General Considerations in the Evaluation and Treatment of Poisoning
Ian M. Ball
Christopher H. Linden
The objective of this chapter is to provide the general intensivist with both an overview and an approach to the management of the critically ill poisoned patient. General concepts germane to the intensive care unit (ICU) will be introduced and explored. Every attempt has been made to be as evidence based as possible, within the intrinsic limitations of the medical toxicology literature.
Because overdose studies cannot ethically be performed in humans and animal data may not be available or applicable to humans, predicting the severity of poisoning must be based on toxicodynamic data from previously published reports of human poisonings. However, such data are often incomplete or altogether unavailable and are always limited by the accuracy of the overdose history.
Poisoning or intoxication is defined as the occurrence of harmful effects resulting from exposure to a foreign chemical or xenobiotic. Such effects may be local (i.e., limited to exposed body surfaces), subjective (i.e., symptoms only) or systemic and objective (e.g., behavioral, biochemical, cognitive, or physiologic). In the absence of signs or symptoms, external or internal body contact with a potentially harmful amount of a chemical is merely an exposure. An overdose is an excessive exposure to a chemical that in specified (e.g., therapeutic) amounts is normally intended for human use. Whether an exposure or overdose results in poisoning depends more on the conditions of exposure (primarily the dose) than the identity of the agent involved. Ordinarily safe chemicals, even those essential for life such as oxygen and water, in excessive amounts or by an inappropriate route can result in harmful effects. Conversely, by limiting the dose, chemicals usually thought of as poisons can be rendered harmless. Poisoning is distinguished from adverse allergic, intolerance, and idiosyncratic pharmacogenetic reactions in that effects are concentration or dose related and, hence, predictable. As such, it includes adverse drug reactions due to unwanted secondary effects and pharmacokinetic and pharmacodynamic interactions.
Poisonings, exposures, and overdoses may be characterized by the route, duration, and intent of exposure. Ingestion, dermal or ophthalmic contact, inhalation, and parenteral injection (including bites and stings) are the most common routes, but rectal, urethral, vaginal, bladder, peritoneal, intraocular, and intrathecal exposures can also occur. Events that occur once or during a short period of time are considered acute, whereas those that occur repeatedly or over a prolonged time interval are said to be chronic
Epidemiology
Although comprehensive data regarding the true incidence of poisoning are not available [1], it is clearly a significant medical problem. Just under two and a half million human exposures were reported to the National Poison Data System in 2007 [2]. Of these, 20% to 25% are treated at a health care facility, and approximately 6% are admitted to a hospital. Half of those admitted are treated in an ICU. In other countries, the ICU admission rate for those evaluated at a health care facility varies from 5% to 22% [3,4,5].
Exposures and poisonings are responsible for 1% to 5% of emergency department visits, 5% to 10% of all ambulance transports, 5% to 14% of adult ICU admissions, and 2% to 5% of pediatric hospital admissions [3,4,5,6,7,8,9]. In addition, 25% of routine medical admissions involve some form of drug-related adverse patient event (an adverse drug reaction or noncompliance), and up to 30% of acute psychiatric admissions are prompted by attempted self-harm via chemical exposure. Although the incidence of poisoning in children has decreased since the introduction of the Poison Prevention Packaging Act in 1970 [10], the overall incidence of poisoning is increasing, particularly that due to suicide attempts in teens, middle-aged adults, and the elderly. The volume of calls handled by United States Poison Centers increased by 7.6% in 2007 [2]. Poisoning is second only to firearms as the leading cause of suicide [5]. Poisoning is the second leading cause of injury death [2]. The yearly medical cost for the treatment of poisoning in the United States is estimated to be $26 billion [11]. Poisoning accounts for 6% of the economic costs of all injuries in the United States [11].
Most exposures reported to US poison centers are acute (90.9%), unintentional (83.2%), occur at home (92.9%), cause minor or no harmful effects (95%), result from ingestion (78.4%), and involve children 6 years of age or younger (51.2%) [2].
Poisoning accounts for 2% to 14% of all ICU admissions, with an average length of stay of about 3 days [3,5,7,8,9]. The mortality rate for such patients varies from 0.6% to 6.1% [3,4,6,7,8,9]. Although only 1,239 poisoning fatalities were reported by US poison centers in 2007 [2], death certificate data indicate that the true number of poisoning deaths is 20 to 50 times higher [12]. Poison center statistics vastly underestimate mortality from poisoning because they rarely capture cases in which the victim is found dead and goes directly to the medical examiner.
Pharmacologic Concepts
Toxic exposures all undergo the same pharmacologic steps, as outlined in Table 117.1. Clinician familiarity with toxicokinetics is essential for predicting the effect of a particular exposure and guiding appropriate treatment and disposition. Only a
brief overview of these concepts is presented here. The reader is referred to other sources for additional information [13,14,15,16,17]. Details regarding the disposition and toxic effects of specific agents can be found in subsequent chapters and other references [14,15,16,17,18,19,20,21,22,23,24,25,26,27].
brief overview of these concepts is presented here. The reader is referred to other sources for additional information [13,14,15,16,17]. Details regarding the disposition and toxic effects of specific agents can be found in subsequent chapters and other references [14,15,16,17,18,19,20,21,22,23,24,25,26,27].
Table 117.1 Toxicokinetic Stages | |
---|---|
|
Mechanism of Action
Most chemicals are absorbed and cause systemic poisoning by selectively binding to and disrupting the function of specific targets (e.g., enzymes, proteins, membrane lipids, or neurohumoral receptors). Effects may be systemic or limited to a specific organ or tissue, depending on the distribution and location of target site.
Poisoning is usually functional and reversible. Hence, if end organ function can be supported, complete patient recovery is possible upon toxin elimination. However, if normal activity of the target site is essential for cell viability, a toxic exposure may result in necrosis. Agents that can cause fatal cellular damage include acetaminophen, carbon monoxide, corrosives, toxic alcohols, heavy metals, and neurotoxic hydrocarbons.
