Introduction
In previous decades, pain research has been relatively stagnant and with limited funding. In 2011, only 1% of the $30 billion National Institutes of Health (NIH) budget was devoted to pain research. An integral piece in the framework of pain research was added in 2009 with the Congressional Mandate and National Pain Care Policy Act, which set out plans for the Secretary of Health and Human Services to meet with the National Academy of Medicine (previously known as Institute of Medicine) for a conference on pain.
In 2011, the NIH and National Academy of Medicine (NAM) published Relieving Pain in America: A Blueprint for Transforming Prevention, Care, Education, and Research . This effort helped guide the development of the National Pain Strategy: A Comprehensive Population Health-Level Strategy for Pain, released by the US Department of Health and Human Services (HHS) and the Interagency Pain Research Coordinating Committee (IPRCC) 5 years later. The National Pain Strategy promoted providing individualized, patient-centered, interdisciplinary care to people experiencing pain. In addition, the Federal Pain Research Strategy was developed to expand upon the goals of the National Pain Strategy.
The Federal Pain Research Strategy was released in 2017 and helped to identify and prioritize a long-term strategic plan for the federal pain research agenda. In 2018, the NIH established the initiative Helping to End Addiction Long-Term (HEAL), which included the efforts of multiple agencies to promote and fund research for managing pain in the context of the national opioid public health crisis.
Pain continues to have a substantial impact on patients, their environment, and the healthcare system. Globally, the estimated prevalence of chronic pain in adults is 20%. , In the United States, an estimated 50 million (20.4%) adults have chronic pain, with 19.6 million (8.0%) of adults having high-impact chronic pain that interferes with work or life most days or every day.
Total annual costs for chronic pain in the United States are estimated at $560–$635 billion. This figure is greater than six of the most costly major diagnoses: cardiovascular diseases ($309 billion); neoplasms ($243 billion); injury and poisoning ($205 billion); endocrine, nutritional, and metabolic diseases ($127 billion); digestive system diseases ($112 billion); and respiratory system diseases ($112 billion). , Interestingly, the per-person cost of pain is less than the other conditions, but because pain affects a larger number of individuals the total costs are higher.
To address the extensive ongoing burden of pain, it is imperative to thoughtfully consider all management options. This chapter focuses on future directions in pain research but does not claim to be an exhaustive list, as the goal of ongoing research is to develop new contributions to the field and continually improve the management available to those suffering from pain.
Broad Research Proposals
The International Association for the Study of Pain defines pain as “an unpleasant sensory and emotional experience associated with actual or potential tissue damage, or described in terms of such damage.” Prompted by this definition, future research will tackle the treatment of pain by attempting to better understand the sensory inputs, the emotional inputs, as well as the whole of the person’s experience. A person’s experience is personal and subjective, and therefore, future research will look into individualized treatment options.
The goal will be to treat acute or chronic pain with a plan that addresses the numerous issues that contribute to pain and affect the patient’s functioning. Research will attempt to address novel nociceptive and neuropathic signaling mechanisms at the point where the pain originates, as well as transduction pathways along the peripheral and central nervous system and cortical areas responsible for perceiving and responding to the signals that create an individual experience of pain. , , It will be important to understand the transition from acute to chronic pain, as well as develop interventions that prevent chronic pain from developing.
There will be a need to increase our knowledge of peripheral and central signaling mechanisms at the molecular level, with a better understanding of receptors, downstream activators, and transcription factors, as well as pathways involved in the pathophysiology of pain. This will also include a better understanding of individualized pain responses based on gender, genetics, epigenetic changes, as well as pharmacogenomic interactions. Improved animal models and high-throughput screening technologies will be needed to quickly screen small molecules for improved pharmacotherapy.
In addition, improved assays will be developed to elucidate drug interactions and drug metabolism based on an individual’s genetic makeup. Furthermore, functional MRIs will be used to evaluate pain in real time, to better understand how brain regions change with pain, which brain regions impact pain processing, and which emotional and cognitive factors can influence pain and alter pain perception and, therefore, the pain experience.
Research will also be aimed at attempting to identify objective measures of pain. This includes biomarkers that may be present in the brain, blood, or tissues, so that researchers can objectively evaluate pain treatment. In addition, research will focus on the identification of pain phenotypes, including descriptions of pain, such as shooting, stabbing, pricking, or burning, and use of specific treatments for different pain phenotypes as a means of attempting to correlate these phenotypes with genotypes.
Multidisciplinary Treatments
Pain is a physical, social, psychological, and spiritual experience. Numerous individualized treatments have been used to address the various aspects of the pain experience, including but not limited to the following: education, exercise (strength training and stretching), acupuncture, physical therapy, occupational therapy, aquatherapy, massage, chiropractic therapy, osteopathic therapy, craniosacral therapy, relaxation training, guided imagery, homeopathy, energy medicine, hypnosis, meditation/mindfulness, sleep hygiene, breathing techniques, yoga, Pilates, tai chi, cognitive behavioral therapy, acceptance and commitment therapy, biofeedback, nutraceuticals, and medications.
