Head Trauma (Severe)
Scott A. Goldberg
Cappi Lay
THE CLINICAL CHALLENGE
Management of severe traumatic brain injury (TBI) focuses not on reversing the initial insult but on minimizing sequelae and preventing secondary injury mediated by hypoxia, hypotension, hyperventilation, seizures, and increased bleeding. These secondary injuries, occurring after the initial insult, can have a profound impact on clinical course and patient outcomes.
Prehospital providers are tasked with the rapid identification of the brain-injured patient and the provision of expedient stabilization and transport to an appropriate facility for definitive care. Prehospital management focuses on the basics of stabilization and resuscitation, avoiding hypoxia, maintaining eucapnia, and identifying and correcting hypotension.
On arrival at the emergency department (ED), the patient should undergo an initial evaluation following the principles of trauma care. The airway should be secured, oxygenation and ventilation secured, and blood pressure optimized. The initial physical examination and brief neurologic examination should include an evaluation of mental status and calculation of a Glasgow Coma Scale (GCS) score, which should be frequently reassessed. In the setting of airway compromise necessitating endotracheal intubation, rapid sequence intubation (RSI) is the preferred technique, optimizing first-pass success. Preoxygenation is essential. Following intubation, the goals of ventilator management are to maintain normoxia and to avoid hyperventilation and hypocapnia. Blood pressure should be maintained with fluid resuscitation and the addition of vasopressors as necessary: Traditional mean arterial pressure (MAP) goals of 65 mm Hg may be insufficient to maintain cerebral perfusion pressure (CPP).
Patients who are anticoagulated should be expeditiously reversed with a goal international normalized ratio (INR) of <1.5. If the patient is taking antiplatelet agents, platelet transfusion may be considered, although existing literature is equivocal on its benefit. Tranexamic acid (TXA) should be administered if the patient presents within 3 hours of injury. Once the patient is stabilized, attention is turned toward management in the intensive care unit or surgical management with hematoma evacuation and cranial decompression.
PATHOPHYSIOLOGY
Anatomy and Intracranial Physiology
The brain is coated with three layers: the pia mater, adhered to the brain itself; the arachnoid mater; and the meningeal dura mater. The inner aspect of the skull is lined with periosteal dura, reflecting
back on itself to form the meningeal dura that compartmentalizes the various components of the brain. In adults, the bones of the skull are fused, creating a chamber with a fixed volume. The internal components of this vault are the brain tissue, cerebrospinal fluid (CSF), and arterial and venous blood compartments.
back on itself to form the meningeal dura that compartmentalizes the various components of the brain. In adults, the bones of the skull are fused, creating a chamber with a fixed volume. The internal components of this vault are the brain tissue, cerebrospinal fluid (CSF), and arterial and venous blood compartments.
All intracranial pressure (ICP) issues are intracranial volume issues. Owing to the rigid nature of the skull, the cumulative volume of intracranial contents must always equal the sum of the various components within it. As long as the cranium is intact, improving compliance requires the reduction of the volumes of one or more of these components. The CSF and venous blood compartments normally serve as a capacitor whose volumes can be extruded with little impact on neurologic function. However, because this capacitance is exhausted with the incursion of hemorrhage or edema, ICP rises rapidly.
Cerebral autoregulation also plays an important role in determining ICP. In the uninjured state, cerebral arterioles reflexively dilate and constrict in response to changes in systemic blood pressure, maintaining a constant cerebral blood flow (CBF). Increases in cerebral arteriolar diameter, even at constant flow, have the effect of increasing the intracranial blood volume. In the injured brain where intracranial compliance has been compromised, increases in arterial blood volume due to arteriolar dilation may lead to upward swings in ICP.
