Although each fracture requires individual evaluation and management, general principles of fracture assessment and fracture healing can be applied to aid providers in the proper care of patients with fractures. Accurate fracture identification is the first step in deciding whether to treat the fracture or refer the patient to a specialist. After carefully selecting which fractures to manage, the primary care provider can follow general guidelines for initial and definitive treatment, immobilization, and follow-up evaluation. Keeping in mind the different healing mechanisms and healing rates of various types of fractures also helps guide decisions about immobilization, duration of treatment, and radiographic follow-up.
Bone Composition
Bone consists of cells imbedded within an abundant extracellular matrix of mineral and organic elements. Mineral in the matrix lends strength and stiffness in compression and bending. The organic component, primarily type I collagen, gives bone great strength in tension. The outer covering of bone, the periosteum, consists of two layers—an outer fibrous layer and an inner more vascular and cellular layer. The inner periosteal layer in infants and children is thicker and more vascular and therefore is more active in healing. This difference partially explains why the periosteal reaction and callus formation after many pediatric fractures are more pronounced than those in adults.
Fracture Healing
Bone has the remarkable and unique ability to heal by complete regeneration rather than by scar tissue formation. Fractures in bones initiate a continuous sequence of healing that includes inflammation, repair, and remodeling. The inflammation phase is relatively short, constituting only about 10% of the total healing time. Bone repair continues for several weeks after the injury. Remodeling of bone begins before repair is complete and may continue for several months to years after a fracture.
Inflammation
Inflammation is the shortest phase of healing and begins immediately after injury. Release of chemical mediators, migration of inflammatory cells to the injury site, vasodilatation, and plasma exudation occur during this phase. Signs and symptoms include swelling, erythema, bruising, pain, and impaired function. After impact to the bone, a hematoma forms between the fracture ends and beneath the elevated periosteum. In a closed fracture, increased interstitial pressure within the hematoma compresses the blood vessels, limiting the size of the hematoma. Nevertheless, the bleeding associated with a closed fracture can still be substantial. For example, a closed fracture of the femoral shaft can result in up to 3 L of blood loss. Generally, open fractures result in much greater blood loss because the tamponade effect of the surrounding soft tissue is absent.
Repair
The bone reparative process is stimulated by chemotactic factors released during inflammation. Electrical stimuli may also play a role. As the inflammatory response subsides, necrotic tissue at the bone ends is resorbed. This resorption of 1 to 2 mm of the fracture ends makes fracture lines more distinct radiographically 5 to 10 days after injury. Fibroblasts appear and start building a new reparative matrix. The fracture hematoma provides a fibrin scaffold for the formation of the fracture callus. The new tissue that arises, the soft callus, is primarily cartilage and acts to stabilize and bridge the fracture gap. As new blood vessels develop that supply nutrients to the cartilage, immobilization of the fracture site is desirable during this phase to allow for revascularization. Bone begins to replace the cartilage approximately 2 to 3 weeks after injury, forming a hard callus. This process continues until continuity is reestablished between the cortical bone ends.
Mineralization of the fracture callus by chondrocytes and osteoblasts mimics similar events in the normal growth plate. As mineralization proceeds, stability of the fracture fragments progressively increases, and eventually clinical union occurs. Clinical union is demonstrated by lack of movement or pain at the fracture site and radiographs showing bone crossing the fracture site. At this stage, fracture healing is not yet complete. The fracture callus is weaker than normal bone and regains full strength only during the remodeling process.
Remodeling
The final phase of fracture healing begins approximately 6 weeks after the injury. During the repair phase, woven bone is deposited rapidly and has an irregular pattern of matrix collagen. Remodeling reshapes the repair tissue by replacing irregular, immature woven bone with lamellar or mature bone and by resorbing excessive callus. Osteoclasts resorb unnecessary or poorly placed trabeculae and form new bony struts oriented along the lines of stress. Although most remodeling that is apparent on plain radiographs ceases within months of injury, removal and reorganization of repair tissue may continue for several years. Bone scans will continue to show increased uptake at the fracture site during this lengthy period of remodeling.
