C. albicans is the most common Candida species involved in invasive candidiasis, followed by C. glabrata, C. tropicalis, and C. parapsilosis. Other species are less common and often associated with underlying malignancy or chemotherapy. Whereas C. tropicalis and C. glabrata are found largely in adults, C. parapsilosis is the leading pathogen in the neonatal population. In many tertiary care centers, C. glabrata has surpassed C. albicans to become the most common Candida sp in invasive candidiasis, accounting for up to 35% of all candidemias (15,16). Among non-albicans Candida species, C. krusei and C. glabrata are particularly important because of their resistance and decreased susceptibility to fluconazole, respectively (17).
Diagnosis
Clinical manifestations of invasive candidiasis are often nonspecific, and fever is frequently the first and only sign. Signs that should raise concern for candidemia are papulopustular or macronodular skin lesions or ocular involvement such as chorioretinitis or endophthalmitis. Symptoms of sepsis, severe sepsis, or septic shock can be presenting symptoms in critically ill patients. Deep-seated infections often present with findings localized to the particular tissue site.
The diagnosis of invasive candidiasis is a challenge due to nonspecific clinical manifestations and the low sensitivity of microbiologic culture techniques. Furthermore, Candida spp are common colonizers of humans, which often makes it difficult to differentiate between colonization and true infections when organisms are isolated from the urine and other nonsterile sites.
Cultures. Blood culture is the gold standard and should be routinely obtained among patients who have suggestive signs and symptoms or those at high risk for invasive candidiasis. However, it is positive in less than 50% of patients (1). Overall, the sensitivity of blood culture is impacted by the mechanisms of pathogenesis: it is highly sensitive in the setting of CVC-associated candidemia (1), but is much less sensitive when candidemia stems from extracellular sources (deep-seated sites) or from translocation across the gastrointestinal mucosa. Blood culture is further limited by time to positivity, with the median time of 2 to 3 days, ranging from 1 to more than 7 days (1,18). For deep-seated candidiasis, the gold standard of diagnosis is culture of sterilely collected samples, a procedure that can be challenging. In this setting, blood culture sensitivity is only 25%, and blood culture may take up to 4 weeks longer than nonculture tests to turn positive. The poor sensitivity and slow time to positivity of culture are important considerations because delays in antifungal therapy are associated with poor outcomes (19,20).
Given the potential for antifungal resistance among the non-albicans Candida spp, isolates recovered from blood or sterile sites should be identified to the species level. The availability of special fungal media (such as CHROMagar), rapid in situ hybridization techniques, DNA sequencing, and other molecular methods such as MALDI TOF have shown promising results (21,22).
Studies have demonstrated that azole minimum inhibitory concentrations (MICs) correlate with the likelihood of success in treating patients. Nevertheless, antifungal susceptibility testing of Candida is not routinely performed in most clinical laboratories, and it is not considered the standard of care, unlike antibacterial susceptibility testing. In fact, antifungal susceptibility patterns are predictable in most cases based on species and prior exposure to antifungal agents (23). For this reason, it has generally been believed that identification of isolates to the species level is more important than MIC data in the management of individual patients. However, triazole resistance among C. glabrata has risen to a degree that it is difficult to select these agents as therapy in the absence of susceptibility testing. Similarly, resistance among C. albicans, C. tropicalis, and C. parapsilosis has also been increasingly reported, especially at tertiary care institutions where azole agents are extensively used. Cross-resistance to other azoles is often seen, which limits the utility of this class against fluconazole-resistant isolates. For these reason, antifungal susceptibility testing should be incorporated in the clinical microbiology laboratory workflow to guide the management of patients with invasive candidiasis.
