherited disorders of hemoglobin synthesis that involve decreased or defective synthesis of one or more globin chains. Thalassemias are named after the affected globin chain, with alpha and beta thalassemia being the most common and clinically important types. When one globin chain is ineffectively produced, the unaffected chains are overproduced, causing red cell abnormalities that lead to immature red cell destruction and a subsequent microcytic, hypochromic, hemolytic anemia.
Alpha Thalassemias
Hemoglobin Bart’s hydrops fetalis syndrome is the homozygous form of the disease that results in the absence of all four alpha globin chains. This condition results in the fetal overproduction of gamma globin chains (hemoglobin Bart’s) that are incapable of releasing oxygen to the tissues. Severe fetal anemia (hydrops fetalis) develops and is not compatible with intrauterine life unless intrauterine transfusions are instituted. After birth, life-long transfusions are necessary and bone marrow transplantation is curative.
Alpha thalassemia intermedia (hemoglobin H disease) results when three globin chains are absent. Hemoglobin H is the beta-4 tetramer that forms as a result of decreased alpha globin production and relative overproduction of beta globin chains. It is characterized by a mild to moderate microcytic anemia. Patients may develop splenomegaly but only rarely require splenectomy. Alpha thalassemia trait (alpha thalassemia minor) is the heterozygous form of the disease that results in the absence of two globin chains. It is present in approximately 3% of African Americans, and manifests as a microcytic anemia (rarely less than 9 g/dL) that is often confused with mild iron deficiency.
Silent-carrier alpha thalassemia is characterized by the absence of only one globin chain. The hemoglobin concentration and red cell indices are normal.
Beta Thalassemias
Beta thalassemia major (Cooley anemia) is the homozygous form of the disease characterized by the absence of beta globin chains. These children develop a severe anemia and splenomegaly during the first year of life. If the condition is left untreated, bone marrow hyperplasia and extramedullary hematopoiesis produce characteristic features such as tower skull, frontal bossing, maxillary hypertrophy with prominent cheekbones, and an overbite. In the absence of chronic blood transfusions, death occurs within the first few years of life owing to progressive congestive heart failure. A typical treatment regimen will consist of 10 to 15 mL/kg of leukodepleted red blood cells every 3 to 5 weeks to maintain the hemoglobin above 9 to 10 g/dL. Transfusion therapy prevents the clinical manifestations of the anemia but requires concomitant chelation therapy to reverse transfusion-related iron overload.
Thalassemia intermedia is a compound heterozygous state and results in a moderate anemia that does not usually require regular blood transfusions. These patients usually present later in childhood, and may develop clinical manifestations of chronic anemia as seen in thalassemia major. Transfusion therapy is reserved for acute illnesses. Beta thalassemia trait is caused by the absence of one beta chain, and is characterized by an asymptomatic mild microcytic anemia with a hemoglobin level rarely less than 9 g/dL.
Anesthetic management of patients with severe forms of thalassemia (i.e., beta thalassemia major) should focus on ensuring an adequate hemoglobin level before the procedure. Patients with iron overload complicated by cardiac disease (i.e., dilated cardiomyopathy) and/or endocrinopathies (i.e., diabetes, hypothyroidism, hypoparathyroidism) require subspecialty consultation to aid in perioperative management. Patients with alpha or beta thalassemia trait do not require any additional hematologic interventions before surgery.
