An acute epidural hematoma (EDH) is a hyperdense (higher Hounsfield unit number), extra-axial (outside the brain parenchyma) blood collection that occurs after a coup injury. The “classical” EDH occurs from a laceration of the middle meningeal artery after blunt trauma and is biconvex (lens-shaped) in appearance.
Hypodense blood within an EDH represents acute unclotted hemorrhage, while hyperdense blood corresponds to acute clotted hemorrhage. The mixed-density pattern, termed the “swirl sign,” is caused by clotted and unclotted blood products.
EDH is confined by dural attachments to the cranium, which occur at cranial sutures. EDH will cross the sagittal suture at the midline but subdural hematomas (SDH) will not, because the dura does not invest the superior sagittal sinus.
EDH in the middle cranial fossa anterior to the temporal lobe tips is due to venous injury of the sphenoparietal sinus.
EDH comprise 1 to 4% of traumatic brain injury (TBI) and most often occur from falls less than 10 ft or from assault with an object (coup injury). The classical clinical scenario, which occurs in 10% of patients with EDH, presents with loss of consciousness, followed by a period of lucency. Patients then become progressively obtunded as the EDH enlarges. EDH may expand rapidly, causing mass effect that can lead to herniation. Decompressive craniectomy is required in most patients with rapidly expanding EDH. Despite the possibility for rapid expansion with EDH, patients with EDH generally have a better prognosis than patients with SDH. EDH of the anterior temporal lobes is venous rather than arterial, and typically has a benign course and frequently does not require surgical evacuation.
Figure 1.6 ▪ Acute and Hyperacute EDH.


A, B: NECT shows a large lenticular mixed-density collection of blood over the left parietal lobe. There is midline shift and transfalcine herniation (left cingulate gyrus pushed beneath the falx cerebri, arrows). Mixed density of the blood indicates both hyperacute and acute blood products that portends a worse prognosis.
Noncontrast head CT is the preferred study to evaluate all acute TBI.
Greater than 90% of EDH have overlying skull fractures.
EDH is a coup lesion, with 85% caused by arterial injury.
The “swirl sign” is due to mixing of the acute clotted and acute unclotted blood in an EDH or SDH and carries a worse clinical prognosis.
Figure 1.7 ▪ Temporal EDH.

EDH overlying the left temporal lobe is caused by venous hemorrhage following disruption of the sphenoparietal sinus (arrow). Ipsilateral preseptal soft tissue swelling is characteristic. EDH in this location (anterior temporal) typically has a benign course. A contrecoup injury to the right posterior temporal lobe, with hemorrhagic contusion, is also present (arrowhead).
Figure 1.8 ▪ Skull Fracture.

Corresponding to Fig. 1.7, subtle fracture line is seen along the sphenoparietal suture on the left (arrow).
Figure 1.9 ▪ EDH and Swirl Sign.

Large epidural hematoma along lateral right temporal lobe. Mixed-density blood indicates hyperacute bleeding (“swirl sign”), which is a worse prognosis. The figure shows medial deviation of the uncus into the basilar cistern representing early transtentorial herniation (arrow).
A SDH is a crescent-shaped hemorrhage typically found along the convexities of the skull due to traumatic disruption of bridging cortical veins. In adults, SDH is not as often associated with overlying fracture as EDH. SDH will cross suture lines except at the falx, which is a dural reflection. Hemorrhage in the subdural space will invaginate with the falx, tracking between the frontal and parietal lobes.
Radiographic density varies with age of the blood products. Acute unclotted hemorrhage, also described as a hyperacute hemorrhage, is hypodense (0-2 hours); acute clotted hemorrhage is hyperdense (<1 week); a subacute SDH is isodense to brain (1-3 weeks); a chronic SDH appears hypodense (>3 weeks). Mixed densities within the SDH represent an acute bleed into a chronic SDH. The “hematocrit effect” is demonstrated when a sharp transition point is seen between the different densities in a SDH, which occurs most often when rebleeding occurs into a chronic SDH. The hyperdense acute blood will layer dependently. Patients with severe anemia can present with acute clotted SDH that appears hypodense. Septa within a SDH represent adhesions and make surgical drainage more difficult.
Detection of SDH is more sensitive with MRI than with CT, particularly for thin convexity SDH. The MRI appearance depends on age of the blood products.
SDH has much higher morbidity and mortality than EDH. In younger patients, SDH is most frequently due to rapid deceleration injury from a motor vehicle crash. While the slowly expanding hematoma can potentially contribute to secondary brain injury, it is the diffuse brain injury caused by the impact that creates the greatest morbidity. In contrast, seemingly minor trauma in elderly, alcoholic, or anticoagulated patients may cause SDH. Patients with SDH should be immediately evaluated by neurosurgery. Decompressive craniectomy may be required if patients are symptomatic.
Figure 1.15 ▪ Convexity and Parafalcine SDH.

