Fig. 3.1
Anatomy of the head: bony structures of the skull (top), the meninges (middle), and the brain (bottom) (adapted from Sobotta 1997)
The inner surface of the cranial vault is concave with an irregular plate of bone forming the base. This base plate contains several small holes for arteries, veins and nerves as well as a large hole (foramen magnum) through which the brainstem passes into the spinal cord.
Three membranes called the meninges protect and support the spinal cord and the brain and separate them from the surrounding bones (Fig. 3.1). From outside to inside, we find the dura mater, the arachnoidea mater, and the pia mater. The dura mater is a tough, fibrous membrane, while the arachnoidea mater resembles a spider-web. Both membranes are separated by a narrow space, the subdural space. Analogously, the subarachnoidal space separates the arachnoidea mater and the pia mater. The pia mater covers the surface of the brain, dipping well into its fissures. Cerebrospinal fluid (CSF) fills the subarachnoidal space and the ventricles of the brain and thus cushions the brain (and the spinal cord) from mechanical shock. As CSF constantly circulates and surrounds the brain on all sides, it serves as a buffer and helps to support the brain’s weight.
Several blood vessels cross the meninges supplying the brain and the scalp. The so-called bridging veins, i.e. the veins that bridge the subdural space, are of particular interest as they may be subject to injury through tearing (see Sect. 3.2.).
Finally, the central nervous system consisting of the brain and the spinal cord is located at the centre of the head. Structurally and functionally the brain can be divided into five parts: cerebrum, cerebellum, midbrain, pons and medulla oblongata (Fig. 3.1).
3.2 Injuries and Injury Mechanisms
The most important injuries to the head are those to the skull and the brain including the meninges. Figure 3.2 gives an schematic overview on possible head injuries. In principle, head injuries are characterised as open or closed depending on whether the dura mater is injured (open) or not (closed). Soft tissue injuries to the scalp and face commonly occur in automotive accidents. The resulting injuries include contusion and laceration but are generally regarded to be of minor importance. Likewise facial injuries, to the eyes or ears for example are considered minor injuries and therefore are mainly rated as AIS1 or AIS2. These injuries will not be discussed here.
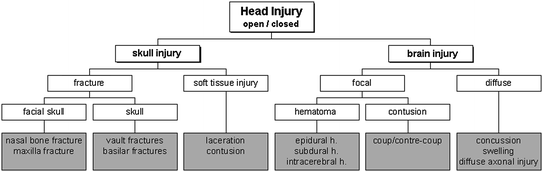
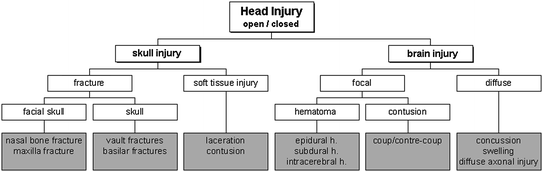
Fig. 3.2
Possible injuries to the head
More severe head injuries can arise from fractures. Facial fractures include fracture of the nasal bone, which occurs most frequently, and maxillary fractures. The latter are considered serious with AIS grades of up to 3. Figure 3.3 shows the LeFort classification that is used to categorise maxillary fractures. Examples of head injuries classified according to the AIS scale are presented in Table 3.1.
AIS code | Description |
---|---|
1 | Skin/scalp: abrasion, superficial laceration Face: nose fracture |
2 | Skin: major avulsion Vault fracture: simple, undisplaced Mandible fracture: open, displaced Maxilla fracture: LeFort I and II |
3 | Basilar fracture Maxilla fracture: LeFort III Total scalp loss Single contusion cerebellum |
4 | Vault fracture: complex, open with torn, exposed or loss of brain tissue Small epidural or subdural hematoma |
5 | Major penetrating injury (>2 cm) Brain stem compression Large epidural or subdural hematoma Diffuse axonal injury (DAI) |
6 | Massive destruction of both cranium and brain (crush injury) |
With respect to the skull, fractures are divided into basilar and vault fractures (i.e. all other fractures not occurring at the basis of the skull). It should be noted that basilar fractures may be difficult to visualise using conventional radiographic methods, so that diagnosis can be difficult.