Injury Patterns
Primary brain injury occurs at the time of the initial insult. This may be the result of a direct force to the head or by an indirect force, such as in an acceleration-deceleration injury. Penetrating injuries may cause direct injury to the brain and surrounding tissues or indirect injury secondary to associated concussive forces. Both epidural hematoma (EDH) and subdural hematoma (SDH) can progress quickly owing to the high pressure of the arterial system. Traumatic subarachnoid hemorrhage (tSAH) is the most common pattern of severe TBI, resulting from shearing of the small subarachnoid vessels and leading to accumulation of blood in the subarachnoid space, within the CSF and meningeal intima. Unlike hemorrhagic lesions, traumatic axonal injury (TAI) results from stretching or tearing of axonal connections within the brain tissue. Intracerebral hematomas or contusions of the cerebrum or cerebellum may also occur.
Cerebral Herniation Syndromes
Herniation refers to the mechanical deformation of brain tissue caused by a mass lesion or cerebral swelling that destroys tissue architecture. Although herniation often occurs in the setting of increased total ICP, certain lesions may result in herniation syndromes and death at relatively low ICP as a result of their locations within the intracranial vault close to vital structures. The temporal lobe and cerebellum are examples of locations where lesions causing local swelling may rapidly result in neurologic deterioration and death due to their direct compression on the adjacent brainstem. Conversely, elevated ICP can cause diffuse neuronal injury even in the absence of frank herniation because of neuronal ischemia.
PREHOSPITAL CONCERNS
Field providers are an essential link between the point of injury and definitive care. Initial interventions provided on scene can impact morbidity and mortality; and prehospital providers are tasked with clinical decision-making, informing where, when, and how rapidly a patient is transported to hospital. Management in the field should be focused on the rapid identification of possible TBI and management of any other traumatic injuries. As with in-hospital management, prevention of secondary injury through the maintenance of blood pressure > 110 mm Hg systolic and oxygenation saturation > 90% is paramount. Continuous pulse oximetry monitoring and frequent assessment of blood pressure should be utilized. A clear algorithm for prehospital management focusing on adequate oxygenation, maintaining blood pressure, and avoiding hyperventilation should be developed and used by field providers.
Airway
Advanced airway management in the field is an inherently risky procedure, and every effort must be made to maximize first-pass success. Although it is clear that some patients require immediate
endotracheal intubation in the field, this population is not well defined. Advanced airway management comes at the expense of prolonged transport times and may delay definitive in-hospital care, which adversely impacts patient outcomes. Conversely, failure of the patient to protect their own airway, persistent hypoxia despite supplemental oxygen administration, and CO2 retention are all indications for advanced airway intervention.
endotracheal intubation in the field, this population is not well defined. Advanced airway management comes at the expense of prolonged transport times and may delay definitive in-hospital care, which adversely impacts patient outcomes. Conversely, failure of the patient to protect their own airway, persistent hypoxia despite supplemental oxygen administration, and CO2 retention are all indications for advanced airway intervention.
Paralytic-assisted endotracheal intubation in the field should only be performed by skilled practitioners practicing in a system with a rigorous quality assurance program and continuous provider training. In systems with short transport times and in patients in whom an oxygen saturation > 90% can be maintained with supplemental oxygen, definitive airway management should be delayed until arrival in the ED. All advanced airway placement should be confirmed with quantitative end-tidal capnography (ETCO2), which dramatically improves the detection of improperly placed airway devices and inadvertent hyperventilation by field providers.
Blood Pressure
Avoiding hypotension, and managing it when and if it does occur, is a critical element of the prehospital treatment of the head-injured patient.1 As MAP falls, CPP follows, predisposing to tissue ischemia. Blood pressure should be monitored as frequently as possible with the most advanced means available.2
Although the concept of prehospital permissive hypotension may be beneficial in some trauma patients, it is detrimental in the setting of brain injury.1 Hypotension should be immediately identified and aggressively managed. Any ongoing external hemorrhage should be expeditiously addressed and controlled. Scalp lacerations may bleed a large volume into a bulky dressing, and a less bulky dressing should be used with firm constant manual pressure applied to avoid excessive blood loss. Fluid resuscitation should be administered on the basis of local prehospital protocols, but generally consists of crystalloids. Hypertonic saline (HTS) has been suggested as a useful resuscitation solution for patients with TBI in the field, but clinical trials have consistently failed to show any benefit over standard resuscitation with crystalloid solution.3,4
Agitation
Severely head-injured patients may present agitated or combative. Transporting a patient in this condition is challenging, and may be unsafe for both patient and providers. Transporting an agitated patient who is fighting against physical restraints may exacerbate physical injury, cause an increase in ICP, and interfere with stabilization and management. The priority of the field provider mirrors that of in-hospital management, including rapidly identifying alternative etiologies of agitation such as hypoglycemia, hypoxia, or hypotension.