Along the right cerebral convexity and falx cerebri are hyperdense extra-axial fluid collections that cross cranial sutures. Areas of heterogeneity in the fluid represent varying ages of the blood products (very high-density structure along the midline falx is normal dural calcification). Mass effect on the right cerebral hemisphere causes loss of the normal sulcation as the cortical gyri are compressed.
An MRI without contrast is better than CT at identifying hyperacute or chronic SDH. In these settings, SDH may be isodense to brain cortex on CT.
Radiographic features differentiating an EDH from SDH are seen in Table 1.1.
CT subdural window settings (window 150, level 5) are helpful for identifying subtle SDH. Subdural windows remove streak artifact that occurs from overlying bone.
Table 1.2 lists the CT window and level settings commonly used to evaluate the NECT of the traumatic brain.
Feature | EDH | SDH |
---|---|---|
Shape | Lenticular | Crescentic |
Origin | Arterial | Bridging vein |
Crosses midline | Yes | No, except in posterior fossa |
Coup injury | Yes | Yes |
Contrecoup injury | No | Yes—more common |
Associated fracture | >90% | Uncommon |
Crosses sutures | No | Yes |
Setting | Window | Level | Utility |
---|---|---|---|
Parenchyma | 80 | 40 | Standard brain evaluation |
Subdural | 150 | 5 | Improves detection of small SDH adjacent to bone |
Bone | 1500 | 450 | Pneumocephalus, fractures, sinuses |
Stroke | 30 | 30 | Ischemia/infarction |
Figure 1.16 ▪ Convexity and Parafalcine SDH.

SDH will not cross the falx cerebri, since the falx is formed by dural reflections at the midline. In this patient, SDH overlies the frontal convexities and has an interhemispheric component. The high attenuation of this SDH will last for a week, and then begin to darken as the SDH ages.
Figure 1.18 ▪ Acute Subdural Hematoma.




A 1-year-old female post injury. A: T1WI, B: T2*/GRE, C: FLAIR, and D: T2WI. On all images note the large right parietal scalp swelling. Best seen on the FLAIR and T1WI are bright fluid collections along the posterior and anterolateral right parietal lobe that represent blood. MRI is much more sensitive than CT for detection of SDH.
Figure 1.19 ▪ Acute Subdural Hematoma.

Large crescentic collection of acute blood along the right hemisphere representing an acute SDH. Note mass effect, dilation of the contralateral ventricle due to CSF outflow obstruction, and subfalcine herniation (arrow). Blood also extends into interhemispheric region.
tSAH is blood within the subarachnoid space that results from injury to small cortical vessels or as contiguous extension of a cerebral contusion, SDH, or EDH. Common locations for tSAH are basilar cisterns, the convexities, and the contrecoup Sylvian fissure. tSAH on unenhanced CT scan appears as curvilinear hyperdense areas that follow the cortical sulci. SAH is visible on CT for up to 1 week. tSAH of 1 to 2 mL, however, may only be picked up by MRI FLAIR, which is much more sensitive than conventional T2-weighted imaging for SAH. Patients with SAH are at risk for communicating hydrocephalus due to blocked arachnoid granulations (which absorb CSF).
tSAH is the most common CT abnormality in patients with moderate to severe TBI and is seen in 11% of TBI patients. Small-volume tSAH is inconsequential if confined to sulci of the convexities. If the tSAH involves ventricular system, mortality and morbidity substantially increases.
Figure 1.22 ▪ Ballistic Injury with tSAH.