Injuries to the brain are clinically classified into two broad categories: diffuse injuries and focal injuries. Diffuse brain injuries form a spectrum ranging from mild concussion to diffuse white matter injuries. The most common form of such brain injury is mild concussion (fully reversible, no loss of consciousness). Particularly in sports, mild traumatic brain injury (mTBI) is often diagnosed (see Sect. 3.5). A more severe form of concussion is cerebral concussion which is characterised by immediate loss of consciousness. The outcome of patients suffering from cerebral concussion strongly depends on whether there are associated brain injuries or not (Melvin and Lighthall 2002). Diffuse axonal injury (DAI) describes disruption to the axons in the cerebral hemispheres and the subcortical white matter.
Focal brain injuries are lesions where the damage is locally well-defined. Possible focal injuries are hematoma and contusions. Contusion is the most frequently found lesion following head impact. Generally, contusion occurs at the site of impact (coup contusion) and at site opposite the impact (contre-coup contusion). Contre-coup contusions are considered more significant than coup-contusions (Melvin and Lighthall 2002). As for hematoma, three different types are distinguished depending on the site of the bleeding: epidural hematoma, subdural hematoma and intracerebral hematoma (Fig. 3.4). Epidural hematoma, i.e. bleeding above the dura mater, is observed as a result of trauma to the skull and the underlying meningeal vessels. It is therefore not due to brain injury. Usually skull fracture is associated, but an epidural hematoma may also occur in the absence of fracture. If the hematoma is found below the dura mater, it is called a subdural hematoma. Three sources were identified for subdural hematoma: lacerations of cortical veins and arteries by penetrating wounds, large-contusion bleeding into the subdural space, and tearing of bridging veins between the brain’s surface and the dural sinuses. The mortality rate of this type of hematoma exceeds 30 % in most studies (Melvin and Lighthall 2002). Intracerebral hematomae are well-defined homogeneous collections of blood within the brain and can be distinguished from contusions by e.g. computer tomography. An increased intracranial pressure due to swelling of the brain may be the consequence of diffuse as well as focal injuries. This secondary effect may lead to a reduced cerebral blood flow and a reduced oxygen supply, whose consequences may well exceed those of the primary injury itself.
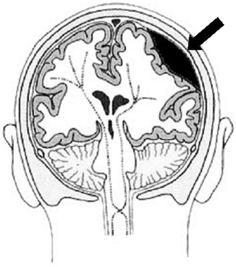
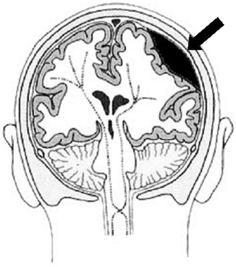
Fig. 3.4
Bleeding into the epidural space is called an epidural hematoma and can cause brain contusion (adapted from Vetter 2000)
The mechanisms causing head injuries are manifold. In principle, injuries can result from static and dynamic loading (Fig. 3.5). For our purpose, static loading is defined as a load lasting for more than 200 ms. Under such static loading the head deforms until it reaches a maximum deformation. Then the skull fractures, often leading to multiple fractures. In accidents, however, this type of loading is rare. Dynamic loading is the predominately loading scenario. Two types, contact and non-contact loading, are distinguished, each resulting in a different head response. Direct contact of the head to (or from) an object can cause the skull to deform, possibly resulting in direct fractures (mostly due to bending and often close to the impact location) or in indirect fractures (burst fractures oriented in the direction of the force vector). Furthermore, after deformation of the head local brain injury (even without fractures) like epidural hematoma or contusion as well as scalp injuries are observed. Additionally, rapid contact loading produces stress waves that propagate in the skull or the brain (Fig. 3.6). Wave propagation in the brain may lead to a pressure gradient with positive pressure at the site of impact (coup) and negative pressure on the opposite side of the impact (contre-coup). Such a mechanism is proposed for the generation of intracranial compression which causes focal injuries of the brain tissue and bruising. However, it is not yet fully understood whether the injury is due to negative pressure (tensile loading causing e.g. bleeding or tissue disruption) or due to a cavitation phenomenon (Viano 2001). In addition, the pressure gradient can give rise to shear strain within the deep structures of the brain.