The pain experience is complex, and the etiology is multifactorial. With multiple factors and pathways contributing to the pain signal and modulating the experience, multiple targets are needed to treat the pain. This would therefore not only include the possibility of multiple pharmaceuticals but also multiple treatment modalities. It is generally accepted that pain management and pain treatment will be a team-based care model that is integrated, interdisciplinary, coordinated, and patient-centered. It will address the multiple dimensions of pain from a physical, social, psychological, and spiritual perspective. However, there is still a lack of data for long-term sustainability of effects and overlapping pain conditions and psychosocial factors, as well as for which modalities should be combined in an interdisciplinary model, and at which intervals or doses. ,
Research will be performed to determine which modalities should be combined, at what time in the healing process, and at what dose and frequency. , Given the multifactorial nature of pain, it is unlikely that the same treatment modality, or combination of modalities, could be used for everyone. Interdisciplinary treatment modalities will need to be individualized, as treatment is not a one-size-fits-all paradigm; therefore, research will need to be performed to help identify which patients will respond to which particular combination of modalities and interventions. This has the potential of dramatically improving the treatment of pain.
In addition, oftentimes, nonpharmacological treatment modalities are not available to patients, either due to access issues or insurance coverage. Future research will help expand healthcare and increase access to treatment options through technologies such as telehealth and digital platforms. This may include educational or instructional videos, podcasts, and live telehealth appointments with health professionals in individual or group settings. More research will be performed using treatment apps on smartphones and web portals on computers and by monitoring progress with direct patient-to-provider communication and alerts. This may help improve patient compliance, increase monitoring that may decrease emergency room or hospital visits, and help advance interdisciplinary treatment models that can be provided long distance through technology.
Novel Pharmaceuticals
The topic of pharmaceuticals is broad and includes medications that have previously been used, are currently being used, or are being considered for future use in treating pain. Here we concentrate on pharmaceuticals and pharmacological targets in the treatment of pain that are in various stages of discovery and development. The discovery, development, and implementation of potential pharmaceutical treatments involve many factors, including the significant roles of the various pharmaceutical regulatory agencies and organizations worldwide; for example, the regulations of the US Food and Drug Administration. While some of the pharmaceuticals and their targets listed below are currently used in practice and are being evaluated for improvements, others are in more experimental stages of development. Clinical trials for the novel pharmaceuticals discussed below and currently registered on https://ClinicalTrials.gov are highlighted in Table 18.1 .
Drug Name | ClinicalTrials.gov Identifier | Mechanism/Target | Condition or Disease | Phase | Recruitment Status |
---|---|---|---|---|---|
PF-04457845 | NCT00981357 | Fatty acid amino hydrolase inhibitor | Pain, knee osteoarthritis | 2 | Completed |
Lacosamide | NCT00220337 | Voltage-gated sodium channels antagonist | Painful diabetic neuropathy | 3 | Completed |
Lacosamide | NCT00546351 | Voltage-gated sodium channels antagonist | Painful distal diabetic neuropathy | 3 | Completed |
Eslicarbazepine acetate (ESL) | NCT00980746 | Voltage-gated sodium channels antagonist | Painful diabetic neuropathy | 2 | Completed |
Eslicarbazepine acetate (ESL) | NCT01129960 | Voltage-gated sodium channels antagonist | Painful diabetic neuropathy | 3 | Terminated |
Rufinamide | NCT02095899 | Voltage-gated sodium channels antagonist | Postthoracotomy pain syndrome | 2 | Terminated |
Tetrodotoxin (TTX) | NCT00725114 | Voltage-gated sodium channels antagonist | Moderate to severe inadequately controlled cancer-related pain | 3 | Completed |
Pregabalin | NCT01529190 | Voltage-gated calcium channels antagonist | Pain intensity and interleukin-6 levels in living donor kidney | 2 | Completed |
Ethosuximide | NCT02100046 | Voltage-gated calcium channels antagonist | Peripheral neuropathic pain | 2 | Completed |
Ziconotide | NCT00002160 | Voltage-gated calcium channels antagonist | Chronic pain in cancer and AIDS | 2 | Completed |
Ziconotide | NCT03942848 | Voltage-gated calcium channels antagonist | Severe refractory neuropathic pain | 3 | Not yet recruiting |
Flupirtine | NCT01450865 | Voltage-gated potassium channel agonist | Pain, neuronal change | 1 | Completed |
SB705498 | NCT00461682 | TRPV1 receptor antagonist | Rectal pain | 2 | Terminated |
SB705498 | NCT00281684 | TRPV1 receptor antagonist | Dental pain | 2 | Completed |
Ketamine | NCT02729805 | TRPV1 and TRPA1 receptor gene expression | Chronic pain, mastectomy | 4 | Active, not recruiting |
Resiniferatoxin | NCT03226574 | TRPV1 receptor agonist | Intractable cancer pain | 1 | Recruiting |
Resiniferatoxin | NCT03542838 | TRPV1 receptor agonist | Pain, knee osteoarthritis | 1 | Recruiting |
Resiniferatoxin | NCT04044742 | TRPV1 receptor agonist | Pain, knee osteoarthritis | 3 | Recruiting |
GZ389988 | NCT02424942 | Tropomyosin-related kinase a (TrkA) inhibitor | Pain, knee osteoarthritis | 1 | Completed |
Oxytocin | NCT02100956 | Oxytocin receptor | Neuropathic pain | 2 | Recruiting |
Oxytocin | NCT03060031 | Oxytocin receptor | Pain, social influence effects on pain | 1 | Completed |
Oxytocin | NCT03011307 | Oxytocin receptor | Postoperative pain (hip surgery) | 2 | Recruiting |
Fatty Acid Amino Hydrolase
Fatty acid amino hydrolase (FAAH) is an intracellular membrane-bound enzyme that hydrolyzes the endocannabinoid N-arachidonylethanolamide (AEA, or anandamide) and other fatty acid amides. AEA acts as an agonist at cannabinoid receptors, and by inhibiting FAAH, its physiologic effects can be prolonged. Some researchers suggest that it is possible to both utilize the cannabinoid system in the treatment of pain and limit the undesirable side effects reported with administration of exogenous cannabinoids by targeting the endocannabinoid system.