NECT shows ballistic tract extending across right temporal lobe into basal ganglia. Metallic fragment seen in left posterior temporal lobe. SAH is present in the basilar cisterns, overlying the cerebellar tentorium, and scattered over the sulci. Ballistic injuries that cross the hemispheres have a worse prognosis.
Figure 1.26 ▪ Traumatic Subarachnoid Hemorrhage.


A, B: Faint hyperdensity over left frontal sulci represents SAH (arrow). Interesting to note is that these 2 images are exactly the same, except the second image is windowed differently with “subdural windows,” which accentuates discovery of the small subdural hematoma along the right frontal bone (arrow).
Once identified, tSAH requires neurosurgical consultation for definitive management.
tSAH can dissipate in 1 to 2 days due to high CSF turnover.
MRI FLAIR sequences suppress the T2 bright signal from CSF. Bright SAH will be contrasted against the darker surrounding cortex.
CT terminology describes acute clotted blood as hyperdense, high attenuation, or higher Hounsfield units. MRI terminology describes acute clotted blood as hyperintense or bright.
Figure 1.28 ▪ Traumatic Subarachnoid Hemorrhage.


A, B: Same patient as in Fig. 1.12: same-day MRI FLAIR imaging better demonstrates the SAH scattered over the left parietal lobe and a small parenchymal contusion (arrow). MRI (especially the FLAIR sequence) is much more sensitive than CT for SAH detection.
Increased cerebral volume results from increased cerebral blood volume (hyperemia) or increased tissue fluid (cerebral edema). Cerebral hyperemia is due to loss of cerebral autoregulation, and may progress to cerebral edema. Cerebral edema may be diffuse or focal. CT findings of diffuse cerebral edema include loss of gray-white attenuation and effacement of ventricles, sulci, or basilar cisterns.
Cerebral edema can occur from trauma, metabolic conditions such as DKA and hyponatremia, ischemic brain injury, tumors, rapid ascent to high altitudes, and intraparenchymal or extra-axial bleeding. Cerebral edema requires neurosurgical or neurological management of the underlying cause.
Cerebral edema may be vasogenic or cytotoxic. Vasogenic edema is primarily caused by increase in extracellular water from disruption of the blood–brain barrier, and is usually reversible. It primarily involves the white matter. Cytotoxic edema is due to cellular swelling with malfunction of ATP Na+/K+ pumps and classically seen with cerebral infarction. Cytotoxic edema is typically irreversible.
Traumatic cerebral edema tends to be diffuse. Focal edema tends to occur around areas of ischemia or tumor.
MRI is more sensitive than CT in detecting small areas of focal edema.
Figure 1.30 ▪ Loss of Gray-White Differentiation.


A, B: NECT at the vertex shows loss of the gray-white differentiation and diffuse cerebral edema. There is also loss of the normally seen sulci (although this can be difficult to detect in younger patients who have less CSF within their sulci compared with elderly patients).
Figure 1.32 ▪ White Cerebellum Sign.