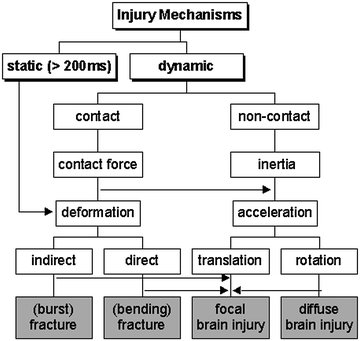
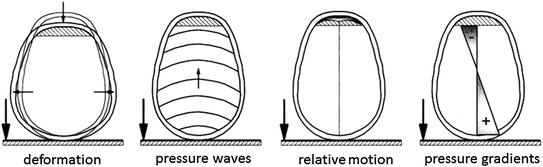
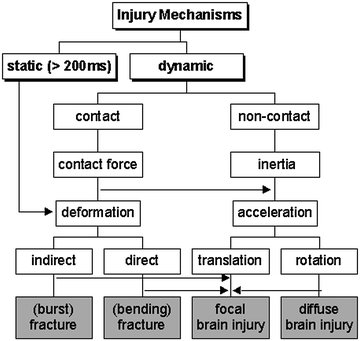
Fig. 3.5
Possible mechanisms of head injury
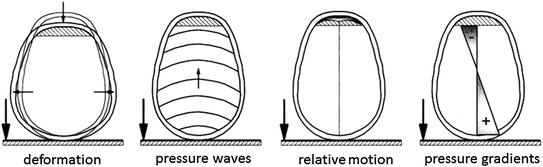
Fig. 3.6
Different injury mechanisms for contact impact; fractures do not necessarily occur (adapted from Vetter 2000)
Contact loading may also result in a relative motion of the brain surface with respect to the inner surface of the skull base. Surface contusions on the brain (so called gliding contusions) and tearing of the bridging veins (causing subdural hematoma) can be the consequences.
In non-contact situations, the head is loaded exclusively due to inertial forces, i.e. acceleration (or deceleration) of the head. Acceleration can either be translational or rotational. Translational acceleration generally results in focal brain injury while rotational acceleration also causes diffuse brain injury. As an exception, subdural hematoma, i.e. a focal skull injury, may arise due to acceleration induced relative motion between brain and skull tearing the bridging veins. Furthermore, acceleration response of the head does, of course, also occur in contact loading. Thus the mechanisms described above apply in the same way.
It should be noted that headaches, which are often erroneously thought to be due to a “head injury”, caused either by contact or non-contact mechanisms, may also be initiated by lesions in the upper area of the cervical spine. Therefore, a comprehensive analysis of the occupant dynamics must be undertaken in order to prevent premature diagnoses of cerebral concussion or mild traumatic brain injury (mTBI).
3.3 Mechanical Response of the Head
Many cadaver studies on head impact have been carried out to investigate the mechanical response properties of the head. In general, the impact responses were described in terms of head acceleration and impact force and therefore depend on the inertial properties of the head and impacted surface. For a 50th percentile male, the average head mass is 4.54 kg and the average mass moments of inertia are Ixx = 22.0 × 10−3 kgm2, Iyy = 24.2 × 10−3 kgm2, and Izz = 15.9 × 10−3 kgm2 (e.g. Beier et al. 1980). For the paediatric head only few data is available (see e.g. Prange et al. 2004).
In these cadaveric studies, mainly drop tests against a rigid flat surface were performed. Table 3.2 summarises the peak force values reported for fracture at different sites of the head. Furthermore the acceleration response of the head was investigated. When measuring the acceleration of the head, two problems arise: firstly, accelerometers cannot be mounted at the centre of gravity of the head and secondly, the head is not a rigid body. Therefore several methods for measuring the acceleration have been proposed (e.g. Padgaonka et al. 1975). It is also recommended to measure the head rotational acceleration so that the acceleration of the head’s centre of gravity can be computed thereof. Nonetheless there remain some uncertainties as the exact stiffness distribution of the skull is generally not known.
