Chapter 108 Heat Injury
The interest in heat-related illnesses has grown enormously, largely because of global warming and an increased frequency of heat waves.1,2 According to the Centers for Disease Control and Prevention, from 1999 to 2003, excessive heat exposure caused 3442 deaths in the United States.3 During this period, more people died of extreme heat than as a result of hurricanes, lightning, tornadoes, floods, and earthquakes. Among the pediatric population, neonates and infants are at highest risk, mainly because of poorly developed thermoregulatory mechanisms and total dependence on caregivers to provide adequate protection from excessive heat. Children with mental illness and chronic diseases are at high risk. Adolescents are also at increased risk mostly due to poor judgment or intoxication.
Over the past decade, the understanding of cellular and molecular responses to heat stress has improved dramatically. This is a multiorgan injury resulting from a complex interplay between the cytotoxic effect of the heat and the inflammatory and coagulation responses of the host.4 Despite progress in the understanding of the pathophysiology of heat injury, treatment remains supportive, with emphasis on immediate cooling. Prevention and education are still the best tools available in the hands of healthcare providers to minimize heat-related morbidity and death. This chapter covers the epidemiology, pathophysiology, clinical manifestations, and treatment of nonexertional heat-related illness in the pediatric population.
Definitions
Epidemiology
Excessive heat is the second largest contributor to death by natural events in the United States.8 In the period from 1999 to 2003, an annual average of 688 deaths in the United States was attributable to “excessive heat exposure.” Persons aged 15 years and younger accounted for 7% of deaths within the group of deaths caused by weather conditions.9 During heat waves, there is a significant increase in the heat-related death rate. For example, in 1980, a year with a record heat wave, the death rate was more than three times higher than that for any other year during the 19-year period of 1979 to 1997.10 Data on heat-related death are imprecise because this condition is underdiagnosed, its definition varies,4 and many cases of patients with near-fatal heat stroke who survive the acute hospitalization have a high 1-year death rate.11 In Saudi Arabia, where the temperature is extremely hot, the incidence of heat stroke varies seasonally, from 22 to 250 cases per 100,000 population.12 Heat-related illness is reported from subtropical and cold parts of the world as well. In Taiwan, a subtropical country without any history of heat waves, a cluster of heat shock cases was reported during periods of sustained hotter-than-average temperatures.13 In an observational study in which cold and hot areas in Europe were compared, it was shown that heat-related death started at higher temperatures in hot regions than in cold ones.14 High ambient temperature and humidity, lack of acclimatization, unavailability of air conditioning, and vigorous physical activity15 are major predisposing factors for heat-related illness. Within the pediatric population, children younger than 2 years are at higher risk, with specific factors like diarrheal disease, sweat gland dysfunction, child neglect, and underlying chronic or febrile illness contributing. Risk factors for adolescents include poor judgment that may lead to continuation of physical exertion despite warning symptoms. Alcohol and drug abuse and exposure to environmental toxins may put the adolescent at risk. Neuroleptic phenothiazines and tricyclic antidepressants, taken for medical indications; amphetamine and derivatives; marijuana and cocaine; or organophosphates, constituents of many pesticides, may all lead to heat-related illness.16 Their effect may be due to impaired heat loss or increased heat production.17 Lithium and fluoxetine (Prozac) may induce heat intolerance.18
Pathophysiology and Pathogenesis of Heat-Related Illnesses
Understanding the systemic and cellular pathophysiology of heat-related illnesses involves an appreciation of thermoregulation, physiologic alterations directly related to hyperthermia, acute phase response, and the production of heat shock proteins (HSPs). For normal enzymatic and cellular function, it is essential that body core temperature be maintained within a narrow range of about 37° C ± 0.5 to 0.9° C.16,19 The thermoregulation system, controlled by the hypothalamus, receives input from thermosensitive receptors in the skin and body core, compares the data with a reference level (the “set point”), and responds to an elevation of 0.3° C16,20 with activation of heat loss mechanisms.4,16,21