Options for management in the field may be limited, and will be based on local emergency medical service (EMS) protocols. Ketamine has a fast onset of action when administered by the intramuscular (IM) route, and may be a viable option for rapid sedation of the agitated patient if available to prehospital providers.5 Concerns about neurotoxicity and transient increases in ICP following administration have historically limited its use in TBI, although recent studies have not demonstrated any harm when used as an induction agent.6 Benzodiazepines including midazolam are also effective in the rapid sedation of an agitated patient, but the patient must be closely monitored for hypoventilation, hypoxia, and hypotension. Antipsychotics including droperidol, haloperidol, or olanzapine are infrequently carried by prehospital providers, but may be additional considerations if available.7,8
THE FOCUSED EXAMINATION
Initial Assessment
A thorough history and physical examination are important, but emergent stabilization must be prioritized in all patients with suspected TBI while minimizing secondary injury during the immediate resuscitation. A brief neurologic examination should be performed on all patients with suspected TBI if at all possible before the administration of sedatives or paralytics, and the neck should be evaluated for evidence of a cervical spine fracture. If the patient is unable to cooperate with a neurologic evaluation, a cervical collar should be placed and remain in place until a definitive neurologic examination can be performed.
Focused Neurologic Examination
Mental Status and Glasgow Coma Score
Evaluation of the patient’s mental status is one of the most critical components of the neurologic evaluation of the patient with TBI. The GCS (Table 12.1) is one of the strongest predictors of outcome following TBI and should be calculated on all patients with suspected TBI. More important than the initial GCS, however, is the change in GCS over time. A declining GCS is a marker of poor prognosis, and a decline in GCS score of ≥ 2 should prompt immediate reevaluation.
A reliable total GCS score may be challenging to obtain in certain patient populations, such as intubated or intoxicated patients or those with other injuries affecting the eye opening or speech component of the GCS score. In these patients, evaluation of the motor component of the GCS score in isolation may still provide sufficient information in lieu of the total GCS (Table 12.2).9
Pupil Examination
Although a portion of the general population has anisocoria at baseline, pupil asymmetry, and specifically a change in the pupillary examination over time, indicates increasing ICP. Although pupil asymmetry in isolation is neither sensitive nor specific for intracranial pathology, a change in pupil asymmetry should raise concern of increasing ICP.
Motor Examination
The patient’s acute motor examination should evaluate for symmetry of strength. A response to pressure is a reasonable surrogate in the comatose or otherwise uncooperative patient. Decorticate posturing (abnormal flexion) implies injury above the brainstem, whereas decerebrate posturing (abnormal extension) suggests an injury of the deeper brain areas and portends a poorer prognosis. Abnormal posturing on arrival at the ED strongly suggests increased ICP and should trigger immediate empiric treatment.
Invasive Monitoring
TBI outcomes are inversely related to the amount of time the patient spends with elevated ICP. Although invasive ICP monitor placement has the advantage of providing a numerical trigger for
delivering treatment, emergency clinicians will generally make decisions about ICP management in the absence of invasive ICP monitoring. Carefully monitoring the clinical examination and radiologic findings are a viable alternative to ICP monitoring, at least until such invasive monitoring is available.
delivering treatment, emergency clinicians will generally make decisions about ICP management in the absence of invasive ICP monitoring. Carefully monitoring the clinical examination and radiologic findings are a viable alternative to ICP monitoring, at least until such invasive monitoring is available.