Table 3.2
Peak force for fracture at different regions of the skull
Impact area | Force (kN) | References |
---|---|---|
Frontal | 4.2 5.5 4.0 6.2 4.7 | Nahum et al. (1968) Hodgson and Thomas (1971) Schneider and Nahum (1972) Advani et al. (1975) Allsop et al. (1988) |
Lateral | 3.6 2.0 5.2 | Nahum et al. (1968) Schneider and Nahum (1972) Allsop et al. (1991) |
Occipital | 12.5 | Advani et al. (1982) |
As a result of extensive cadaver tests focusing on head acceleration, the Wayne State University Cerebral Concussion Tolerance Curve, abbreviated as the Wayne State Tolerance Curve (WSTC), was established (Gurdjian et al. 1953; Lissner et al. 1960; Gurdjian et al. 1966). The WSTC indicates a relationship between the duration and the average antero-posterior translational acceleration level of the pulse that accounts for similar head injury severity in head contact impact (Fig. 3.7). Clinically observed prevalence of concomitant concussion in skull fracture cases was used to relate cadaver impacts to brain injury. In fact, 80 % of all concussion cases also had linear skull fractures (Melvin and Lighthall 2002). Gurdjian and colleagues assumed that by measuring the tolerance of the skull to fracture loads, one is effectively inferring the tolerance to brain injury.
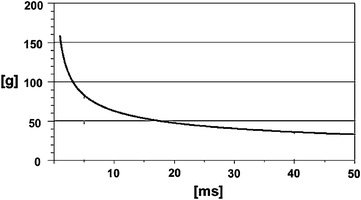
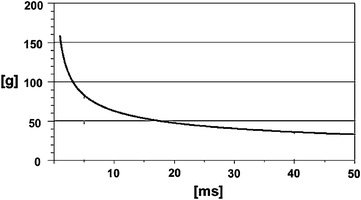
Fig. 3.7
The Wayne State Tolerance Curve (acceleration vs. duration of acceleration pulse) (adapted from Krabbel 1997)
Combinations of acceleration level and pulse duration that lie above the curve are thought to exceed the human tolerance, i.e. they cause severe, irreversible brain injury. Combinations below the curve do not exceed human tolerance, but may result in reversible injury. As the original WSTC covers a time duration range of 6 ms only, the curve was extended for durations longer than 6 ms using animal and volunteer data. Figure 3.7 presents the modified curve; the test conditions used to obtain the data are given in Table 3.3. As can be seen, the head can withstand higher acceleration for shorter durations.
Table 3.3
Test conditions of the experiments the WSTC is based upon
Pulse duration | Test objects | Test set-up | Response measured | Injury criterion |
---|---|---|---|---|
2–6 ms | Cadavers | Drop test | Acceleration at the back of the head | Skull fracture |
6–20 ms | Cadavers and animals | Impact test | Acceleration of skull, brain pressure | Pathological changes |
>20 ms | Volunteers | Sled tests | Whole body acceleration without head impact | Concussion, state of consciousness |
The WSTC is supported by experiments conducted in Japan which led to the Japan Head Tolerance Curve (JHTC) (Ono et al. 1980). JHTC was mainly obtained from experiments with primates and scaling of results to humans. Differences between the WSTC and JHTC are negligible for time intervals up to 10 ms, and only minor differences exist for longer durations.
When the WSTC is plotted in a logarithmic scale, it becomes a straight line with a slope of −2.5. Based on this finding, Gadd (1961) proposed a first head injury criterion, the severity index (SI). A modified form of this criterion is still in use today (see Sect. 3.4.1).
Using the WSTC or any criterion developed thereof, restrictions that arise from the test conditions have to be considered. The paucity of data points, the position of the accelerometer (back of the head), the fact that rotational acceleration is not considered, and the techniques used to scale the animal data are, for instance, major limitations. However, from a biomechanical point of view the main criticism concerns the correspondence of skull fracture and brain injury that was assumed. This hypothesis remains to be verified, as there was no direct demonstration of functional brain damage in an experiment in which biomechanical parameters sufficient to determine a failure mechanism in the tissue were measured (Melvin and Lighthall 2002).
Bearing in mind that the WSTC is based on direct frontal impact tests, the results can, strictly speaking, not be applied to non-contact loading conditions and to other impact directions, respectively. Nonetheless, WSTC is still the most important data source with respect to the linear acceleration response of the head.