Factors That Influence Fracture Healing
Fracture healing is a complex process and can be influenced by a number of injury, patient, and treatment factors. Severe injuries with significant soft tissue and bone damage, open fractures, segmental fractures, inadequate blood supply, and soft tissue interposition adversely affect healing. Fracture healing ranges from rapid and complete to delayed or incomplete. When fracture healing progresses more slowly than usual, it is referred to as delayed union . When the healing process is arrested, a nonunion occurs, and a pseudarthrosis or fibrous tissue that does not progress to complete healing forms at the fracture site. Intraarticular fracture healing may be delayed because of excessive motion of fracture fragments or synovial fluid collagenases that weaken the fracture callus. Because of this, intraarticular fractures must be in excellent alignment and sufficiently stabilized to reduce the possibility of poor healing.
Age is one of the most important factors that influence bone healing. Whereas children’s fractures heal rapidly, fractures heal much more slowly in older persons. Hormonal factors also affect healing. Growth hormone, thyroid hormone, insulin, calcitonin, cortisol, anabolic steroids, and gonadal steroids all play roles. Fractures in patients with a hormonal imbalance generally heal, although union may be delayed. Nutritional factors are also important in the healing process. An adequate balanced diet and sufficient amounts of vitamin D and vitamin C are essential for normal fracture healing. Conditions that compromise fracture healing include diabetes, hypothyroidism, excessive chronic alcohol use, and smoking. Corticosteroids compromise fracture healing, and patients who use steroids on a long-term basis are at increased risk of fractures because of the increased risk of osteoporosis. A causal relationship between nonsteroidal antiinflammatory drugs (NSAIDs) and an increased risk of nonunion has not been established despite some reports of an effect on fracture healing.
The treatment factors that promote bone healing include adequate fragment apposition, weight bearing or fracture loading, and proper fracture stabilization. For most fractures, inappropriate or ineffective stabilization slows healing and may lead to nonunion. Some fractures heal well even though the fracture remains mobile until callus forms. This is true of clavicle, some metacarpal, and many humeral shaft fractures.
Potential Fracture Sites
Identifying the specific location of the fracture within a bone is the first step in the proper evaluation of fractures. In a skeletally mature adult, fractures may occur in the diaphysis (e.g., shaft of long bones) or in the metaphysis (e.g., neck of long bones or short, flat bones) or may extend into the joint (intraarticular). Fractures in children may also involve the growth plate (physis) or the epiphysis. Fig. 2-1 shows the potential fracture locations in adult and growing bone.

Bone tissue is of two types: cortical or compact bone and cancellous or trabecular bone. The diaphysis is made up mostly of solid, hard, cortical bone. Metaphyseal bone consists of a thin shell of cortical bone surrounding primarily spongy, cancellous bone. Differences in the distribution of cortical and cancellous bone in various locations result in differences in healing mechanisms and rates.
In a diaphyseal fracture with minimal separation in cortical bone, healing occurs by formation of callus that progressively stabilizes the fracture fragments. In shaft fractures that require surgery and rigid internal fixation, healing can occur without callus formation. In this type of healing (called primary bone healing ), the bone surfaces are in direct contact, and lamellar bone forms directly across the fracture line. In cancellous bone, which consists of a labyrinth of trabeculae lined by osteoblastic cells, new bone is created in all areas after a fracture. Healing in cancellous bone is usually much more rapid and complete than cortical bone healing, but it is more difficult to evaluate radiographically because it does not produce an external callus.
Fracture Description
The management of fractures begins with proper identification and description, including fracture location, fracture type, and the amount of displacement. Learning to describe fractures accurately and precisely is essential for primary care providers. Effective communication with consultants who provide advice over the telephone or receive the patient in referral is difficult without this skill.