TABLE 12.1 The Glasgow Coma Scale Score | ||||||||||||||||||||||||||||||||||
---|---|---|---|---|---|---|---|---|---|---|---|---|---|---|---|---|---|---|---|---|---|---|---|---|---|---|---|---|---|---|---|---|---|---|
|
TABLE 12.2 The mGCS Score and SMS | |||||||||||||||||||||||||||||||||||
---|---|---|---|---|---|---|---|---|---|---|---|---|---|---|---|---|---|---|---|---|---|---|---|---|---|---|---|---|---|---|---|---|---|---|---|
|
Laboratory Testing
No specific laboratory tests that reliably diagnose TBI or predict outcomes have been identified. Laboratory evaluation in patients with TBI should follow standard practice for management of the trauma patient. The INR should be routinely evaluated, and although there are no clear guidelines for INR target, we recommend an INR < 1.5.10 The direct oral anticoagulants (DOACs) are not reliably detected by routine coagulation testing, making the history essential in identifying patients who require reversal. The brain is a rich source of tissue thromboplastin that is released after injury, causing a consumptive coagulopathy with an elevated INR that is not related to oral anticoagulant use. An elevated INR in this case is a poor prognostic indicator.
Recent interest has shifted to the use of viscoelastic hemostatic assays in trauma such as thromboelastography (TEG) and rotational thromboelastometry (ROTEM) to better characterize the specific nature of the coagulopathy present and potentially guide treatments, providing information not only about the initiation of clotting but also about clot strength and fibrinolysis. Unfortunately, the standard TEG and ROTEM assays have inconsistent sensitivity for the presence of the newer DOACs such as dabigatran, rivaroxaban, and apixaban, as well as for antiplatelet medications such as aspirin and clopidogrel.
Radiography
The standard imaging modality in a patient with suspected TBI is non-contrast computed tomography (CT) scan of the head, which should be performed in any patient with head injury and a GCS of 14 or lower. CT imaging of the brain provides information about the likelihood of raised ICP, can demonstrate impending herniation, and guides the need for surgical intervention. The Marshall classification and the Rotterdam Score are CT grading scales developed for patients with TBI that have been shown to predict outcome. Both scoring systems have identified the presence of midline shift and compression or effacement of the basal cisterns as strongly predictive of mortality. Although hyperdense blood and obvious asymmetry are usually noticed on the CT, the loss of visible CSF spaces around the midbrain is more subtle and is an important clue to increased ICP and early herniation.
In healthy individuals, CT imaging of the brain normally demonstrates at least a thin margin of CSF outlining the midbrain (Figure 12.1(B)) , distinguishing it from the temporal lobes to either side. In the setting of uncal herniation, the ipsilateral temporal lobe is displaced medially, obliterating the CSF space on that side. In the setting of global cerebral edema that ultimately leads to downward transtentorial herniation, both cerebral hemispheres are displaced inferiorly, causing effacement of the cisterns bilaterally. Other CT findings that have been associated with worse outcome after TBI are the presence of midline shift >5 mm, and mass lesions >25 cm3. All patients with TBI with cisternal effacement or significant midline shift on CT should be treated as having critically elevated ICP.
Repeated CT imaging may be useful in monitoring the evolution of TBI, and is warranted if the patient’s condition changes. The utility of repeat CT scan in patients with stable neurologic examinations is less clear, and although a repeat scan 4 to 6 hours following an initial diagnostic scan is reasonable, it does not regularly change management.11 CT angiogram may be considered in patients without a clear etiology of head trauma or in those with central subarachnoid hemorrhage, although it rarely changes management.12,13
Ultrasound may help identify patients at risk for intracranial hypertension by assessing optic nerve sheath diameter. The optic nerve sheath is in continuity with the dura and envelops the optic nerve with a thin intervening layer of CSF that expands under conditions of increased ICP. Nerve sheath diameter of >5.0 mm has been demonstrated to correlate with presence of intracranial hypertension with a sensitivity of 97% and a specificity of 86%.14 Ultrasound thus has the potential to help the emergency provider expedite ICP lowering therapy in the first minutes after arrival before initial head imaging (see Chapter 16: Headache).