Absorption
Absorption involves the translocation of chemicals across the membranes of cells that make up mucosal surfaces, pulmonary epithelium, and skin, all of which function as biologic barriers. Translocation occurs by filtration or passive diffusion through gaps or membrane pores by dissolving in and diffusing through the membrane itself (e.g., lipid-soluble chemicals), or by attaching to carrier molecules in the membrane, which actively or passively facilitate diffusion (e.g., water-soluble chemicals). The rate and extent of absorption depend on physical properties of the chemical and the route of exposure. In general, only chemicals that are small (i.e., < 4 nm in diameter), have low molecular weight (i.e., < 50 Da), and are soluble in both water and lipids at the pH of body fluids can readily cross membranes.
Absorption after intravenous injection is complete and almost instantaneous. Peak arterial and venous blood concentrations occur within 30 to 90 seconds. Most toxins cross biologic membranes by simple passive diffusion. The rate at which this occurs is governed by Fick’s law of diffusion.

where D is the diffusion constant (constant for each toxin), A is the membrane surface area, K is the partition coefficient (represents the lipid: water partitioning of the toxin), d is the membrane thickness, and C is the toxin concentration.
Pulmonary absorption is rapid but incomplete. Blood concentrations peak within seconds to minutes. The absorption of chemicals after intramuscular or subcutaneous injection is slower but relatively complete. Peak blood levels generally occur within an hour of administration. Poor water solubility (low K) is responsible for the slow absorption and long duration of action of intramuscular depot formulations (e.g., neuroleptics).
The rate and extent of absorption after ingestion are variable. Peak blood levels are typically noted within 0.5 to 2.0 hours of a therapeutic dose. The absorbed dose is proportional to, but not necessarily equal to, the administered dose. The rate and extent of absorption after contact with other mucosal surfaces (e.g., oral, nasal, ophthalmic, rectal) is similar to ingestion. Skin absorption, if it occurs at all, is usually considerably slower. Regardless of route, absorption tends to follow first-order kinetics (i.e., the amount of chemical absorbed per unit of time is directly proportional to its concentration). Hence, threshold tissue concentrations are usually reached more quickly and effects begin sooner after an overdose than after a therapeutic dose.

The dissolution and solvation of particulate material is often a rate-limiting step in gastrointestinal (GI) drug absorption. Hence, pill, solid, and suppository formulations tend to be absorbed more slowly than liquids, powders, or suspensions. Slow dissolution and solvation also account for the delayed and prolonged absorption of enteric-coated tablets (e.g., aspirin, potassium), sustained-release preparations (e.g., cardiovascular drugs, lithium, phenytoin, theophylline), drugs that tend to form concretions (e.g., ethchlorvynol, glutethimide, heavy metals, iron, lithium, and meprobamate), and those with poor water solubility (e.g., carbamazepine and digoxin). The rate of dissolution is also inversely related to the tablet concentration. Hence, absorption generally takes longer and peak effects occur later after an overdose than after a therapeutic one.
Ingested chemicals are predominantly absorbed from the small intestine rather than the stomach because the small intestine has a larger surface area. Hence, decreased gastric emptying or bowel activity caused by the presence of food, disease, or the effects of ingested agents (e.g., anticholinergics, opioids, sedative–hypnotics, salicylates) can also delay or prolong absorption. Food and coingestants may decrease absorption by binding to the chemical within the gut lumen or by competitively inhibiting its dissolution and translocation. Absorption may also be decreased if intestinal motility is excessive.
Distribution
During distribution, chemicals may become bound to and inactivated by endogenous nontarget molecules such as serum proteins. The final distribution of chemical is uneven and reflects its affinity for active and inactive binding sites and the locations of such sites. It is also influenced by biologic variables such as age, sex, weight, and disease states as they relate to body composition (e.g., water, fat, muscle content) and serum protein concentrations. The extent of distribution of a chemical is reflected by its apparent volume of distribution, measured in liters per kilogram of body weight, and calculated most simply by dividing the amount of chemical in the body (i.e., the absorbed or bioavailable dose) by its plasma concentration.

Because distribution is also a translocation process, it is influenced by the same chemical characteristics as absorption and follows first-order kinetics. Distribution generally occurs much faster than absorption, as evidenced by the occurrence of peak effects within minutes of an IV drug injection. Slow distribution is partly responsible for the delayed onset of action of some agents (e.g., digitalis, heavy metals, lithium, and salicylates).
Tissue Concentration
The severity of poisoning reflects the concentration of a chemical at its site(s) of action and is proportional to the dose. Because the blood concentration of a chemical is also proportional to the dose, blood levels are sometimes used as a surrogate to assess the severity of poisoning. However, blood and target site concentrations are not always in steady-state equilibrium. When distribution occurs more slowly than absorption (e.g., after IV administration, inhalational exposure, and the ingestion of agents with inherently slow distribution), blood levels may be higher than those in tissue. Conversely, when redistribution of a chemical from tissue to blood occurs more slowly than elimination (e.g., after extracorporeal removal), blood levels may be lower than those in tissue. In both instances, blood levels do not accurately reflect those in tissue and do not correlate with the severity of poisoning.
Age, genetic influences, tolerance, underlying disease, and the presence or absence of other chemicals may have synergistic or antagonistic effects and may also influence the response to a given level of toxin exposure. The effect of metabolites must also be considered. Many chemicals have metabolites that remain pharmacologically active. Some (e.g., acetaminophen, toxic alcohols, chlorinated hydrocarbons, meperidine, paraquat, and certain organophosphate insecticides) undergo metabolic activation, resulting in the production of compounds that are more toxic than the parent one.
Metabolism/Elimination
Elimination of chemicals from the body (detoxification) is accomplished by urinary, pulmonary, GI, and glandular (e.g., bile, milk, tears, saliva, sweat) excretion or metabolic inactivation. Hepatic metabolism and renal excretion are the major routes of elimination for most agents. Pulmonary excretion also plays a major role in the elimination of gases and volatile chemicals. Elimination generally follows first-order kinetics. For some toxins, hepatic metabolism has a finite capacity (i.e., becomes “saturated”) and proceeds at a constant rate (zero-order kinetics). When the primary route of elimination is a zero-order metabolism, a small increase in dose can result in a large increase in blood and tissue concentrations and potential poisoning. Chemicals exhibiting such metabolism include alcohols, phenytoin, salicylate, and theophylline.