Further experimental studies addressed rotational acceleration which may cause diffuse brain injury and subdural hematoma. Besides volunteers and cadavers, primates were subjected to head rotation, where the rotational acceleration was measured and the resulting degree of injury was assessed (e.g. Ommaya et al. 1967; Hirsch et al. 1968; Gennarelli et al. 1972). It was found that the angular acceleration and the according injury thresholds are related to the mass of the brain. Thus, the tolerance limit for the human was obtained by scaling the results from the primate tests (Fig. 3.8). Table 3.4 gives tolerance values that are commonly used. However, additional studies on volunteers suggest that much higher tolerance values up to 25000 rad/s2 may be possible for a short duration (Tarriere 1987).
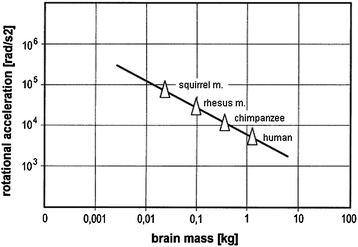
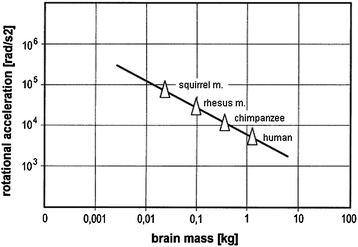
Fig. 3.8
Results from experiments and scaling addressing tolerance towards rotational acceleration (adapted from Krabbel 1997)
Table 3.4
Tolerance thresholds for rotational acceleration and velocity of the brain
Tolerance threshold | Type of brain injury | References |
---|---|---|
50 % probability: ![]() ![]() | Cerebral concussion | Ommaya et al. (1967) |
![]() ![]() | Rupture of bridging vein | Löwenhielm (1975) |
2000 rad/s2 < ![]() | Brain surface shearing | Advani et al. (1982) |
![]() AIS 5: ![]() ![]() AIS 2: ![]() AIS 3: ![]() AIS 4: ![]() AIS 5: ![]() | (General) | Ommaya (1984) |
In this section several experimental studies were presented that aimed at predicting head injury from one specific input parameter, i.e. the translational or the rotational acceleration, respectively. A more extensive review of these studies is given by Goldsmith and Monson (2005). However, in the vast majority of head impact situations it can be expected that both translational and rotational acceleration are present and combine to cause brain injury. Accordingly, comprehensive brain injury prediction requires taking into account the various responses of the brain tissue for any combination of mechanical loading. The development of sophisticated mathematical models of the head using, for example, the finite element method addresses this task and aims at determining measures for prediction of the head’s mechanical response to impact. When combined with results of detailed investigation of the response of the living human, such models promise to contribute substantially to today’s understanding of head injury mechanisms and the impact tolerance of the head.
3.4 Injury Criteria for Head Injuries
Although great progress in passive safety, such as the introduction of advanced restraint systems, was made in the last couple of years to reduce the number and severity of head injuries, there is only one injury criterion in wide use, the Head Injury Criterion (HIC). Besides the HIC and its European equivalent, the Head Protection Criterion (HPC), the “3 ms criterion” and the Generalised Acceleration Model for Brain Injury Threshold (GAMBIT) are presented. However, it should be noted that all these criteria are based on acceleration response only. Consequently, injuries that are related to impact force rather than acceleration are not addressed by these criteria. In other words, those criteria do not allow an evaluation of the injury risk of sustaining fractures of the bony structures of the head. The only dummy capable of measuring a force response to facial impact is the THOR dummy (see Sect. 2.6.1), but this dummy is not included in recent crash test standards. Further attempts on improving head injury criteria include a criterion based on the total change of kinetic energy of the head during impact (HIP, Newman et al. 2000), or employ e.g. finite element models to predict shear strain in the brain tissue, thus bypassing the discussion on whether rotational or translational acceleration is more important (Willinger and Baumgartner 2001; Takhounts et al. 2003, 2008; Patton et al. 2012). Such criteria, however, require extensive calculation and modelling steps after e.g. a crash test.