One example of the effect of the endocannabinoid system and AEA can be seen by looking at a microdeletion in a novel FAAH pseudogene (FAAH-OUT), identified in a patient with high AEA concentrations and pain insensitivity. Both pharmacologic and genetic targeting of FAAH have been studied in relation to multiple medical conditions, including pain.
Pharmacologic studies have yielded a wide range of results. A prior Phase I trial had unfortunate outcomes, when an acute neurologic disorder developed in healthy volunteers. Subsequent use of FAAH inhibitors for treating pain and other medical disorders has shown more promise, however. Another Phase I trial evaluating an FAAH inhibitor and peripheral neuropathic pain demonstrated a more favorable safety profile but no significant difference in primary endpoint versus placebo; however, it did so within subgroup analysis. More promise has been shown in Phase II trials evaluating FAAH inhibitors with cannabis use disorder and as a possible treatment for posttraumatic stress disorder. ,
In addition to further FAAH inhibitor trials, gene-editing techniques are also being considered. One such study explored how utilizing CRISPR interference (CRISPRi) to enable gene repression at the FAAH-OUT promoter reduces FAAH-OUT and FAAH expression. This highlights how CRISPRi and gene-editing techniques targeting FAAH-OUT may be developed as novel therapies in the treatment of pain.
Voltage-Gated Sodium Channels
Voltage-gated sodium channels (VGSCs) have nine identified subtypes (Na v 1.1–Na v 1.9), each with unique properties and tissue distributions. They play an integral role in membrane excitability and the propagation of action potentials. Although many VGSCs may have a role in pain, three are most strongly associated: Na v 1.7, Na v 1.8, and Na v 1.9. In particular, Na v 1.7 has demonstrated properties that make it a target of interest in the treatment of pain. Na v 1.7 channel gain of function mutations are linked to severe pain, and loss of function mutations are linked to pain insensitivity. , In three related families demonstrating a congenital inability to perceive pain but intact ability to perceive other sensory modalities, a mutation was found in gene SCN9A, the gene encoding the alpha subunit of Na v 1.7.
Clinical trials thus far exploring novel Na v 1.7 channel inhibitors have shown good safety but inconsistent clinical efficacy. Although results were less promising in subjects with pain due to trigeminal neuralgia and diabetic neuropathy, lacosamide, with a mechanism involving Na v 1.3, Na v 1.7, and Na v 1.8, had a significant effect on pain, general well-being, and sleep quality in subjects with Na v 1.7-related small-fiber neuropathy. In addition, tetrodotoxin, a neurotoxin produced by the pufferfish that primarily blocks Na v 1.7, has shown a favorable risk-benefit profile in the treatment of uncontrolled moderate to severe cancer-related pain. There have been numerous clinical trials with ongoing studies underway.
Voltage-Gated Calcium Channels
Voltage-gated calcium channels (VGCCs) play an important role in regulating signal transduction and initiating multiple physiologic events. The two major classes of VGCC include high voltage-activated (L, P/Q, N, R types) and low voltage-activated (T type). L, N, and T type VGCCs have been studied regarding their influence on pain.
L type VGCCs (Ca v 1) are primarily located postsynaptically and regulate neuronal firing and gene expression in a number of physiologic processes, including pain. With application to the dorsal horn, they were found to alter short-term pain sensitization, inflammation-induced hyperexcitability, and neuropathy-induced allodynia.
N type VGCCs (Ca v 2.2), located presynaptically, play a large role in neurotransmitter release. They are concentrated in dorsal root ganglia and the dorsal horn and have currently accepted medical use in the treatment of pain. Ziconotide (SNX-111) is a synthetic form of the peptide ω-conotoxin MVIIA (ω-MVIIA), which is found in Conus magus snail venom. Ziconotide has limited permeability across the blood-brain barrier and must be administered intrathecally. Potential limitations include side effect profile and mode of administration.