Heat dissipation occurs by means of four mechanisms: (1) conduction to the adjacent air and objects, (2) convection through air or liquid, (3) radiation of heat energy, and (4) evaporation. Once activated by the hypothalamus, the efferent heat response is both autonomic and behavioral. Blood delivery to the body surface is increased by sympathetic discharge causing cutaneous vasodilatation. Blood flow may increase eightfold to sixteenfold, up to 8 L/min.22 Thermal sweating, in response to parasympathetic discharge, can produce approximately 1 L/h/m2 of body surface of sweat. Evaporation of 1.7 mL of sweat will consume 1 kCal of heat energy; thus at maximal efficiency sweating can dissipate 588 kCal of energy per hour. Secondary to cutaneous vasodilatation and sweating, blood is shunted toward the periphery, and visceral perfusion is reduced, especially to the liver, kidneys, and intestines.23 Rising core temperature will also lead to tachycardia, a high cardiac output state, and an increase in minute ventilation. When ambient temperature equals or exceeds body temperature, conduction, convection, and radiation cease to be effective. Losses of salt and water through sweating may lead to dehydration and salt depletion, resulting in impaired thermoregulation.24 A combination of high ambient humidity and temperature creates a particularly dangerous situation. With ambient humidity of 90% to 95%, evaporation of sweat essentially stops, and if ambient temperature reaches body temperature, the body can no longer eliminate heat.
Acclimatization
Prolonged exposure to a hot environment results in adaptation and tolerance to higher temperature levels. Acclimatization to heat may take several weeks and involves multiple organs. Sweat glands develop increased capacity to secrete sweat, plasma volume is increased, and the renin-angiotensin-aldosterone axis is activated and leads to improved salt conservation. The adaptability of the cardiovascular system is probably the most important single determinant of one’s ability to tolerate heat stress.4,25 Even acclimatized people have definite limitations for heat tolerance. Once driven beyond a critical level, progression to a catastrophic condition may result.
Hyperthermia directly induces cellular injury. The severity of injury is cumulative, so exposure to a very high temperature for a brief period of time may cause similar injury to an exposure to a lower temperature for a longer period of time.26 Once extreme temperatures of 49° to 50° C have been reached, full destruction and cell necrosis occur. At lower temperatures cell death is mainly due to apoptosis.27
Acute Phase Response
Heat stress initiates cellular acute phase responses aimed at protecting against injury and promoting tissue repair. A variety of cytokines are produced in response to heat stress. Cytokines mediate a wide range of cellular, systemic, and both proinflammatory and antiinflammatory acute phase protein productions. For example, interleukin-6 (IL-6) has a pivotal role in modulating synthesis of inflammatory cytokines, both locally and systemically.4,28 IL-6 also stimulates production of antiinflammatory cytokines, which inhibit production of reactive oxygen species (ROS) and proteolytic enzyme release from activated leukocytes.28,29 Plasma levels of both proinflammatory (tumor necrosis factor alpha [TNF-α], IL-1, and interferon-γ) and antiinflammatory cytokines (IL-6, IL-10, TNF receptors p55 and p75) are elevated in patients with heat stroke.30–35 Soluble TNF, IL-2, and IL-6 receptors are also elevated in heat stroke.36,37 It has been shown that the severity of symptoms during heat stroke correlates well with IL-1 and IL-6 levels.30 Progression from heat stress to heat stroke depends on the time and extent of exposure to severe environmental conditions, but the acute phase response may continue after the patient is cooled. Onset of inflammation may be local with systemic progression,4,35 involving endothelial cell activation, release of endothelial vasoactive factors,38 endothelial cell injury, and microvascular thrombosis.38–41 The gastrointestinal tract may also play a role in the exaggeration of the inflammatory response. Vascular congestion, hemorrhage, thrombosis, and massive loss of surface epithelium in the jejunum was observed in a baboon model of heat stroke.42 These changes facilitate bacterial and endotoxin translocation which contributes significantly to inflammation and multiple organ dysfunction syndrome (MODS).23,43–46 Evidence for this phenomenon exists in animal models, but much less in humans.23,43,45–49 Alterations in the barrier function of the intestines may allow leakage of endotoxins that fuel the inflammatory response.