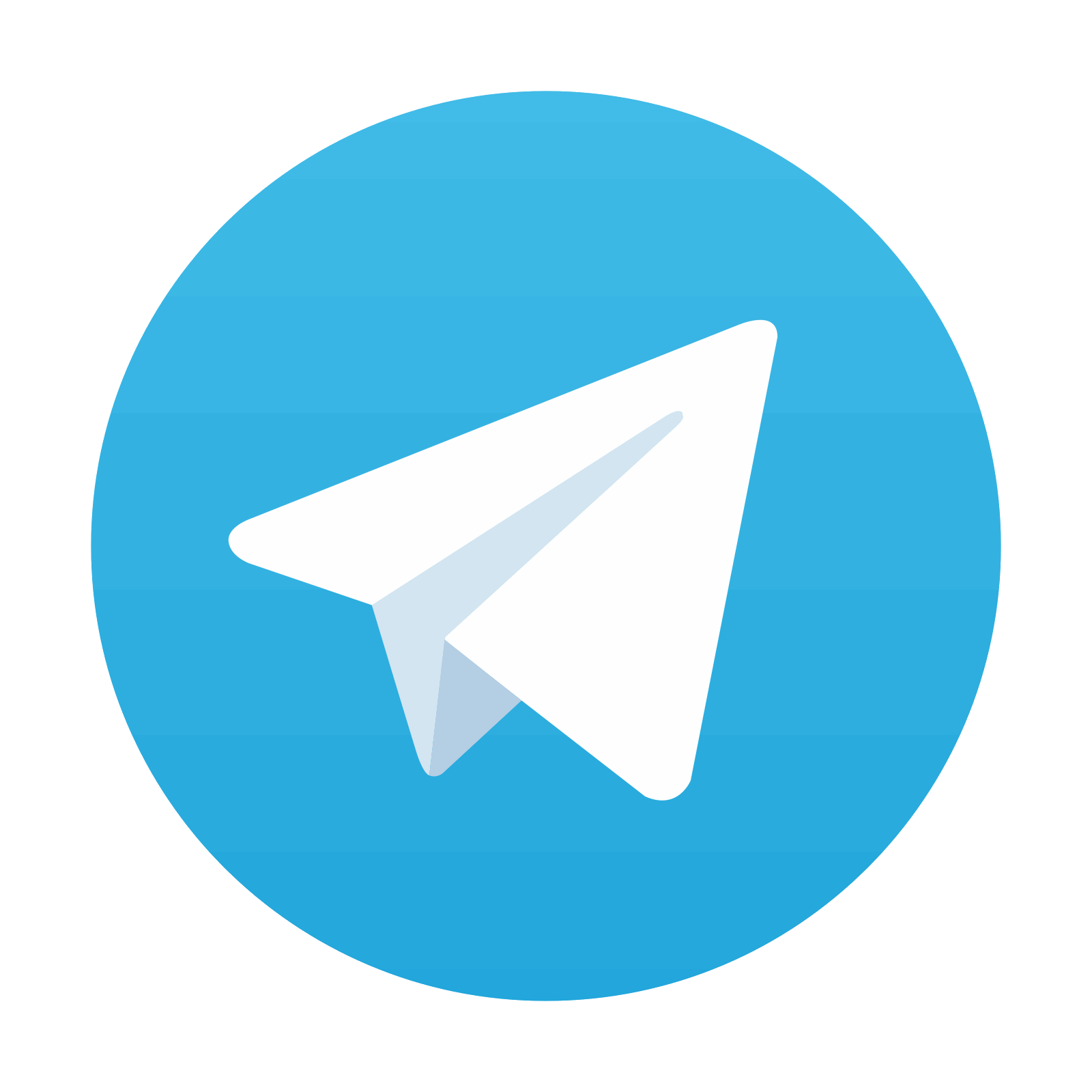
Stay updated, free articles. Join our Telegram channel

Full access? Get Clinical Tree
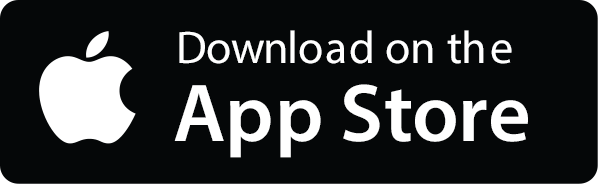
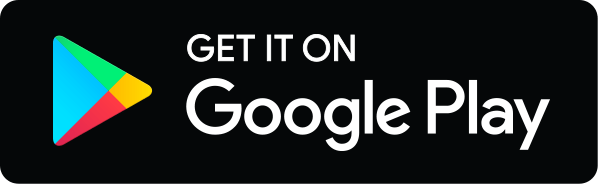