Fracture Type
Many terms are used to describe fractures. Using precise language and avoiding vague terminology help ensure proper treatment, especially when the primary care practitioner is relying on telephone advice. Fracture type includes description of the direction of the fracture line, the number of fragments, and the injury force applied to the bone. A transverse fracture has a fracture line oriented perpendicular to the long axis of the bone. Fracture lines can be transverse, oblique, or spiral. A true spiral fracture involves a fracture line that traverses in two different oblique directions. A long oblique fracture line is often mistakenly called a spiral fracture. Both of these fracture types are relatively unstable and can result from a rotational force applied to the bone. An intraarticular fracture extends into the joint space and is typically described in relation to the percentage of the joint space that is disrupted. A comminuted fracture has multiple fragments, and a segmental fracture is a type of comminuted fracture in which large well-defined fragments occur. Radiographic examples of these fracture types are shown in Figs. 2-2 to 2-6 .





Other terms used to describe fracture types relate to the deforming forces applied to the fracture fragments ( Fig. 2-7 ). In an impacted fracture, a direct force applied down the length of the bone results in a telescoping of one fragment on the other. An avulsion fracture occurs after a forceful contraction of the muscle that tears its bony attachment loose. Compression fractures are common in cancellous flat bones because they are spongy. A pathologic fracture occurs at the site of bone weakened by tumor or osteoporosis. A stress fracture results from chronic or repetitive overloading of the bone ( Fig. 2-8 ).


The fracture types unique to growing bone are torus (buckle), greenstick, and plastic deformation. These are discussed in the Pediatric Fracture section at the end of this chapter.
Fracture Displacement
Fracture displacement occurs when one fragment shifts in relation to the other through translation, angulation, shortening, or rotation. In general, displacement is described by referring to the movement of the distal fragment relative to the proximal fragment. Translation can occur in either the anteroposterior (AP) plane or the medial-lateral plane. In the description of displacement of hand and wrist fractures, the terms volar and dorsal are commonly used instead of anterior and posterior , and ulnar and radial are used instead of medial and lateral . In addition to a description of the direction of translation, the amount of translation should be reported. This can be measured on the radiograph in millimeters, or the percentage of apposition can be estimated ( Fig. 2-9 ). Generally speaking, 3 mm or less of translation is considered “minimally displaced.”

Angulation at the fracture site may be in the frontal or sagittal plane or both. True AP and lateral radiographs, at 90 degrees from each other, are necessary to accurately estimate angulation of a fracture. Angulation cannot be assessed from an oblique film. In the description of angulation, the direction in which the apex of the angle (i.e., the point of the “V” formed by the angulated fragments) is pointing should be stated. Fig. 2-10 is an example of apex medial angulation. Fig. 2-11 demonstrates apex dorsal angulation. The amount of angulation is measured in degrees with the aid of a goniometer ( Fig. 2-12 ).



Shortening of the bone is another type of displacement. A change in bone length occurs in an impacted fracture or in bayonet-type apposition. Fractures vary as to how much shortening is acceptable for proper healing. The deforming forces of trauma, gravity, or muscle pull can cause rotational displacement of fracture fragments. Rotation is difficult to visualize radiographically and is more often detected clinically ( Fig. 2-13 ).

Radiographic Interpretation
Using proper terminology as already described leads to accurate and clear descriptions of radiographs. Description of the radiographic findings of a fracture should identify the following aspects: the bone involved, the location of the fracture, the type of fracture, and the amount of displacement. Noting whether a fracture is diaphyseal or metaphyseal helps with decisions that affect healing. Other terms used to describe the location of a fracture within a bone include proximal or distal ; medial and lateral ; and head , neck , shaft , or base .
In the radiograph in Fig. 2-14 , the fracture would be accurately described as a nondisplaced, nonangulated oblique fracture of the left distal fibula (or distal fibula metaphysis). Examples of other fractures and corresponding radiographic interpretations are presented in Figs. 2-15 to 2-17 .