Renal excretion is accomplished by translocation processes (e.g., glomerular filtration, tubular secretion, and reabsorption) and is therefore influenced by the same factors as absorption and distribution. Any condition that impairs hepatic or renal blood flow or function can decrease toxin elimination. Metabolic enzymes are also subject to genetic influences and to induction or inhibition resulting from past or current chemical exposures. Regardless of the kinetics and route of elimination, the time required for elimination increases as the tissue concentration of chemical increases. Hence, the duration of the effect tends to be longer after an overdose than after a therapeutic dose.
Clinical Considerations
The principal objectives in the diagnosis and evaluation of the poisoning are recognition of an exposure or poisoning, identification of the offending agent(s), prediction of potential toxicity, and assessment of the severity of clinical effects. Treatment objectives include resuscitation, prevention of further absorption, enhancement of elimination, and the administration of antidotal therapy (Table 117.2).
Early accurate diagnosis is a prerequisite for optimal management.
The priority of assessment and treatment objectives depends on the phase of poisoning [28]. During the preclinical phase (i.e., the time between exposure and the onset of clinical or laboratory evidence of toxicity), management priorities include chemical identification, prediction of toxicity, and prevention of absorption (i.e., decontamination). The sooner decontamination is accomplished, the greater its efficacy. Hence, the physical examination and gathering of ancillary data should initially be brief. Assessment should focus on the exposure history, whether or not poisoning is likely to ensue, and whether or not decontamination is indicated.
Table 117.2 Treatment Objectives—General Principles | |
---|---|
|
During the toxic phase (i.e., the time between the onset of toxicity and its peak), assessment of the severity of poisoning, resuscitation, prevention of further absorption, enhancement of elimination, and antidotal therapy are the primary objectives. If the patient is critically ill, the history, physical examination, and diagnostic testing must be conducted concurrently with resuscitation.
During the resolution phase (i.e., the time between peak toxicity and full recovery), continued supportive care, enhancement of elimination, antidotal therapy, and reassessment of severity (i.e., evaluation of the response to treatment) are the most important management considerations. Measures to prevent subsequent reexposure should also be initiated before discharge.
Recognition of Poisoning
Although poisoning can cause a wide variety of nonspecific signs and symptoms, the diagnosis can usually be established by the history, physical examination, routine and toxicologic laboratory evaluation, and the clinical course. Ideally, criteria similar to Koch’s postulates for infectious disease should be met: A chemical is identified in or on the body in an amount known to cause the observed signs and symptoms within the reported time frame. In reality, the diagnosis is often made on the basis of a history of exposure, a clinical course consistent with poisoning, and exclusion of other etiologies.
Making the diagnosis is easy when an accurate history of exposure is available. However, patients may be unaware of an exposure, unwilling to admit to one, or unable to give a history at all. Patients may give a history that is vague, confusing, or intentionally disguised.
Circumstances that should arouse suspicion of occult poisoning include sudden or unexplained illness in a previously healthy individual; similar unexplained symptoms in a group of individuals; a psychiatric history, alcoholism, or drug abuse; a recent change in health, economic status, or social relationships; and the onset of illness shortly after ingesting food, drink, or medication. Poisoning should always be considered in patients with metabolic abnormalities (especially acid–base disturbances), gastroenteritis, or changes in behavior or mental status of unclear etiology. Leakage of illicit drug packets that have been ingested or concealed in body cavities should be suspected in patients with altered mental status or unusual
behavior who have just arrived from abroad (especially Asia and South America) or who have recently been arrested or incarcerated for criminal activity [29,30]. Drug intoxication is a risk factor for trauma and suicide and should also be considered in all injured patients [31].
behavior who have just arrived from abroad (especially Asia and South America) or who have recently been arrested or incarcerated for criminal activity [29,30]. Drug intoxication is a risk factor for trauma and suicide and should also be considered in all injured patients [31].
To avoid missing the diagnosis of poisoning, the physician must specifically inquire about toxin exposure. In suspicious cases, the physician should assume the role of detective to elicit historical support for the diagnosis [32]. Paramedics, police, and family, friends, employer, pharmacist, or personal physician can be questioned regarding the circumstances and events surrounding the illness, particularly the availability of chemicals and the likelihood of exposure. The patient’s clothes and place of discovery should be searched for a suicide note, xenobiotics, and open or empty medication containers. Third parties should be instructed to search the house for such evidence and to bring it in for inspection.
In the absence of a history of exposure, the characteristic clinical course of poisoning may also suggest the diagnosis. Signs and symptoms of poisoning typically develop within minutes to an hour of an acute exposure, progress to a maximum within several hours, and gradually resolve over a period of hours to a few days. In such situations, toxicology screening (see later) may allow for a positive diagnosis if signs and symptoms are consistent with the known toxicity of the toxin(s) detected and other etiologies have been excluded.
Identification of the Offending Agent
History
The etiology of poisoning may or may not be disclosed by the patient history. Even when a history is available, its accuracy and reliability must be assessed. The identity of the toxin involved is incorrectly reported by up to 50% of patients with intentional ingestions [33]. The amount reportedly taken is also unreliable. Hence, in such patients, the history should be approached with caution. Layperson misidentification of acetaminophen as aspirin and vice versa is also relatively common. To avoid missing the correct diagnosis, the presence or absence of both drugs should be confirmed by laboratory analysis when an overdose of any kind is suspected.
Pill, Product, Plant, and Animal Identification
Drugs in pill form can often be identified by the imprint code, the alphabetical and numeric markings on tablets and capsules. A listing of imprint codes with the corresponding trade name and ingredient(s) can be found in the Identidex portion of Poisindex [27], which is available at virtually all poison centers in the United States. It also provides the identities of street drugs based on their slang names. Prescription drugs may be identified by contacting the dispensing pharmacy. Drug samples can sometimes be identified by direct chemical analysis (Toxicology Screening section). Police and government toxicology laboratories may be of assistance when illicit drug use is involved.
By US law [34], the ingredients of potentially hazardous commercial products used in and around the home must be stated on their label. This information, however, is not necessarily present or accurate, and labels may be missing or unreadable. In such cases, the ingredients may be identified by consulting Poisindex [27] or a regional poison center. Alternatively, the manufacturer or distributor can be called to obtain information on drugs or products that they produce or distribute. This action may be particularly helpful if the product is an outdated formulation or a recently reformulated or released one. Most large companies maintain 24-hour emergency telephone numbers for such purposes, and many employ medical consultants who can also provide management advice. Although industrial products do not have the same labeling requirements as household ones, right-to-know legislation requires that companies make information regarding the ingredients and potential toxicity of products they make, distribute, or use available to workers and health care providers [35]. Such information can be obtained by requesting a Material Data Safety Sheet (MSDS).