MANAGEMENT
Airway Management
In the setting of TBI, primary airway injury may result from concomitant craniofacial trauma or neck trauma, bleeding, or vomiting. Secondary airway compromise may be caused by loss of brainstem reflexes, or may result from agitation, hypotension, or mental status changes limiting the patient’s ability to protect their airway. In the setting of existing or anticipated airway compromise,
the airway should be secured early to prevent secondary injury from hypoxia or hypoventilation. A brief neurologic examination should be conducted before advanced airway management with sedation or paralysis.
the airway should be secured early to prevent secondary injury from hypoxia or hypoventilation. A brief neurologic examination should be conducted before advanced airway management with sedation or paralysis.
Before endotracheal intubation, the patient should be preoxygenated to avoid hypoxia during the intubation attempt. Volume resuscitation is paramount to avoid hypotension during intubation, especially when giving medications that may blunt sympathetic drive and its compensatory effect on blood pressure. If there is concern that the patient might become hypotensive during or immediately after intubation, vasopressors either as a drip or in push-dose should be administered before or during the intubation attempt.
Supraglottic stimulation during airway manipulation leads to a release of systemic catecholamines, resulting in a transient increase in ICP. Lidocaine to blunt this response or a defasciculating dose of succinylcholine have shown no benefit in patient-centered outcomes and are no longer recommended. Administration of a short-acting opioid, such as fentanyl at a dose of 3 mcg/kg, may blunt the sympathetic reflex and should be considered, but any benefit must be balanced against risks of hypotension.
RSI is safe and effective in the emergent setting, decreasing the risk of aspiration and increasing the likelihood of first pass success. Although research studies have not demonstrated a clear benefit of RSI over other techniques in terms of outcomes, it remains the preferred method of airway management in the patient with TBI. Etomidate (0.3 mg/kg) is the drug of choice owing to its hemodynamic stability. Ketamine (1-2 mg/kg) is an alternative, especially in a hypotensive patient. Although the use of ketamine in TBI has historically been controversial because of a purported transient increase in ICP after ketamine administration, it is generally considered safe and effective for this indication.15,16 Rocuronium (1.2 mg/kg) is a nondepolarizing agent and as such does not cause the fasciculations and corresponding rise in ICP seen with the depolarizing agents. However, its duration of action can exceed 1 hour, making repeat neurologic assessments challenging. As such, succinylcholine (1.5 mg/kg) is the drug of choice for paralysis in patients with TBI. Rocuronium would be a second-line agent for those with contraindications to succinylcholine.
Ventilator Management Following TBI
Following intubation, ventilation parameters should be carefully controlled and eucapnia meticulously maintained. The partial pressure of arterial carbon dioxide (PaCO2) is a potent regulator of cerebral arteriolar diameter, impacting ICP and in turn CBF. (see Figure 12.2). Reductions in PaCO2 cause reflex vasoconstriction throughout the brain, whereas hypercapnia results in vasodilatation. Although hyperventilation decreases ICP, the corresponding decrease in cerebral perfusion leads to worse patient outcomes. Unfortunately, unintentional hyperventilation during manual ventilation following intubation is common, underscoring the importance of using quantitative ETCO2. Although hypocapnia may be deleterious, hypercarbia (PaCO2 > 45 mm Hg) has also been associated with increased hospital mortality and should similarly be avoided.