Part of the effect on endothelial cells involves activation of both the coagulation and the fibrinolytic systems.40 Heat stress by itself is a procoagulation condition since it causes platelet clumping in small vessels. In addition, heat stress may mediate endotoxemia, elevated levels of proinflammatory cytokines, and macrophage activation (via factor VIIa), all of which are well known inducers of coagulation. Injured endothelium, (e.g., heat stroke) plays an important role in producing and releasing both procoagulant and anticoagulant substances (e.g., von Willebrand factor antigen [vWF-Ag], tissue plasminogen activator, and plasminogen activator inhibitor).38,39 Circulating vWF-Ag, thrombomodulin, endothelin-1, nitric oxide (NO) metabolites, soluble E-selectin, and ICAM-1 (intercellular adhesion molecule 1) are elevated in heat-related illness, creating a clinical picture of disseminated intravascular coagulation (DIC).38,50–52 The cooling of patients with heat stroke reverses only part of these coagulation abnormalities.40 Another aspect of the cellular response to stress involves the heat shock response.
Nearly all cells will respond to heat stress with increased production of HSPs. Expression of HSP is controlled mainly at the gene transcription level. Increased intracellular HSP levels facilitate tolerance to heat stress with better cell survival.53,54 In animal models, it has been shown that preconditioning with heat or chemical stress conferred significant protection against heat stroke-induced hyperthermia, hypotension, and brain injury.55–57 These effects were mediated mainly by Hsp70 and Hsp72.56 Blocking the production of HSP results in increased cellular sensitivity to even mild degrees of heat stress.58 Conditions associated with