Information on drugs and chemical products manufactured or obtained outside the United States can be found in Poisindex [27] and Martindale: The Complete Drug Reference [21], or obtained from a domestic or foreign poison center. Information on drugs undergoing clinical trials in the United States may also be found in Martindale, since such drugs are often already available in other countries. Most foreign poison centers have English-speaking staff or translators available.
Plants (including fungi or mushrooms), along with their active parts and chemical constituents, can be identified by consulting Poisindex [27] if either their common or botanical name is known. If the name is not known but a sample is available, a representative from a local nursery, horticultural or mycologic society, or university botany department may be of assistance in identifying it. Similarly, pet stores, zoos, veterinarians, amateur or academic entomologists, herpetologists, zoologists, and field guides can be helpful in identifying potentially venomous insects, reptiles, snakes, and other animals. Poison centers usually maintain lists of local experts who are willing to help with such identifications.
Toxidromes
A toxidrome is a clinical syndrome that involves multiple physiologic systems and facilitates bedside identification of the culprit toxin [36]. The physiologic state of the patient can usually be characterized as excited (i.e., central nervous system [CNS] excitation with increased blood pressure, pulse, respirations, and temperature), depressed (i.e., decreased level of consciousness and decreased vital signs), discordant (i.e., inconsistent, mixed, or opposing CNS and vital sign abnormalities), or normal. The differential diagnosis can then be narrowed to the common or characteristic causes of these physiologic states (Table 117.3).
The excited state is primarily caused by sympathomimetics (agents that directly or indirectly stimulate α- and β-adrenergic receptors), anticholinergics (agents that block parasympathetic muscarinic receptors), hallucinogens, and withdrawal syndromes. The depressed state is primarily caused by sympatholytics (agents that block adrenergic receptors or depress cardiovascular activity), cholinergics (agents that directly or indirectly stimulate muscarinic receptors), opioids, or sedative hypnotics (which enhance the effect of the inhibitory CNS neurotransmitter gamma-aminobutyric acid [GABA] or depress neuronal membrane excitability). The discordant state is primarily due to asphyxiants (agents that decrease the availability, absorption, transport, or use of oxygen), membrane active agents (those that block sodium channels or otherwise alter the activity of excitable cell membranes), and agents that cause a variety of CNS syndromes due to interference with dopamine, GABA, glycine, or the synthesis, metabolism, or function of serotonin. A normal physiologic state may be due to a nontoxic exposure (Table 117.4), psychogenic illness, or presentation during the preclinical phase of poisoning. Agents that have a long preclinical phase (i.e., delayed onset of toxicity) are known as toxic “time bombs.” Delayed onset of toxicity may result from slow absorption or distribution, metabolic activation, or a mechanism of action
that involves the disruption of metabolic or synthetic pathways. Psychogenic illness should be considered when symptoms are inconsistent with the reported exposure and cannot be substantiated by objective physical findings, laboratory abnormalities, and toxicologic testing and other etiologies have been excluded [37].
that involves the disruption of metabolic or synthetic pathways. Psychogenic illness should be considered when symptoms are inconsistent with the reported exposure and cannot be substantiated by objective physical findings, laboratory abnormalities, and toxicologic testing and other etiologies have been excluded [37].
Table 117.3 Differential Diagnosis of Poisoning Based on Physiologic Assessment and Underlying Mechanisms | ||||||||||||
---|---|---|---|---|---|---|---|---|---|---|---|---|
|
Table 117.4 Criteria for a Nontoxic Exposure | |
---|---|
|
An excited or depressed state may be mischaracterized as a discordant one when the activity of a stimulant or depressant is selective for a receptor subtype or results in a compensatory or opposing autonomic response. For example, hypotension caused by an alpha-blocker, β2-agonist, or vasodilator may be
accompanied by tachycardia, and hypertension due to a selective α-agonist (e.g., phenylpropanolamine) may be accompanied by bradycardia. Severe stimulant or depressant poisoning can also cause what appears to be a discordant state (Table 117.5). For example, prolonged seizures and extreme hyperthermia caused by sympathomimetics can culminate in cardiovascular collapse as a consequence of anaerobic metabolism, acidosis, or depletion of neurotransmitters. Similarly, marked hypotension and hypoventilation caused by physiologic depressants can precipitate seizures and tachyarrhythmias as a result of ischemia, anoxia, and acidosis. In addition, paradoxic excitation can result from the preferential inhibition of cortical function that normally controls social activity by low doses of CNS depressants, most notably alcohol and other sedative hypnotics. In such cases, the physiologic state and its cause can often be correctly identified by the overall clinical picture and course of events.
accompanied by tachycardia, and hypertension due to a selective α-agonist (e.g., phenylpropanolamine) may be accompanied by bradycardia. Severe stimulant or depressant poisoning can also cause what appears to be a discordant state (Table 117.5). For example, prolonged seizures and extreme hyperthermia caused by sympathomimetics can culminate in cardiovascular collapse as a consequence of anaerobic metabolism, acidosis, or depletion of neurotransmitters. Similarly, marked hypotension and hypoventilation caused by physiologic depressants can precipitate seizures and tachyarrhythmias as a result of ischemia, anoxia, and acidosis. In addition, paradoxic excitation can result from the preferential inhibition of cortical function that normally controls social activity by low doses of CNS depressants, most notably alcohol and other sedative hypnotics. In such cases, the physiologic state and its cause can often be correctly identified by the overall clinical picture and course of events.