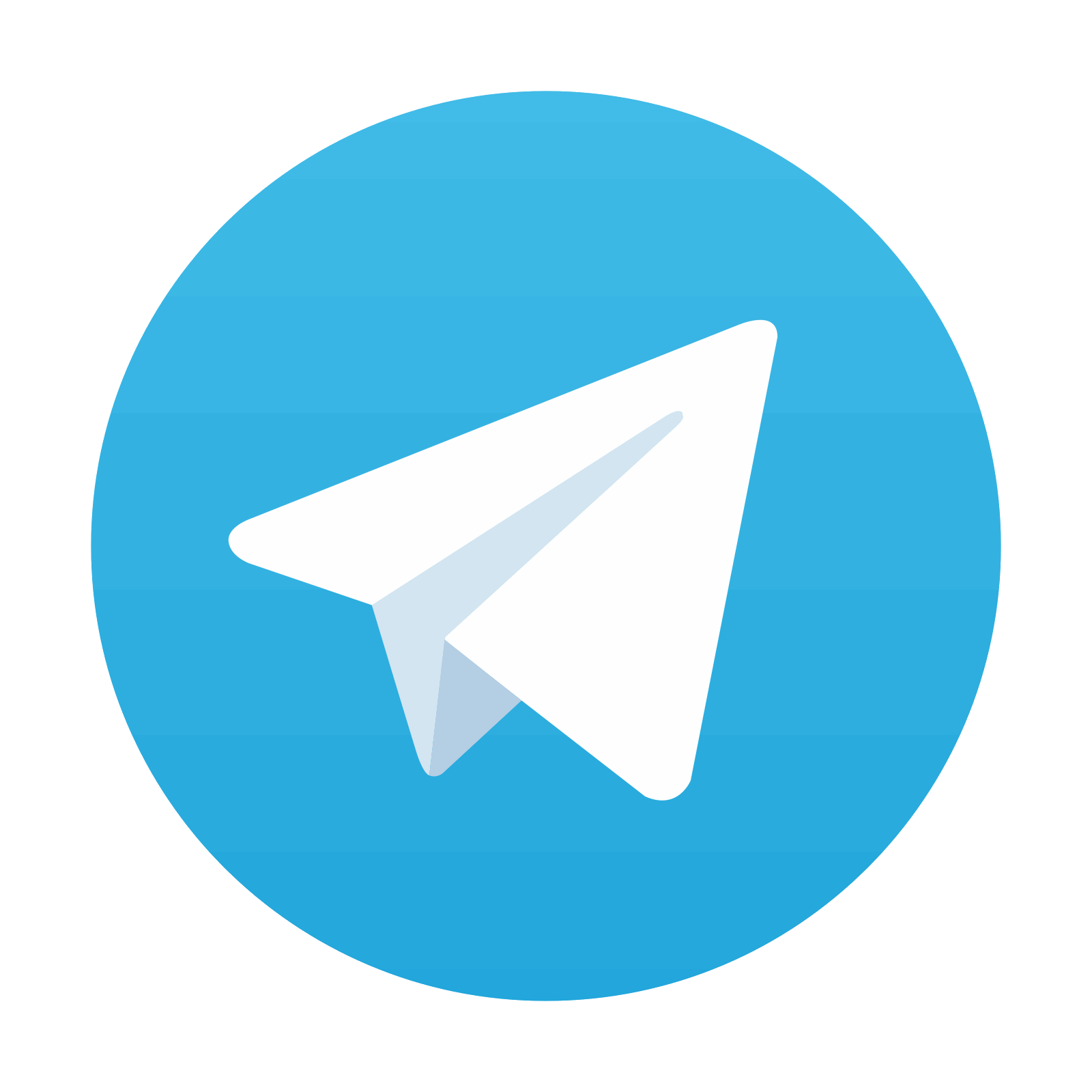
Stay updated, free articles. Join our Telegram channel

Full access? Get Clinical Tree
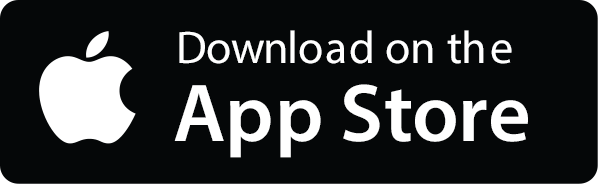
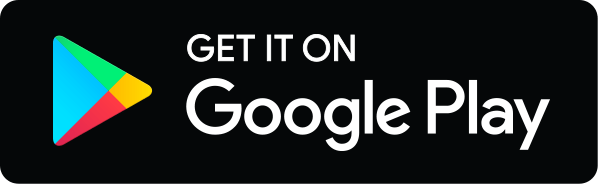