A, B: Axial CT without intravenous contrast demonstrates diffuse supratentorial cerebral edema with resulting hypodensity of parenchyma, loss of gray-white matter differentiation, and effacement of sulci and basilar cisterns. Cerebellum looks “whiter” because it is not as edematous as cerebrum. This is a very poor prognostic sign as it indicates severe brain edema.
Acute hemorrhagic contusion appears as round or irregularly shaped hyperdensity within the brain parenchyma on noncontrast CT (NCCT) and occurs when the brain impacts the irregular inner table of the calvarium. Contusions always involve the cortical gray matter and may extend into the white matter. Common areas of contusion include frontal and temporal lobes. As contusions evolve, surrounding vasogenic edema becomes more pronounced, making the contusion more obvious on CT. Additionally, there may occasionally be “blossoming” of contusions on follow-up imaging as further hemorrhage occurs inside the contusion.
MRI is much more sensitive than CT to detect acute hemorrhagic and nonhemorrhagic contusions, especially along the convexity, where beam-hardening artifact limits CT. A chronic contusion may appear as a wedge-shaped area of enchephalomalacia.
Figure 1.34 ▪ Blooming of Contusion.

NECT 24 hours after Fig. 1.33 shows marked “blooming” or enlargement of the right frontal contusion with increased mass effect and edema.
All patients with hemorrhagic contusion require neurosurgical consultation. Hemorrhagic contusions in the temporal lobe are of particular concern because of the potential for uncal herniation if the contusion blossoms. Thus, patients with temporal lobe contusions are usually admitted.
Traumatic axonal injury (TAI) occurs from a shear-strain deformation of the brain, that is, a change in shape of the brain without change in volume that causes severe injury to axons. Imaging features depend on whether the TAI is nonhemorrhagic or hemorrhagic. Nonhemorrhagic shear comprises 80% of TAI injuries and will not be visible on NCCT. MRI is much more sensitive to nonhemorrhagic TAI, which will be visible in the acute phase on both FLAIR and DWI sequences. Several hours after injury, T2 hyperintensity is usually seen, most often in the frontal/parietal white matter. The corpus callosum is the next most common area affected. Involvement of the brainstem and cerebellar hemispheres suggests severe injury.
Hemorrhagic TAI may on NCCT appear as small collections of hyperdense fluid collections at the junction of the gray and white matter, typically in the frontal or temporal lobes. However, hemorrhagic TAI is best seen on susceptibility-weighted MR sequences, which are sensitive to blood breakdown products. These sequences include GRE/SWI/T2* (pronounced “tee-two-star”). Blood products create a “dropout” signal (dark black signal) in the areas of acute hemorrhage.
Location of the TAI determines the grade. Grade I TAI occurs at the gray-white matter junction. Grade II lesions (moderate TAI) involve lobar white matter and the corpus callosum, which is susceptible to shear due to its immobility relative to adjacent brain parenchyma. Grade III lesions (severe TAI) additionally involve the dorsolateral midbrain and upper pons.
The clinical manifestations of TAI range from postconcussive syndrome (headaches, light-headedness, inability to concentrate) to coma. MRI should be obtained in head trauma patients where CT is normal but symptoms are persistent.
Figure 1.40 ▪ Hemorrhagic TAI.

FLAIR MR image near the vertex shows several foci of white matter hyperintensity in the right frontal lobe (arrows) representing lobar axonal injury in this clinical setting. Note small amount of bright SAH near the left frontal precentral gyrus (arrowhead) (corresponding to CT in Fig. 1.37).
Figure 1.41 ▪ Hemorrhagic TAI.