low level of expression of HSP such as lack of acclimatization or certain genetic polymorphisms may make certain patients more vulnerable to heat stress or faster progression to heat stroke. There appears to be a preferential expression of different families of HSPs in different cell populations. There are also distinct post-injury time frames of induction for each family of HSP, emphasizing differences in cellular functional requirements for each family of HSP.59 It was suggested that Hsp72 may be used as a semiquantitative diagnostic probe of heat stress.60 In one human study, researchers found that levels of autoantibodies against Hsp71 in heat-induced diseases correlated well with the severity of illness.61 Thus the individual response to heat stress depends on the direct thermal injury (including thermal cytotoxicity, cardiovascular failure, and hypoxia in the face of increased metabolic requirement) and the acute-phase response of the host. Genetic factors likely play a significant role in determining response to heat stress. This complex interplay between leukocytes, endothelial and epithelial cells, and a variety of systemic changes may lead to the most extreme form of heat-related illness, heat stroke. A similar sequence of events has been shown to occur in sepsis.62 Recent studies, using a baboon model for heat stroke, provide more data on pathways of heat stroke-induced tissue injury and cell death. This model can be used to evaluate clinical changes and may be suitable to test immunomodulation therapies to improve outcome.42,63
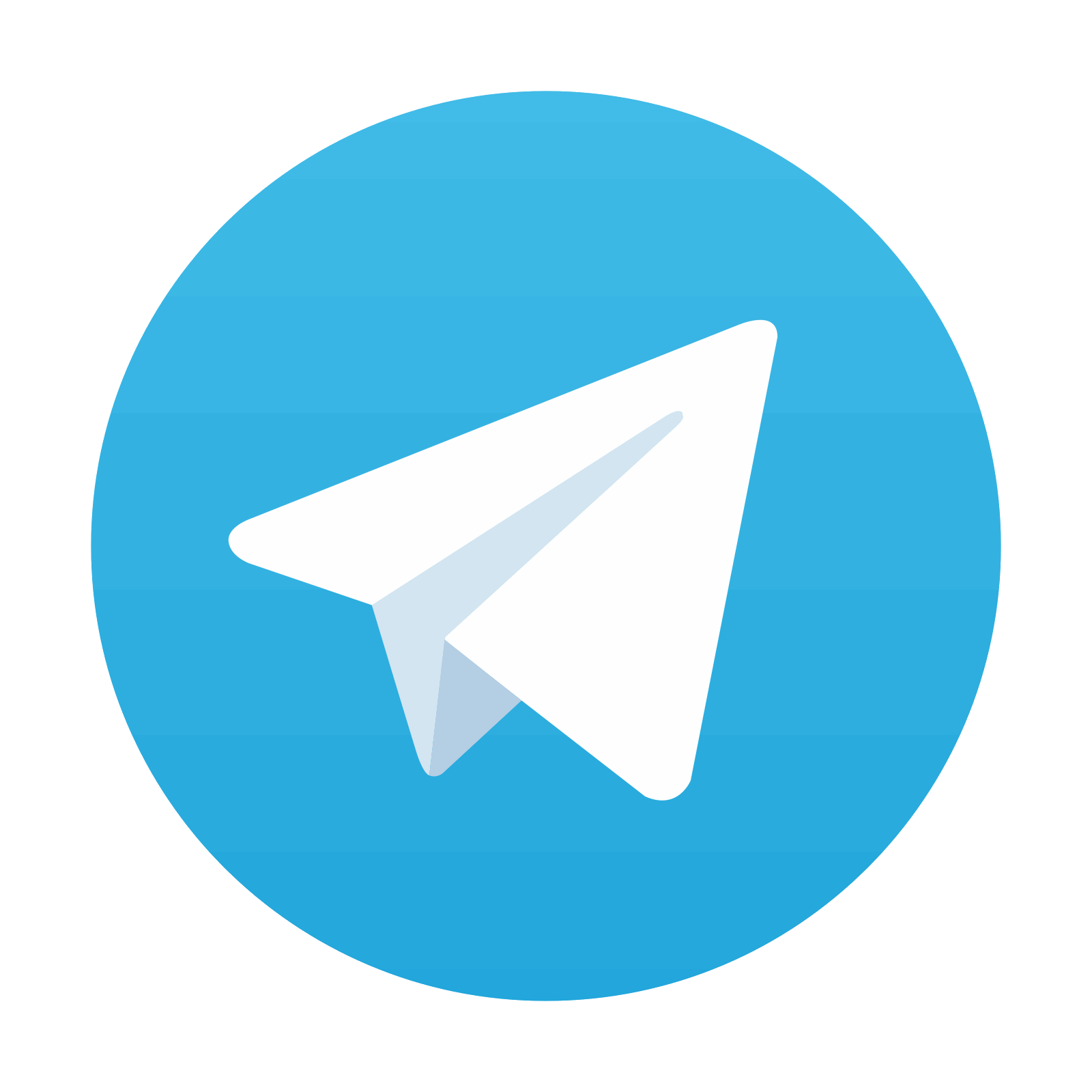
Stay updated, free articles. Join our Telegram channel

Full access? Get Clinical Tree
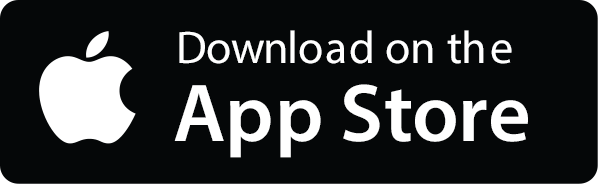
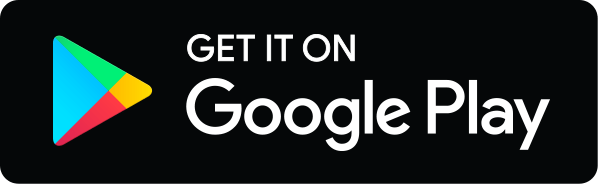