References
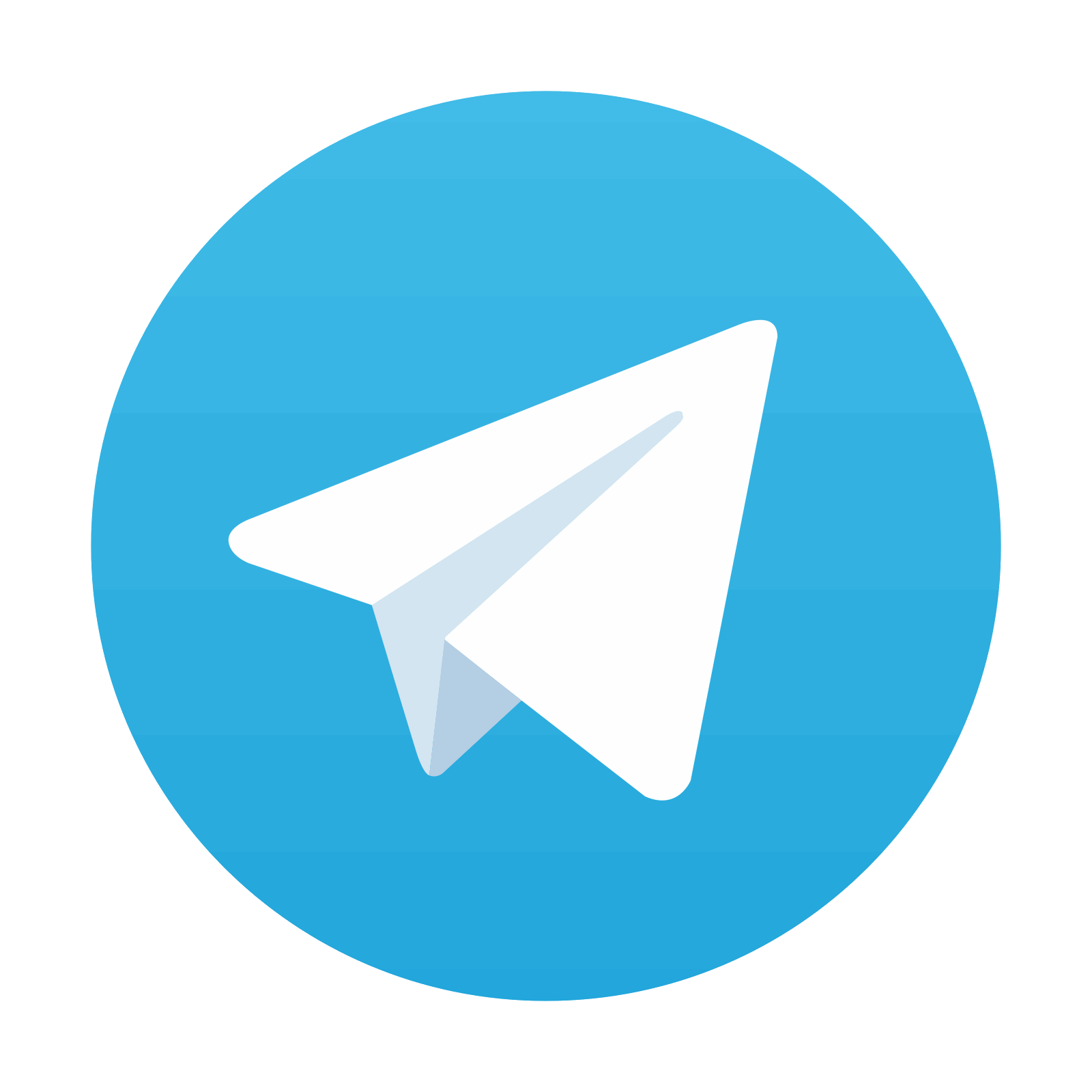
Stay updated, free articles. Join our Telegram channel

Full access? Get Clinical Tree
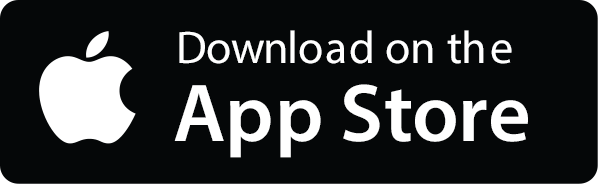
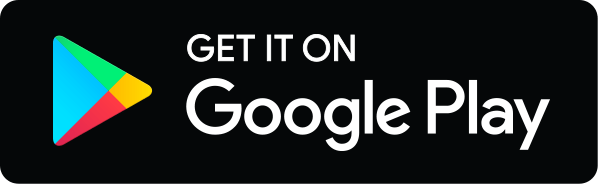