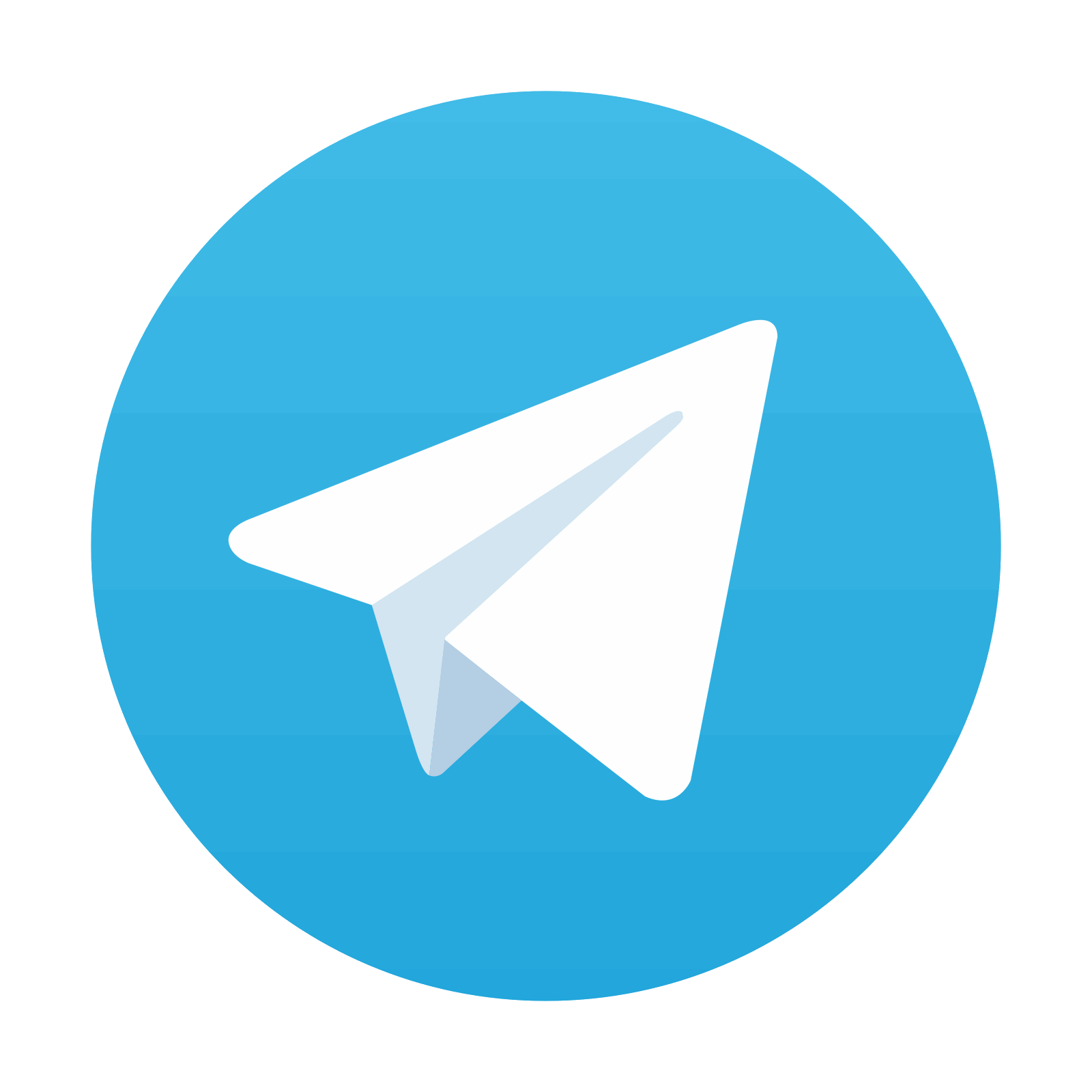
Stay updated, free articles. Join our Telegram channel

Full access? Get Clinical Tree
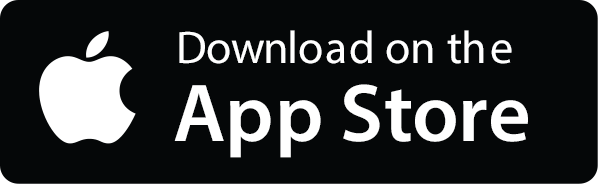
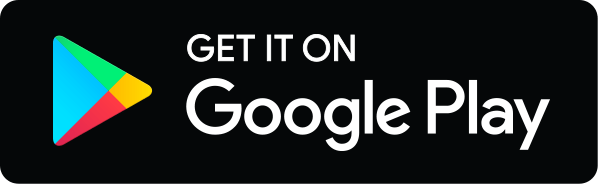