Fracture Selection
In the approach to a patient with a newly diagnosed fracture, emphasis should be placed on identifying patients who need prompt treatment and those who can have a splint applied and receive definitive treatment later. Primary care providers can manage a wide range of fractures and achieve good clinical results if they carefully select which fractures to manage based on general guidelines.
Referral Decisions
This decision is influenced by the nature of the fracture, the presence or absence of coexistent injuries, the characteristics of the patient, local practice patterns, and the expertise and comfort level of the primary care provider. The following guidelines can be used in making decisions regarding orthopedic referral:
- 1.
Avoid managing any fracture that is beyond your comfort zone unless a more experienced provider is available to guide your management. The comfort of both the patient and the provider is often enhanced if the provider explains his or her experience with fracture management and lets the patient choose between referral and continuing under his or her care.
- 2.
Identify patients with complicated fractures.
- 3.
Strongly consider referring any patient who is likely to have difficulty complying with treatment.
Complicated Fractures Requiring Urgent Action or Consultation
A minority of fractures are complicated by conditions that require urgent action. The key to the management of these conditions is early recognition followed by prompt definitive treatment.
Life-Threatening Conditions
Fortunately, life-threatening conditions are rare. When they do occur, they are almost always associated with major trauma or open fractures. Life-threatening conditions that may occur with fractures include hemorrhage, fat embolism, pulmonary embolus, gas gangrene, and tetanus.
The most common life-threatening condition associated with fractures is significant hemorrhage. Half of all pelvic fractures cause blood loss sufficient to require transfusion, and significant hemorrhage frequently occurs with closed femur fractures.
Fat embolism is much less common. It is usually associated with long bone or pelvis fractures in young adults or hip fractures in elderly adults. It generally develops 24 to 72 hours after the fracture, and symptoms include a classic triad of hypoxemia, neurologic impairment, and a petechial rash. In the early stages, fat embolism may be difficult to distinguish from acute lung injury (ALI) or acute respiratory distress syndrome (ARDS). After respiratory distress occurs, patients develop confusion and an altered level of consciousness. The characteristic petechial rash, which is the last of the triad to develop and present in less than half the cases, is caused by the occlusion of capillaries by fat globules and is found on the head, neck, trunk, subconjunctiva and axillae. Overall mortality ranges from 5% to 15%.
Patients with fractures are predisposed to venous thrombosis and therefore pulmonary embolism. Immobilization of limbs, decreased activity levels, and soft tissue injury contribute to the increased risk of venous thrombosis. Gas gangrene after a fracture is almost always associated with injuries that penetrate to muscle. This infection is marked by pain and wound drainage and typically progresses rapidly to local spread, toxemia, and death. Tetanus may also occur after open fractures and may involve local or generalized muscle spasm and muscle hyper-irritability.
Arterial Injury
Only a small percentage of fractures involve arterial injuries. However, such injuries can produce disastrous outcomes, such as loss of a limb or permanent ischemic contracture. Fortunately, when recognized early and treated appropriately, arterial injuries usually have a good outcome. Arterial injuries are most common in dislocations, fractures with penetrating injuries (e.g., gunshot wounds), and fractures of certain sites. Arterial injury commonly accompanies displaced fractures and dislocations of the elbow and knee. A displaced supracondylar fracture is shown in Fig. 2-18 . In such injuries, the proximal fragment often causes kinking and occlusion of the brachial artery ( Fig. 2-19 ). When evaluating a supracondylar fracture, the physician should presume that an arterial injury is present until proved otherwise. Arterial injuries should be suspected in any patient with a knee dislocation. Some institutions routinely perform arteriograms on all knee dislocations regardless of a normal circulatory examination to detect initially asymptomatic intimal tears that can go on to develop complete occlusion.


Primary care providers can minimize the adverse outcomes of arterial injuries by following these guidelines:
- 1.
Assess circulation distal to the injury in all patients with a fracture or dislocation . This is most often done by assessing the presence and strength of distal pulses. Slow capillary refill (greater than 3 seconds) and pallor are signs of arterial injury.
- 2.