Table 117.5 Physiologic Grading of the Severity of Poisoning | ||||||||||||||||||
---|---|---|---|---|---|---|---|---|---|---|---|---|---|---|---|---|---|---|
|
The severity of mental status changes and the nature of associated autonomic findings can be used to narrow the differential diagnosis of physiological stimulation and depression to one of four subcategories (see Table 117.3). In the excited patient, marked vital sign abnormalities (e.g., severe hypertension with end-organ ischemia, tachyarrhythmias, hyperthermia, cardiovascular collapse) with minor mental status changes suggest an agent with peripheral sympathomimetic activity as the cause. Conversely, marked mental status abnormalities with nearly normal vital signs suggest a centrally acting hallucinogen. Anticholinergic poisoning (Table 117.6) can be differentiated from sympathomimetic (Table 117.7), hallucinogen, and withdrawal syndromes by the presence of dry, flushed, and hot skin; decreased or absent bowel sounds; and urinary retention. Other causes of excitation are usually accompanied by pallor, diaphoresis, and increased bowel or bladder activity.
In the patient with physiological depression, marked cardiovascular abnormalities (e.g., hypotension and bradycardia) with relatively clear sensorium suggest a peripherally acting sympatholytic, whereas marked CNS and respiratory depression with minimal pulse and blood pressure abnormalities suggest a centrally acting agent (opioid or sedative hypnotic). Cholinergic poisoning (Table 117.8) can be distinguished from other causes of physiologic depression by the presence of characteristic autonomic findings: Salivation, lacrimation, urination, defecation, GI cramps, and emesis (SLUDGE syndrome). In addition, cholinergic poisoning causes pallor and diaphoresis, whereas the skin is usually warm and dry with opioid and sedative–hypnotic poisoning.
Table 117.6 Anticholinergic Toxidrome | |
---|---|
|
Table 117.7 Sympathomimetic Toxidrome | |
---|---|
|
Other findings can sometimes help narrow the differential diagnosis further. Only the most common and diagnostically useful ones are noted here. Because of limited specificity and sensitivity, the presence or absence of a particular sign or symptom cannot be used to confirm or exclude a given etiology.
Ocular findings can sometimes help narrow the diagnostic possibilities. Although mydriasis can be caused by any agent or condition that results in physiologic excitation (see Table 117.3), it is most pronounced in anticholinergic poisoning, in which it is associated with minimal pupil response to light and accommodation. Similarly, although miosis is a nonspecific manifestation of physiologic depression, it is usually most pronounced in opioid poisoning. Notable miosis can, however, also be caused by cholinergic agents and sympatholytics with alpha-blocking effects (e.g., phenothiazines). Visual disturbances suggest anticholinergic, cholinergic, digitalis, hallucinogen, methanol, and quinine poisoning. Horizontal nystagmus and disconjugate gaze are nonspecific manifestations
of sedative–hypnotic poisoning. Although vertical and rotary nystagmus can be seen in patients with lithium and phenytoin poisoning, they are most suggestive of phencyclidine intoxication. These etiologies should be readily distinguishable by assessing the physiologic state. Rapidly alternating lateral “ping-pong” gaze has been described in monoamine oxidase inhibitor poisoning. Except for abnormalities due to topical chemical exposure, both eyes are equally affected. Although failure to respond to topical miotics has been said to be diagnostic of drug-induced pupillary dilatation, this is only true for topical exposures. Hence, unilateral pupillary abnormalities should generally prompt evaluation for a central, structural lesion.
of sedative–hypnotic poisoning. Although vertical and rotary nystagmus can be seen in patients with lithium and phenytoin poisoning, they are most suggestive of phencyclidine intoxication. These etiologies should be readily distinguishable by assessing the physiologic state. Rapidly alternating lateral “ping-pong” gaze has been described in monoamine oxidase inhibitor poisoning. Except for abnormalities due to topical chemical exposure, both eyes are equally affected. Although failure to respond to topical miotics has been said to be diagnostic of drug-induced pupillary dilatation, this is only true for topical exposures. Hence, unilateral pupillary abnormalities should generally prompt evaluation for a central, structural lesion.
Table 117.8 Cholinergic Toxidrome | |
---|---|
|
Dermatologic abnormalities may also be helpful. Flushed skin can be caused by anticholinergics, boric acid, a disulfiram-ethanol reaction, monosodium glutamate, niacin, scombroid (fish poisoning), and rapid infusion of vancomycin (red man syndrome). The skin is hot and dry in anticholinergic poisoning but normal or moist with other etiologies. Flushing should not be confused with the orange skin discoloration caused by rifampin. Pallor and diaphoresis may be due to cholinergics, hallucinogens, hypoglycemics, sympathomimetics, and drug withdrawal (see Table 117.3). As noted previously, manifestations of the SLUDGE syndrome distinguish cholinergic poisoning from other etiologies. Cyanosis may be due to agents that cause cardiovascular or respiratory depression, methemoglobinemia, pneumonitis, or simple asphyxia. In methemoglobinemia, it may have a chocolate-brown or slate-gray hue and is unaffected by oxygen administration. Cyanosis should not be confused with the blue discoloration of the skin caused by amiodarone or by topical exposure to blue dyes. The latter condition can be diagnosed by wiping the skin with acetone or alcohol. Hair loss, mucosal pigmentation, and nail abnormalities are suggestive of heavy metal poisoning (e.g., arsenic, lead, mercury, thallium).
Finally, the presence of neuromuscular abnormalities may suggest certain etiologies. Seizures and tremors can be caused by cholinergics, hypoglycemic agents, lithium, membrane-active agents, some narcotics (e.g., meperidine, propoxyphene), and most physiologic stimulants [38] (see Table 117.3). They can also occur in patients poisoned by agents that cause asphyxia, low lactate increased AGMA (see later), and cerebral hypoperfusion or hypoventilation (e.g., physiologic depressants; see Table 117.3). The most common causes of seizures due to poisoning are tricyclic antidepressants, sympathomimetics, antihistamines (primarily diphenhydramine), theophylline, and isoniazid. Although carbon monoxide, hypoglycemics, lithium, and theophylline can cause focal seizures, seizures due to poisoning are usually generalized. Because hypertensive and traumatic CNS hemorrhages are known complications of poisoning, the possibility of a structural lesion should be considered if focal signs and symptoms are present. Myoclonus suggests anticholinergic or sympathomimetic poisoning. Fasciculations are typical of cholinergic insecticide poisoning but can also be caused by sympathomimetics. Rigidity may be seen in phencyclidine and sympathomimetic poisoning and in those with CNS syndromes (see Table 117.3). Dystonic posturing is most often caused by antipsychotic agents. It is also a characteristic feature of strychnine poisoning.