Various studies have been conducted in an effort to utilize the N type VGCC with an orally administered medication. Phase II trials evaluating a novel oral VGCC inhibitor with lumbosacral radiculopathy and postherpetic neuralgia did not meet their primary endpoint and were discontinued. Adjustments to the structure of these agents and their mode of action are currently being evaluated with electrophysiology experiments.
T type VGCCs (Ca v 3), located presynaptically, have a role in excitatory synaptic transmission and have multiple locations, including reticular thalamic neurons, cortical pyramidal neurons, the dorsal root ganglia, and the dorsal horn of the spinal cord. , Various subtypes have been implicated in multiple neurologic disorders, ranging from epilepsy to various chronic pain states, with a notably large impact on the initiation and maintenance of neuropathic pain.
A few examples of currently utilized pharmaceutical agents that target T type VGCC and their indications include ethosuximide (epilepsy), valproic acid (epilepsy, bipolar disorder), zonisamide (epilepsy), and nimodipine (hypertension). A Phase II trial evaluated the safety profile and analgesic efficacy of a novel selective T type Ca v 3.2 VGCC blocker in subjects with neuropathic pain. The novel agent was compared with pregabalin and a placebo and was found to lack analgesic efficacy, with mild to moderate side effects reported.
Another study compared ethosuximide with an inactive control in subjects with nondiabetic peripheral neuropathic pain but was stopped due to adverse events reported in the treatment group. A double-blind, placebo-controlled, randomized study of a novel orally available triple T-type Ca 2+ channel blocker (blocking Ca v 3.1, Ca v 3.2, Ca v 3.3) was found to have good tolerability after a single administration in healthy male subjects. Its tolerability, along with pharmacokinetic and pharmacodynamic properties, warrants further studies of multiple doses.
Potassium Channels
Potassium channels play a role in repolarization/hyperpolarization of the neuronal membrane, which can limit action potential propagation and firing rate. Potassium channels are described as the most widely distributed and diverse group of ion channels in neurons and are organized into four subgroups: voltage-gated (VGKC, or K v ), two-pore (K 2P ), calcium-activated (K Ca ), and inward-rectifying (K ir ). One illustration of the potential impact of potassium channels may be found in Isaacs Syndrome (acquired neuromyotonia), an antibody-mediated potassium channel disorder (channelopathy). Autoantibodies target VGKC and decrease the voltage-gated outward potassium current, causing peripheral motor nerve hyperexcitability.
VGKC complex autoantibodies have more recently been identified as being involved in the hyperexcitability of nociceptive pathways and development of chronic pain. As a decrease in potassium channel expression/current density can contribute to the development of chronic pain, therapeutically targeting the opening of potassium channels may promote analgesia. The results of an animal study showed that the drug riluzole prevented the chronic neuropathy that is often associated with the use of the chemotherapy agent oxaliplatin. The two-pore potassium channel TREK-1 was identified as playing a central role in the riluzole-induced antineuropathic effect.
Transient Receptor Potential Channels
Transient receptor potential channels (TRP channels) are a group of cation channels that play a role in the response to various external stimuli, including light, sound, chemical, temperature, and touch. There are seven subfamilies of TRP channels in total, some of which play a large role in nociception. The two most often implicated in pain are the transient receptor potential ankyrin 1 (TRPA1) and transient receptor potential vanilloid 1 (TRPV1) channels. TRPA1 and TRPV1 are coexpressed in a number of nociceptive sensory nerves, and their function is at times interlinked. ,
TRPA1 is the only member of the TRPA subfamily. The classic TRPA1 agonist was identified as mustard oil (active component allyl isothiocyanate), which is also present in wasabi and horseradish. There have been multiple agonists identified over time, with TRPA1 being identified as a potential therapeutic target in the treatment of pain.
The wide range of toxins that target TRPA1 have unique mechanisms to be considered. Crotalphine is the venom isolated from the South American rattlesnake Crotalus durissus terrificus that has been shown to provide significant analgesia in inflammatory, neuropathic, and cancer pain models. An animal study found that picomolar concentrations of administered crotalphine resulted in analgesia. By selectively acting as partial agonists at TRPA1 channels, they led to prolonged desensitization of the channel, causing the analgesic effect. An alternate mechanism was utilized while incorporating the wasabi receptor toxin (WaTx) isolated from the Black Rock scorpion. WaTx binding was found to stabilize the TRPA1 in an open state and diminish calcium permeability. This did produce pain and pain hypersensitivity but did not cause neurogenic inflammation. This suggests that WaTx as a potential treatment in limiting the neurogenic inflammation component to TRPA1-mediated pain, while preserving its acute protective role in chemical nociception.
TRPV1 is one of the six members in the vanilloid TRP subfamily. TRPV1 is the only member that is actually activated by vanilloids, with its classic agonist identified as capsaicin, the active component in chili peppers. It was then established that heat greater than 43°C and pH less than 5.9 also activates the channel.