Assess circulation as soon as possible after the patient seeks treatment. Ideally, this would be done within minutes of an initial examination before radiographs are ordered. Signs of disrupted blood flow include skin mottling, a cool extremity, and decreased sensation.
- 3.
When dislocations and displaced fractures are accompanied by an absent distal pulse and orthopedic assistance is not readily available, primary care providers should attempt such reductions promptly. In many cases, kinking of the artery rather than actual arterial injury impairs circulation. If the limb is pulseless, much is to be gained and little lost by attempted reduction. If no one with experience in reductions is available soon after radiographs are obtained, one or two gentle reduction attempts by the primary care provider would be appropriate. The reduction technique would differ from those of other reductions in which it is desirable to first reproduce the force that created the fracture, briefly exaggerating the deformity. In these cases, such a maneuver could cause additional vascular damage and should be avoided if possible.
- 4.
Repeat the vascular examination after any manipulation of the fracture site and whenever symptoms that suggest possible ischemia develop. Symptoms suggestive of limb ischemia include disproportionate pain, especially if limb immobilization and appropriate analgesia fail to relieve the pain. Ideally, ischemia would be detected at this early stage before permanent damage occurs. If ischemia is suspected, consultation should be considered even if pulses are present (see Compartment Syndrome , discussed later).
- 5.
Document all vascular examinations in the medical record.
Nerve Injury
Many patients have mild paresthesia directly over the fracture site. This is most likely caused by local soft tissue edema. This paresthesia is benign and self-limited. Proximal humerus fractures, however, are an exception. Whereas most injuries to other nerves involve impaired nerve function distal to the fracture, paresthesia overlying the deltoid in these cases may actually indicate injury to the axillary nerve. For detection of nerve injuries, distal sensation should be assessed and documented in all fracture patients. When feasible, motor function should also be assessed, especially when a sensory deficit is suspected.
Nerve injuries are most common when a penetrating injury is present and in fractures near the elbow and knee. Dislocations of the hip, knee, and shoulder also have a high incidence of nerve injury. Most nerve injuries associated with fractures and dislocations are temporary neurapraxias caused by nerve stretching and resolve spontaneously within 2 to 3 months. Nerve injuries associated with penetrating injury, open fracture, or complete loss of nerve function are likely to be more serious and require orthopedic referral. Open exploration and nerve repair are often necessary in such cases.
Compartment Syndrome
Compartment syndrome results when the pressure within a rigid fascial compartment prevents adequate muscle perfusion. It is most common in tibial and forearm fractures, where several such compartments exist. Soft tissue swelling within the fixed compartment causes increased pressure. As the pressure within the compartment increases, perfusion becomes impaired, leading to muscle ischemia and further swelling. Myonecrosis, ischemic nerve injury, and occlusion of arterial flow then occur. If left untreated, permanent ischemic contracture or loss of the limb may result.
The symptoms and signs of compartment syndrome appear in a step-wise manner and are variable, so serial examination is important. Pain is the most reliable early symptom, but it is not present in all cases. Typically, pain caused by compartment syndrome is disproportionate, deep, and poorly localized (analogous to the pain of cardiac ischemia). The presence of such pain, especially in a high-risk situation (e.g., crush injuries, fractures of the lower leg or forearm, and acute fractures treated with a circumferential cast), strongly suggests a compartment syndrome. Paresthesia of the sensory nerve that passes through the compartment is another relatively early sign. Other symptoms and signs, that may or may not be present, include pain that increases with passive stretch of the affected muscle, a firm “wood-like” feeling to the limb, and muscle weakness. When paralysis and pulselessness are present, permanent damage, loss of the limb, or both are likely.
To detect compartment syndrome early, the clinician must remain extremely alert to its potential occurrence, be aware of the early symptoms, and pursue further evaluation with compartment pressure measurement and orthopedic consultation in suspected cases. Interpreting the results of compartment pressure measurements can be complex because accuracy depends on proper calibration of the device, and needle placement and the pressure necessary to cause injury vary depending on the clinical scenario. A difference between the diastolic blood pressure and the compartment pressure of 30 mmHg or less is a commonly accepted threshold for an elevated compartment pressure. Fasciotomy to decompress the affected compartments is the definitive treatment in the vast majority of cases.