Laboratory Findings
Acid–base status, anion gap, serum osmolality, ketone, electrolyte, glucose, and organ function abnormalities identified by routine laboratory tests can be extremely helpful in the differential diagnosis of poisoning. As with clinical manifestations, the diagnostic sensitivity and specificity of a single finding is not sufficiently high for its presence or absence to confirm or exclude a specific etiology. The use of anion and osmolar gaps and serum ketone and lactate levels in the diagnosis of poisoning of unknown etiology is summarized in Figure 117.1.
Assessing acid–base status and calculating the anion gap is particularly important because an increased AGMA may be due to advanced ethylene glycol, methanol, and salicylate poisoning. In such cases, prompt initiation of specific therapies is essential to prevent progressive, irreversible, or fatal poisoning [39,40]. The normal anion gap is 13 ± 4 mEq per L in unselected acutely hospitalized patients. In ethylene glycol and methanol poisoning, AGMA is primarily due to the accumulation of acid metabolites. In salicylate poisoning, it is caused by the accumulation of a variety of endogenous organic acids resulting from salicylate’s interference with intermediary metabolism. Agents that cause hypoxemia, cellular asphyxia, seizures, shock, or extensive tissue necrosis can also cause an AGMA, but in these instances, the accumulation of lactic acid generated by anaerobic metabolism is responsible for the AGMA. When the underlying cause is unclear, measuring the serum lactate level may be helpful. The lactate concentration is usually low (< 5 mEq per L) or significantly less than the anion gap in ethylene glycol, methanol, and salicylate poisoning, but high (> 5 mEq per L) or nearly equal to the anion gap in conditions associated with anaerobic metabolism.
Other common toxicologic causes of a low-lactate AGMA include ethanol, which can cause ketoacidosis by disrupting intermediary metabolism in susceptible alcoholics, and toluene, which can cause renal tubular acidosis with bicarbonate wasting. Rarely, this metabolic picture occurs in poisoning by formaldehyde (which is metabolized to formic acid), paraldehyde (presumably as a result of its metabolism to acetic acid), phosphate [41], and sulfur (and possibly sulfates) [42]. It can also be seen with large overdoses of ibuprofen (and probably all nonsteroidal anti-inflammatory agents) and valproic acid (due to high levels of these acidic drugs and their metabolites) [43,44]. Metformin and nucleoside reverse transcriptase inhibitor antiretroviral agents (e.g., zidovudine or azidothymidine) can interfere with normal-lactate metabolism and cause a high-lactate AGMA at therapeutic as well as excessive doses [45,46]. A high-lactate AGMA can rarely occur soon after massive acetaminophen ingestion [47].
An abnormally low anion gap may be seen in severe bromide, calcium, iodine, lithium, magnesium, and nitrate intoxication [39,48,49]. In bromide, iodine, and lithium intoxication, the low anion gap results from spuriously elevated chloride levels, and with nitrate poisoning, it is due to falsely elevated bicarbonate levels.
Serum osmolality can help differentiate the toxic causes of a low-lactate AGMA. An increased osmole gap may be seen early in the course of ethylene glycol and methanol (when high serum levels of the parent compounds are present) but not salicylate poisoning. Although not strictly accurate from a physical chemistry perspective [50], the osmole gap is typically defined
as the difference between the measured serum osmolality and the calculated serum osmolality.
as the difference between the measured serum osmolality and the calculated serum osmolality.

where normal serum osmolality is 290 ± 10 mOsm per kg of H2O normal osmole gap is 5 ± 7 mOsm per kg (in unselected acutely hospitalized patients [40]).

Normal osmole gap is 5 ± 7 mOsm per kg (in unselected hospitalized patients [40])
This formula assumes that all concentrations are measured in millimoles per liter. If the glucose and BUN concentrations are measured in milligrams per deciliter, dividing them by 18 and 3, respectively, gives their approximate concentrations in millimoles.
Additional causes of an increased osmolar gap include other low-molecular-weight solutes, such as acetone, ethanol, isopropyl alcohol, magnesium, mannitol, and propylene glycol [51]. The approximate concentration of these substances that will increase the serum osmolality by 1 mOsm per kg of H2O, calculated on the basis of their molecular weights, is shown in Table 117.9. When direct measurements are not readily available, the serum concentration of these agents can be estimated by multiplying this amount by the osmolar gap. Serum osmolality must be measured by freezing point depression rather than the headspace or vapor pressure method to detect the presence of volatile agents such as acetone and toxic alcohols. An increased osmolar gap has also been reported in alcoholic ketoacidosis and conditions causing lactic acidosis [52].
Serum ketones can also help to differentiate the toxic causes of a low-lactate AGMA. Ketosis, as defined by a positive nitroprusside reaction, is relatively common in salicylate poisoning but unusual in ethylene glycol and methanol poisoning. Ketosis is also seen in alcoholic ketoacidosis and in acetone and isopropyl alcohol poisoning.
Table 117.9 Effects of Some Solutes on Serum Osmolality | ||||||||||||||||||||||||||||
---|---|---|---|---|---|---|---|---|---|---|---|---|---|---|---|---|---|---|---|---|---|---|---|---|---|---|---|---|
|
The urinalysis, serum calcium concentration, and the overall clinical picture can also be helpful in differentiating the toxic causes of a low-lactate AGMA. Crystalluria, hypocalcemia, and back pain or flank tenderness suggest ethylene glycol; visual symptoms implicate methanol; and tinnitus or impaired
hearing point to salicylates. Crystalluria can also be caused by acyclovir [53], felbamate [54], indinavir [55], oxalate [56], primidone [57], and sulfa drugs [58]. Hypocalcemia is also seen in fluoride and oxalate [56] intoxication.
hearing point to salicylates. Crystalluria can also be caused by acyclovir [53], felbamate [54], indinavir [55], oxalate [56], primidone [57], and sulfa drugs [58]. Hypocalcemia is also seen in fluoride and oxalate [56] intoxication.