Over time, many exogenous and endogenous agonists, as well as endogenous modulators, have been identified. One exogenous agonist that has been investigated extensively is resiniferatoxin (RTX). RTX is derived from the Euphorbia resinifera plant and has been reported to be the most potent among all synthetic and endogenous TRPV1 agonists. RTX acts by producing prolonged channel opening and calcium influx, causing cytotoxicity to the TRPV1-positive pain fibers or cell bodies. In addition, its mechanism has been referred to as “molecular neurosurgery,” or selective neuroablation, as it spares motor, proprioceptive, and other somatosensory function.
An animal study evaluating pain in the postsurgical period found that a targeted injection of RTX reduced evoked and nonevoked pain-related behaviors in subjects over the duration of postsurgical recovery. Animal studies with intrathecal administration of RTX on subjects with cancer pain showed promising results and have led to Phase I clinical trials in humans. In Phase I clinical trials, changes in pain assessed with the numeric rating scale (NRS) were not statistically significant at the doses of RTX tested. There are ongoing studies evaluating the safety and efficacy of higher administered doses of intrathecal RTX in subjects with severe refractory pain due to advanced cancer.
Tropomyosin-Related Kinases
Tropomyosin-related kinases (Trk), a family of receptor tyrosine kinases, have three isomers—TrkA, TrkB, and TrkC—that are expressed in neurons. These isomers are each activated by complementary neurotrophins: nerve growth factor (NGF) binds to TrkA; brain-derived neurotrophic factor (BDNF) binds to TrkB; and neurotrophin-3 (NT-3) binds to TrkC. All three Trk receptors have been implicated in neuropathic pain, while also being evaluated for their role in other types of pain.
TrkA receptors have been targeted as a potential therapeutic target for the treatment of pain. The neurotrophin NGF has roles in early neural development and in adults and contributes to neural plasticity, hypersensitization to noxious stimuli, and the signaling of pain. Increased levels of NGF have been associated with acute and chronic pain states.
The humanized anti-NGF monoclonal antibody tanezumab has been studied in a variety of clinical scenarios. Two randomized, double-blind, placebo-controlled, Phase I studies were conducted to evaluate safety, tolerability, and analgesic efficacy of tanezumab. Statistically significant improvements in tanezumab-associated analgesia in doses greater than or equal to micrograms/kg were seen in a study evaluating knee pain secondary to osteoarthritis; however, no statistically significant improvements were noted in the study evaluating patients undergoing bunionectomy.
A notable Phase III clinical trial included subjects with hip or knee osteoarthritis randomly placed in groups, with one taking 2.5 mg tanezumab every 8 weeks, another taking 5 mg tanezumab every 8 weeks, and a third taking daily NSAIDs. Results suggested that tanezumab has some analgesic efficacy for hip or knee osteoarthritis but is associated with increased rates of rapidly progressive osteoarthritis and total joint replacement. Phase III clinical trials are also underway evaluating tanezumab and chronic low back pain.
TrkB receptors and the neurotrophin BDNF have roles in both central and peripheral pain mechanisms. BDNF supports neuronal growth and is upregulated in the central nervous system in chronic pain states. The peripheral role of TrkB and BDNF in pain is increasingly being studied. TrkB receptors in the synovial tissue have been associated with increased levels of osteoarthritic knee pain. An animal study employing rodent models of osteoarthritis compared intraarticular injections of exogenous BDNF and TrkB-Fc chimera (proposed to sequester endogenous BDNF). Findings supported the hypothesis that BDNF and TrkB receptors play a role in the maintenance of chronic pain due to peripheral joint osteoarthritis and are a potential therapeutic target.
TrkC receptors and the neurotrophin NT-3 have been shown to modulate pain responses with a variety of proposed mechanisms. In animal studies, NT-3 and TrkC receptors had modulatory effects on neuropathic pain via their impact at the dorsal root ganglia.
Oxytocin
Oxytocin is a nonapeptide hormone that is primarily synthesized in the hypothalamus, transported to the posterior pituitary gland, and subsequently released into peripheral circulation. There is great diversity in the physiologic roles oxytocin plays, ranging from lactation to social attachment. Regarding pain transmission, oxytocin plays a role at peripheral, spinal, and supraspinal levels. The exact mechanism is evolving over time, with reports that oxytocin has analgesic effects via the central endogenous opioid system, while others suggest that the combined actions of oxytocin, GABA, and TRPV1 contribute to oxytocin’s analgesic effects.
Various modes of oxytocin administration have been studied for therapeutic treatment of pain. A Phase I safety assessment of intrathecal oxytocin was conducted in a dose-escalating manner. One subject in the study experienced focal neurologic symptoms and transient cardiovascular depression; however, overall, the study did not demonstrate a high incidence of effects on blood pressure, heart rate, QT interval, serum sodium, or neurologic symptoms. The intrathecal oxytocin administered was not found to produce analgesia when acute noxious heat stimuli were introduced to healthy subjects. A placebo-controlled, double-blind, within participants, crossover investigation evaluated the effect of intranasal oxytocin administration on acute cold pressor pain. Subjects receiving a 40-IU dose of intranasal oxytocin reported lower pain intensity, unpleasantness, and pain descriptors after a cold pressor task versus placebo.