Open Fractures
Open fractures are those in contact with the outside environment and require emergent orthopedic referral for irrigation, surgical debridement, and treatment with intravenous antibiotics to minimize complications. In obvious open fractures, exposed bone is clearly seen. In many open fractures, however, the bone edges pull back after breaking the skin and are no longer visible. To avoid missing these injuries, the clinician should suspect an open fracture whenever a break in the skin overlies a fracture. An open fracture exists whenever the hematoma around the bone ends communicates with the outside environment. Any break in the skin near a fracture should be carefully inspected and, if necessary, gently explored. Concern that the wound may communicate with the fracture hematoma warrants orthopedic consultation. Management of open fractures depends on the extent of soft tissue damage, the degree of wound contamination, and the overall health of the patient. Although they technically meet the definition of an open fracture, fractures of the distal phalanges with minor adjacent lacerations or nail bed injuries do not require emergent treatment by an orthopedist and can be managed by primary care providers.
Tenting of the Skin
Occasionally, severely angulated or displaced fractures produce enough pressure on the overlying skin to cause the skin to become ischemic. In such cases, the skin appears blanched and taut. If the pressure continues, the skin ultimately breaks down, converting a closed fracture into an open one. Prompt reduction is necessary in these situations. In most of these cases, delaying the reduction for 10 to 20 minutes while obtaining adequate anesthesia or orthopedic consultation does not adversely affect the outcome.
Significant Soft Tissue Damage