Serum potassium and glucose abnormalities may also provide clues to the etiology of poisoning [18,59,60]. Toxicologic causes of hypokalemia include barium, β2-adrenergic agonists, calcium channel blockers, chloroquine, diuretics, insulin, licorice, methylxanthines, and toluene. Hyperkalemia can be caused by α-adrenergic agonists, angiotensin-converting enzyme inhibitors, beta-blockers, digitalis, fluoride, potassium-sparing diuretics, and trimethoprim. Common toxicologic causes of hypoglycemia are ethanol, beta-blockers, hypoglycemics, quinine, and salicylate. Common causes of hyperglycemia include acetone, β-agonists, calcium channel blockers, iron, and methylxanthines.
Common toxicologic causes of acute liver dysfunction are acetaminophen, ethanol, halogenated hydrocarbons (e.g., carbon tetrachloride), heavy metals, and mushrooms (e.g., Amanita phalloides and related species) [61]. Acute renal toxicity is most often due to ethylene glycol, halogenated hydrocarbons, heavy metals, nonsteroidal anti-inflammatory drugs, toluene, envenomations, and agents that cause hemolysis or rhabdomyolysis [62]. An elevated creatinine with a normal BUN can be seen in acetone and isopropyl alcohol poisoning because acetone interferes with colorimetric assays for creatinine, resulting in falsely high results. Acute hemolysis (in the absence of glucose-6-phosphate dehydrogenase deficiency) can result from poisoning by arsine gas, naphthalene, and inducers of methemoglobinemia. Rhabdomyolysis is associated with toluene abuse, CNS syndromes (see Table 117.3), and severe physiologic dysfunction (e.g., extreme agitation, deep or prolonged coma, hyperthermia, seizures) of any etiology [63]. The most common agents involved are sympathomimetics, ethanol, heroin, and phencyclidine.
Electrocardiographic Findings
The ECG may provide clues to the cause of poisoning [18]. Ventricular tachyarrhythmias that occur in patients with normal QRS and QT intervals suggest myocardial irritation (i.e., increased automaticity) as the underlying mechanism. Sympathomimetics, digitalis, and cardiac-sensitizing agents such as chloral hydrate and aliphatic or halogenated hydrocarbons, which potentiate the action of endogenous catecholamines, are common causes [64]. In contrast, ventricular tachyarrhythmias that occur in the setting of depolarization and repolarization abnormalities, reflected by QRS and QT interval prolongation, respectively, suggest a reentrant mechanism. Causes include electrolyte abnormalities, organophosphate insecticides, and other membrane active agents (see Table 117.1) [65,66]. Torsades de pointes (polymorphous) ventricular tachycardia strongly implicates an agent that prolongs the QT interval.
Atrioventricular conduction abnormalities (atrioventricular block) and bradyarrhythmias can be caused by beta-blockers, calcium channel blockers, digitalis, membrane-active psychotherapeutic agents, organophosphate insecticides, and α-agonists such as phenylpropanolamine. With α-agonists, they are a reflex (i.e., homeostatic) response to hypertension, but with other causes, they are associated with generalized cardiovascular depression and hypotension.
Radiologic Findings
Ingested chemicals can sometimes be visualized within the GI tract by abdominal radiographic imaging, and such imaging can occasionally be helpful in suggesting the etiology or amount of an unknown ingestion. Although a large variety of chemicals can be detected by routine radiography in vitro, relatively few are visible in vivo [67]. Agents most likely to be visible on plain films are indicated by the mnemonic CHIPES (Table 117.10) [18].
Table 117.10 Xenobiotics Visible on Plain Stomach Radiographs | |
---|---|
|
Ingested drug packets may appear as uniform, ovoid or round, marble-sized densities scattered along the GI tract [29,30]. Ingested hydrocarbons may sometimes appear as a double gastric fluid level or “double bubble” because of the air–fluid and fluid–fluid interface lines created when less dense hydrocarbons layer on top of gastric fluids. Computed tomography may be superior to plain films in detecting ingested drug packets but the optimal test in this setting remains unclear [68,69]. Whether contrast should be used or not remains controversial. Abdominal ultrasound can detect ingested pills, particularly enteric-coated and sustained-released formulations [70]. Such imaging may be useful in confirming or refuting some recent specific (CHIPES) ingestions. Because the volume of pills can be determined, plain radiography may be used to guide GI decontamination.
Abnormal findings on chest radiography can be caused by a wide variety of chemicals [18,71]. Diffuse or patchy infiltrates (i.e., pneumonitis or acute lung injury) can be due to the inhalation of irritant gases (e.g., ammonia, chlorine, hydrogen sulfide, nitrogen oxides, phosgene, smoke, sulfur dioxide), fumes (e.g., beryllium, metal oxides, polymers), and vapors (e.g., acids, aldehydes, hydrocarbons, isocyanates, mercury). They can also be seen in patients who have ingested or injected cholinergic agents (e.g., carbamate and organophosphate insecticides), metabolic poisons (e.g., cyanide, carbon monoxide, heavy metals, hydrogen sulfide), paraquat, phencyclidine, salicylates, thiazide diuretics, and tocolytics and in patients with envenomations. Aspiration pneumonitis is quite common and can occur in patients with coma or seizures of any etiology [72]. Acute lung injury can also develop in any patient with prolonged or pronounced anoxia, hyperthermia, or hypotension (e.g., those with severe opioids or sedative–hypnotic or sympathomimetic poisoning). Chronic chemical exposure can cause pulmonary fibrosis, granulomas, or pleural plaques.
Response to Antidotes
The use of antidotes for diagnostic purposes has largely fallen from favor. The availability of point of care blood glucose measurement negates the need for empiric intravenous dextrose in altered patients. Many antidotes may be harmful if used inappropriately, including flumazenil physostigmine, glucagon, nitrites, and chelators. Naloxone remains a reasonably safe therapy in a patient with clinical signs of opiate intoxication. Clinicians should be prepared to manage acute withdrawal and its sequelae.