Mammalian Target of Rapamycin Pathway
The mammalian target of rapamycin (mTOR) pathway plays an integral role in cell growth and proliferation. There are two complexes: mTOR complex 1 (mTORC1) and mTOR complex 2 (mTORC2). mTORC1 is switched on by oncogenic signaling pathways and can be hyperactive in many forms of cancer. Its most established current role as a therapeutic target is demonstrated by mTOR inhibitors, which are FDA approved for the treatment of cancer.
The mTOR pathway is also being investigated for its potential role in pain. This includes the development and maintenance of chronic pain, opioid-induced tolerance, and opioid-induced hyperalgesia. Microinjections of rapamycin (mTORC1 inhibitor) to the insular cortex of rats after sciatic nerve lesion caused an increase in pain threshold and reduction in mechanical hypersensitivity. A retrospective review evaluated three cases of patients with neurofibromatosis 1 and painful plexiform neurofibromas. Administration of the immunosuppressive agent and mTOR inhibitor sirolimus not only stabilized the cancerous mass but also resulted in alleviation of pain symptoms. These examples highlight that mTOR inhibitors being utilized in the treatment of certain cancers may be a potential treatment for chronic pain.
Janus Kinase/Signal Transducer and Activator of Transcription 3 Signaling Pathway
The Janus kinase/signal transducer and activator of transcription 3 signaling pathway (JAK/STAT3 pathway) has a large number of roles in the body, including embryonic development, stem cell maintenance, hematopoiesis, and inflammatory responses. Signals are transduced via cytokines, interleukins, and growth factors acting through transmembrane receptor families. An FDA-approved JAK/STAT inhibitor, tofacitinib, is utilized in the treatment of rheumatoid arthritis. The JAK/STAT pathway also has an established role in certain cancers.
The JAK/STAT3 pathway has been found to have a potential role in the treatment of pain. Ligustrazine is an active ingredient in the Chinese medicine Ligusticum chuanxiong Hort and has been used in the treatment of cardiovascular disease. Its role in pain is also becoming more established. In a rat model of sciatic nerve chronic constriction injury, ligustrazine decreased neuropathic pain via JAK/STAT3 pathway inhibition.
Noninvasive Neuromodulation
The field of brain neuromodulation includes both invasive and noninvasive stimulation to artificially stimulate or inhibit brain regions involved in specific brain signaling, leading to enhanced neuroplasticity. Examples of invasive brain stimulation are deep brain stimulation and motor cortex stimulation; both have shown to be beneficial in reducing pain, but they also have limitations of cost and greater potential complications. As a result, noninvasive forms of brain neuromodulation are also being studied, including transcranial magnetic stimulation (TMS), transcranial direct current stimulation (tDCS), and transcranial ultrasound (TUS).
TMS is increasingly being studied as a noninvasive form of neurostimulation for treating neurologic disorders impacting brain function and as a noninvasive neurostimulation treatment for pain. It was first utilized in 1985 and employs electromagnetic induction to reach neighboring cells. Application can be in the form of single or multiple stimulation pulses. Repetitive TMS (rTMS) utilizes a repetition of multiple pulses rapidly applied and is the primary form of TMS in therapeutic use. The frequency of rTMS also plays a role on the subject’s physiologic response to treatment. High-frequency rTMS (5Hz or faster) usually increases excitability, whereas low-frequency rTMS (approximately 1Hz) usually decreases excitability.
One example of the use of rTMS in the treatment of pain involves diabetic neuropathic pain. In a study, high-frequency rTMS was applied to subjects in two groups with diabetic neuropathy: insulin-dependent and noninsulin-dependent. Visual analog scale for pain and nerve conduction studies had significant improvements in both subject groups when comparing values prior to and following rTMS treatment. Notably, there were sustained analgesic effects in a portion of subjects who completed extended follow-up of 5 weeks. The proposed mechanism for the reduction in pain following rTMS treatment was attributed to enhanced cortical plasticity and inhibition of pain-processing pathways.
Another study of high-frequency rTMS in subjects with painful diabetic neuropathy highlighted an interesting response. Cortical inhibition was decreased in diabetic patients with neuropathic pain versus in diabetic patients without neuropathic pain. It was suggested that rTMS strengthened the defective intracortical inhibition and subsequently improved neuropathic pain symptoms.
tDCS is another noninvasive technique that utilizes an electrical current conducted to the brain through electrodes on the scalp. The application of the electrical current is used to modulate brain activity, induce neuroplasticity, and thereby modify brain function. Animal studies in rats showed that electrical currents induced cortical activation or inhibition that was stable after the end of stimulation and led to intracellular modifications similar to long-term potentiation and long-term depression ; therefore, it can both excite and inhibit brain activity, and the results can be long lasting.
tDCS has been investigated for the treatment of numerous conditions, including drug addiction, major depression, attention deficit hyperactivity disorder, schizophrenia, Alzheimer’s disease, and disorders of consciousness. It has also been investigated as a treatment for neuropathic pain from spinal cord injuries, multiple sclerosis, trigeminal neuralgia, poststroke pain syndrome, and amputation, and has had promising short-term results when compared with a placebo. , It is also currently being investigated for treatment of carpal tunnel syndrome to assess improvement in pain and function. tDCS is being compared with trans-spinal DCS for chronic pelvic pain due to endometriosis, assessing the differences between cortical modulation and spinal modulation.