Some fractures are accompanied by severe injury to the adjacent muscle and skin. These injuries are prone to the development of compartment syndrome, infection, skin breakdown, and other complications. When considerable soft tissue damage is present, managing the soft tissue injury may be more difficult than managing the fracture itself. Early orthopedic consultation is recommended in such injuries to optimize management and prevent complications. Soft tissue damage of this extent is generally seen only in crush injuries.
Complicated Fractures That Often Require Referral
In addition to the injuries discussed earlier, other types of complicated fractures are likely to necessitate referral. These include fractures requiring reduction, multiple fractures, intraarticular fractures, fracture dislocations, epiphyseal plate fractures, and fractures with associated tendon injury. The referral rate for each type is highly dependent on the training and experience of the provider. Referral rates for complicated fractures managed in rural settings are shown in Table 2-1 .
patients managed by family physicians only | patients managed by family physicians with consultation | patients referred | total number (% of all fractures ) | |
---|---|---|---|---|
Displaced fractures requiring reduction * | 12 | 0 | 24 † | 35 (12) |
Multiple bones fractured | 27 | 0 | 8 | 35 (12) |
Intraarticular | 3 | 1 | 8 | 12 (4) |
Fracture-dislocation | 0 | 0 | 11 | 11 (4) |
Open fracture | 2 ‡ | 0 | 9 | 11 (4) |
Epiphyseal plate fracture | 2 | 3 | 5 | 10 (3) |
Associated tendon injury | 1 | 2 | 3 | 6 (2) |
Possible nerve injury | 0 | 1 | 1 | 2 (1) |
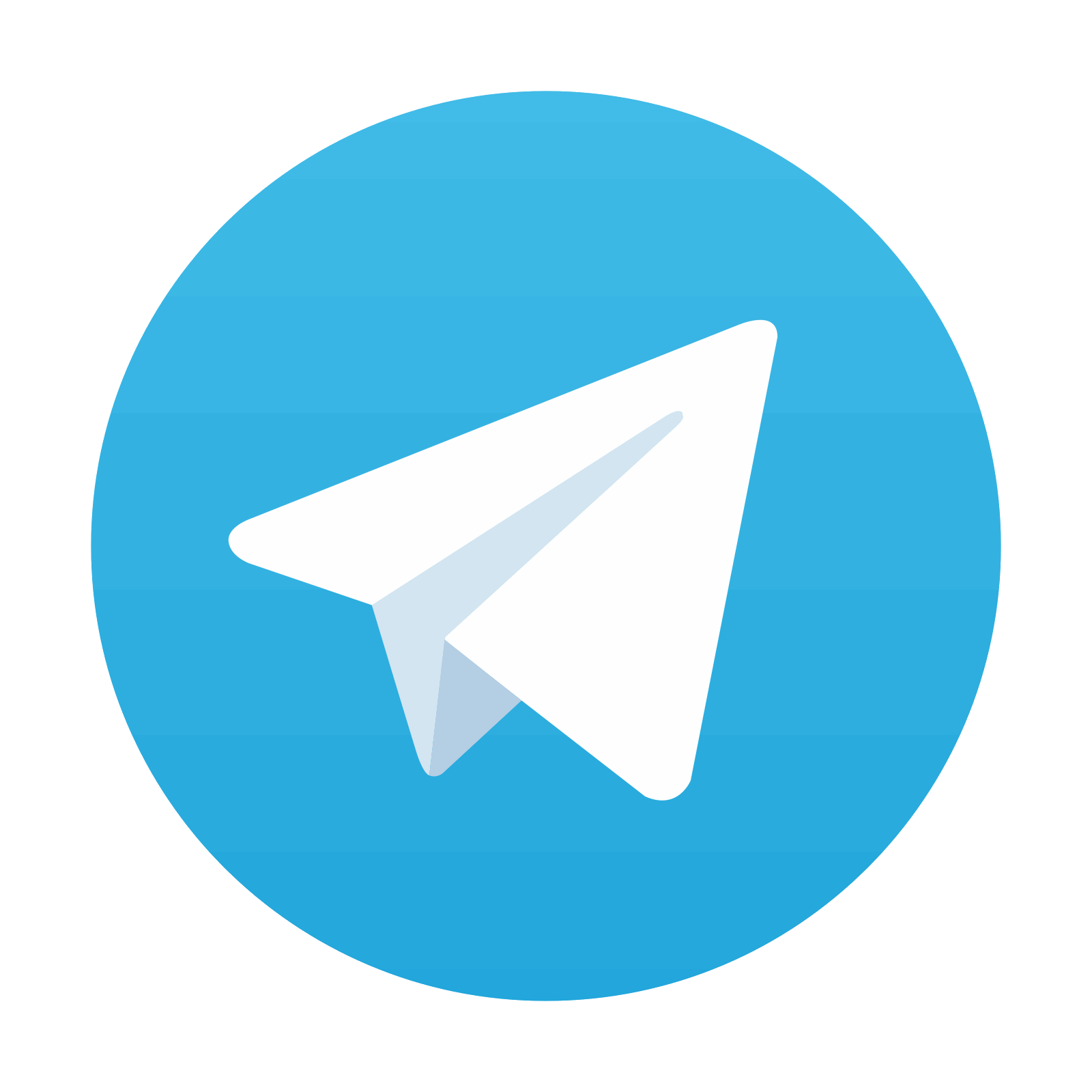
Stay updated, free articles. Join our Telegram channel

Full access? Get Clinical Tree
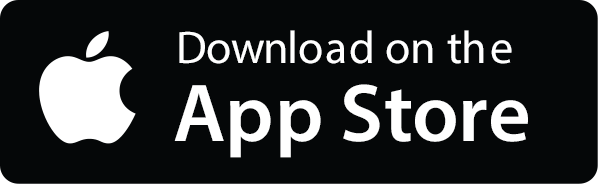
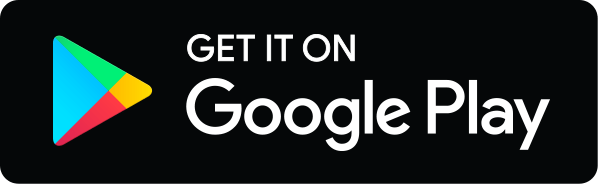