Toxicology Screening
Analysis of a sample of the toxin itself, or patient urine, blood, gastric contents, or hair [71,73] can sometimes be helpful in identifying the cause of poisoning. Urine is generally the best specimen to analyze because large quantities can be obtained
for extraction procedures and many chemicals are normally concentrated in urine. However, toxicology testing can detect only a small fraction of all chemicals (primarily drugs) and is not always reliable [74]. Immunoassay screens are inexpensive and provide results within minutes, but they are only capable of detecting a few agents. They suffer from many false positives and false negatives. Patients may be misdiagnosed and potentially harmed by clinicians acting solely on the results of immunoassay screens [75]. Comprehensive screens are expensive and require 2 to 6 hours for completion (excluding transportation times). Although results may increase diagnostic certainty or specificity, they rarely change disposition or treatment in patients who are asymptomatic or who have signs and symptoms consistent with the reported exposure [75,76,77,78,79,80]. Noteworthy exceptions are acetaminophen and salicylate, which are widely available, commonly ingested, sometimes misidentified, require specific treatment, and cause few or nonspecific early signs and symptoms. Hence, in most overdose patients, quantitative acetaminophen and salicylate levels are the only toxicology tests likely to be clinically useful.
for extraction procedures and many chemicals are normally concentrated in urine. However, toxicology testing can detect only a small fraction of all chemicals (primarily drugs) and is not always reliable [74]. Immunoassay screens are inexpensive and provide results within minutes, but they are only capable of detecting a few agents. They suffer from many false positives and false negatives. Patients may be misdiagnosed and potentially harmed by clinicians acting solely on the results of immunoassay screens [75]. Comprehensive screens are expensive and require 2 to 6 hours for completion (excluding transportation times). Although results may increase diagnostic certainty or specificity, they rarely change disposition or treatment in patients who are asymptomatic or who have signs and symptoms consistent with the reported exposure [75,76,77,78,79,80]. Noteworthy exceptions are acetaminophen and salicylate, which are widely available, commonly ingested, sometimes misidentified, require specific treatment, and cause few or nonspecific early signs and symptoms. Hence, in most overdose patients, quantitative acetaminophen and salicylate levels are the only toxicology tests likely to be clinically useful.
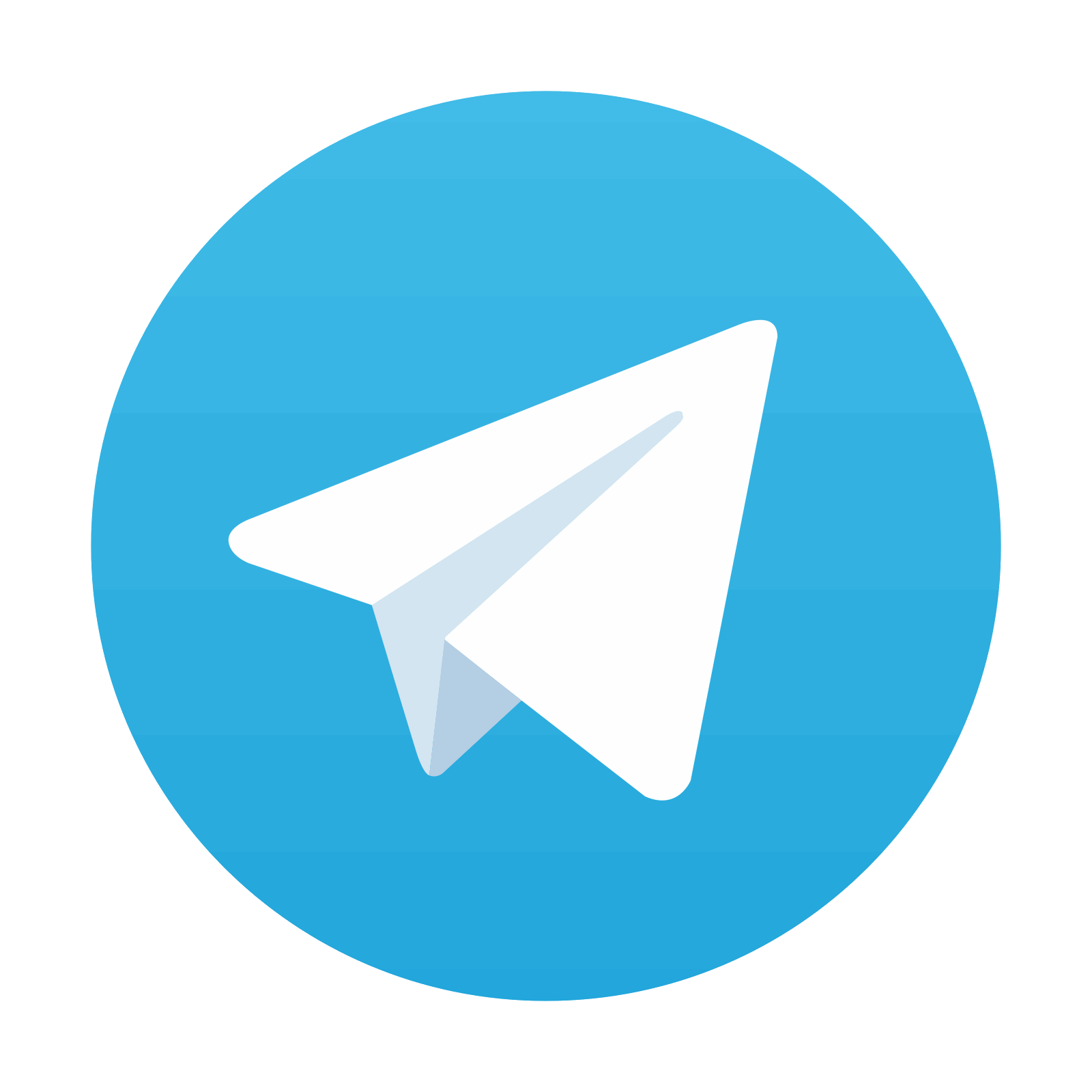
Stay updated, free articles. Join our Telegram channel

Full access? Get Clinical Tree
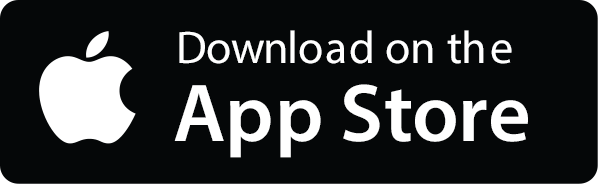
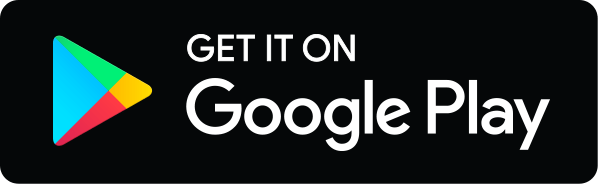