There are some advantages of tDCS over TMS, in that tDCS is relatively cheaper, portable, and user-friendly. tDCS is also considered safer, as TMS has been associated with seizures; furthermore, tDCS has been postulated to have longer-lasting effects on cortical activity.
Combining tDCS with other techniques and modalities, such as MRI, TMS, or TUS, is currently under investigation, as combinatorial modalities may have an increased potential for targeting the current, and therefore, increased cortical modulation. High-definition tDCS is being developed for improved stimulation of cortical regions.
As noted, TUS is another modality that is currently under investigation for the treatment of pain. Brain activity can be modulated using ultrasound as measured with fMRI and EEG. To date, the brain targets that have been modulated include the motor cortex, somatosensory cortex, thalamus, caudate, and visual cortex, and the majority of studies have been performed on healthy volunteers assessing perception and behavior. One study specifically looked at ultrasound stimulation over the posterior frontal cortex, contralateral to the most pain in patients with chronic pain, and showed reduced pain and improved mood compared to sham controls.
TUS is believed to be safe, with minimal side effects. The most common reported side effects are neck pain, headache, sleepiness, muscle twitch, and itchiness, all of which were temporary. However, both short- and long-term safety studies need to be performed in humans. Compared with TMS and tDCS, TUS could be superior, due to increased spatial resolution and depth perception. , TUS can target superficial and deep cortical tissues as well as subcortical targets. In addition, although all the modalities can lead to modulation of brain activity, only TUS has been investigated for its ability to ablate tissues.
TUS is currently FDA approved for thalamotomy, as a treatment for medication refractory essential tremor. A feasibility study currently underway assesses thalamotomy for the treatment of chronic trigeminal neuropathic pain, as well as TUS thalamotomy for neuropathic pain due to lumbar radiculopathy, spinal cord injury, or phantom limb pain. Investigations of combinatorial noninvasive modulation are also underway to assess TUS plus tDCS for painful diabetic neuropathy, and the effects of pain perception and functional limitations in chronic low back pain and knee osteoarthritis. ,
TUS is also being combined with magnetic resonance (MR) for focusing the ultrasound to the appropriate cortical area. Interestingly, ultrasound is not only being investigated transcranially for the treatment of pain but peripherally as well. Current research is underway assessing MR-guided ultrasound for pain relief in residual limb neuromas in amputees, bone metastasis, facet arthropathy, as well as osteoarthritis of the knee, hip, and hand. ,
Future research will need to elucidate the mechanism of action for TMS, tDCS, and TUS. It will need to optimize treatment parameters, including location of treatment, dosing, frequency, intensity, and duration. Lastly, it will need to assess both short- and long-term safety and effects of cortical structural changes.
Gene Therapy
Gene therapy utilizes a vector to deliver the intracellular instructions from a therapeutic gene to target cells. The target cells subsequently express the encoded peptides and proteins to produce the desired substance at their site of action. The potential benefits of gene therapy and pain are being investigated.
To investigate a treatment for neuropathic pain induced by spinal nerve transection in mice, a gene therapy was engineered using lentiviral vectors targeting dorsal root ganglion tissues. This was found to silence TNF-alpha and relieve neuropathic pain. Another animal study evaluated intraarticular administration of an adeno-associated virus (AAV) vector, targeting genes encoding matrix metalloproteinase 13 (MMP13), Interleukin-1 (Il-1), and nerve growth factor (NGF) in a surgically induced osteoarthritis mouse model. Although osteoarthritic pain improved, articular cartilage destruction and osteophyte growth increased.
Gene therapy has many potential advantages, warranting exploration into new viral vectors and signaling pathways. These advantages include the longevity of benefits, potentially reducing the need for frequent medication dosing, and the increased specificity for target tissues, potentially limiting side effects experienced from the many systemic pharmaceutical treatments we currently use in the treatment of pain.
Conclusion
An increase in dedicated initiatives for pain research is creating an important structure to explore promising advances in the field. New research is being directed at improving our understanding, assessment, and treatment of pain processes. As each person’s experience of pain is unique, identifying ways to further individualize treatment will be essential. This includes not only developing novel techniques and technologies but also understanding their roles alone, in combination, and in unique individuals.
Novel treatments are being discovered all the time, with only a small number able to be discussed in this chapter. Multiple resources should be reviewed to recognize the diverse perspectives being applied to pain research and for updates in the field. Awareness of ongoing advances can be fostered via many avenues. These include reviewing clinical trials, exploring newly funded initiatives, and meeting with peers in the field. As pain continues to have a widely detrimental impact on patients, their environment, and the healthcare system, the current expansion in pain research provides an exciting opportunity for truly consequential advances for the future of pain management.
References
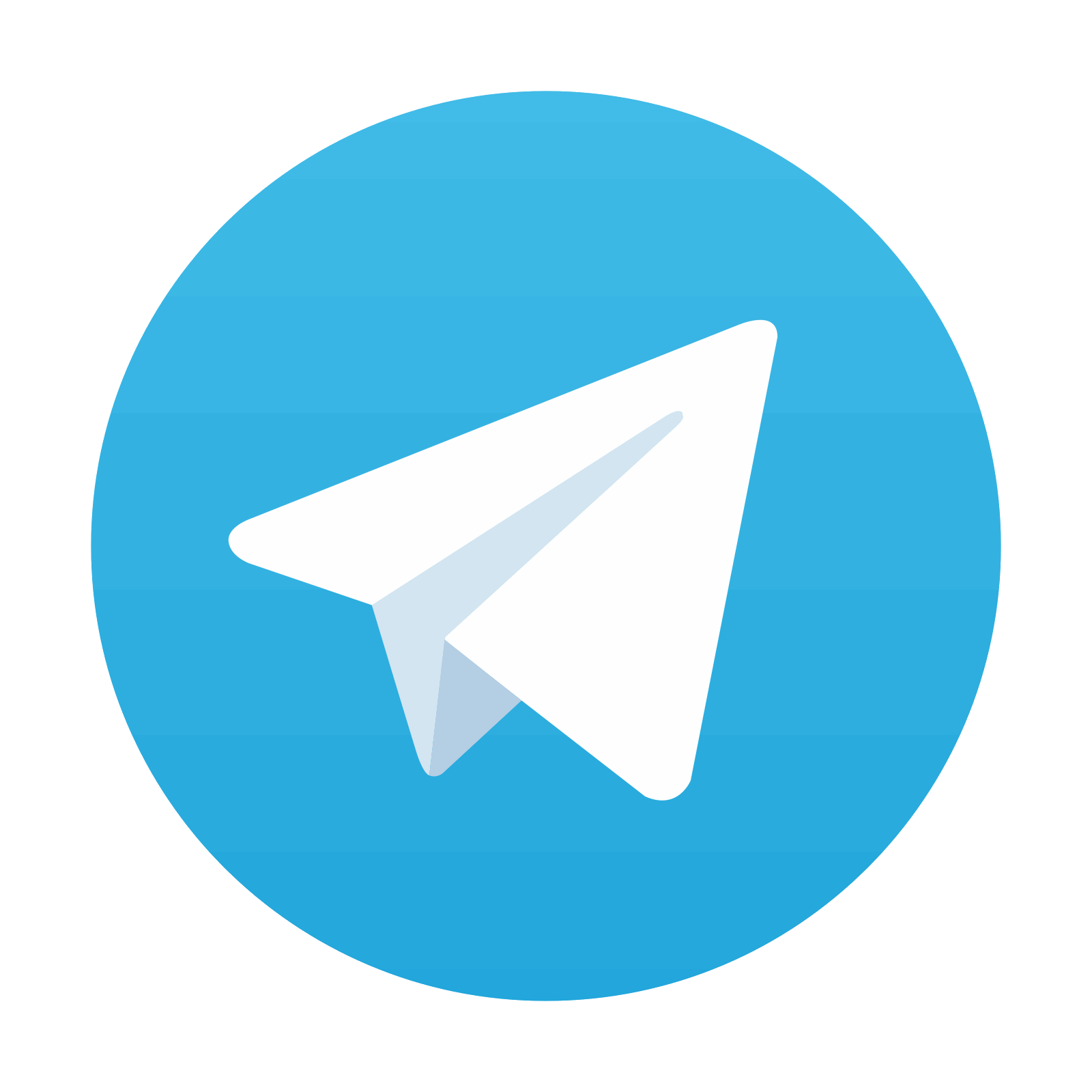
Stay updated, free articles. Join our Telegram channel

Full access? Get Clinical Tree
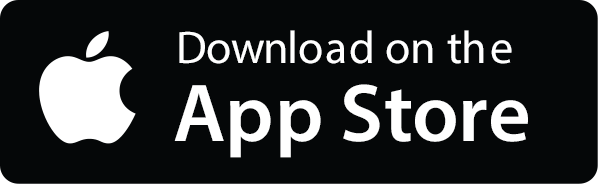
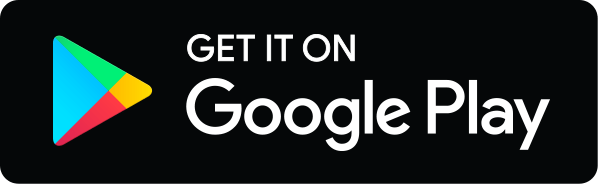