Resistance among Candida spp to the echinocandin class of antifungals is uncommon, except for C. glabrata, where the rate of resistance can be as high as 13% at certain institutions (24,25). The most important risk factors for echinocandin resistance among C. glabrata are prior prolonged exposure to this agent, especially in the setting of breakthrough infection. In one study, 36% of echinocandin-resistant C. glabrata were associated with cross-resistance to the triazoles, yielding multi–drug-resistant phenotypes and limiting therapeutic options (26). Amphotericin B resistance is difficult to document using current testing methods. Resistance among C. lusitaniae and C. guilliermondii isolates is well described but not seen with all isolates. Clinicians should probably avoid amphotericin B if elevated MICs are documented. At centers where candidiasis is a particular problem and antifungal use is widespread, it is useful to conduct periodic susceptibility testing to generate an institutional antibiogram. Clinicians should be aware if such reports exist at their institution, as susceptibility patterns against different species can be used to guide empiric antifungal therapy.
Nonculture Diagnostics. Efforts have been devoted to develop non–culture-based diagnostic tests for invasive candidiasis. β D-glucan assay (Fungitell; Associates of Cape Cod, Massachusetts) is a pan-fungal diagnostic assay that detects a nonantigenic cell wall component of most pathogenic fungi, including Aspergillus, Candida, and Pneumocystis, but not Mucorales and Cryptococcus (27). In the United States, the Fungitell assay (BDG) has been approved by the Food and Drug Administration for the diagnosis of invasive fungal infections, with positive and negative tests defined as at least 80 pg/mL and below 60 pg/mL, respectively. Levels of 60 to 79 pg/mL are considered indeterminate. BDG becomes positive earlier than blood culture, and is a relatively sensitive, but nonspecific, test. In meta-analyses, the pooled BDG sensitivity and specificity for diagnosing invasive candidiasis were 75% to 80% and 80%, respectively (28,29). In a few studies evaluating nonneutropenic critically ill patients with candidemia or IAC, the sensitivity and specificity of BDG ranged from 52% to 100% and 58% to 94%, respectively (30). A major drawback of BDG is its low specificity and positive predictive value for invasive candidiasis. Indeed, BDG is not specific for Candida, as it also detects other fungi such as Aspergillus, Fusarium, and Trichosporon. Furthermore, numerous factors might cause false-positive BDG tests, including fungal colonization, disruption of GI mucosa, bacteremia, hemodialysis with cellulose membranes, glucan-contaminated blood collection tubes and surgical gauze, administration of human blood products, and use of certain broad-spectrum antibiotics (1,31). In general, false-positive BDG results are rare in healthy controls, but common in patients with bacteremia and ICU residents (32).
Detection of Candida DNA using polymerase chain reaction (PCR) is a potentially useful diagnostic test that enables early detection of invasive candidiasis. A meta-analysis of 54 studies with 4,694 patients demonstrated that PCR in whole blood had pooled sensitivity and specificity for suspected invasive candidiasis of 95% and 92%, respectively (33). In probable invasive candidiasis, the sensitivity of PCR was 85%, higher than that of the blood culture (38%). To date, PCR data for patients colonized with Candida are limited, but overall, there was trend toward lower specificity (30). Major limitations of PCR are the lack of standardized methodologies and multicenter validation of assay performance (33,34). In 2015, U.S. Food and Drug Administration approved the T2Candida assay (T2 Biosystems, Massachusetts), which amplifies and detects Candida DNA within whole blood by PCR and T2 magnetic resonance (T2MR), respectively. The test is able to detect five most common Candida spp (C. albicans, C. glabrata, C. parapsilosis, C. tropicalis, and C. krusei). Published clinical study on the T2Candida assay is limited to a report of 250 contrived blood specimens spiked with five Candida spp and 1,501 control patients with Candida-negative blood culture (35). In this setting, the sensitivity and specificity were 91% and 99%, respectively. A potential advantage of T2Candida assay is time to test positivity (mean time of 4.4 hours) compared with automated blood culture (2–5 days). A potential advantage of T2Candida over BDG is its ability to speciate Candida and to identify poly-Candida fungemia. Nevertheless, studies are required to evaluate the performance of T2Candida assay in real time among patients with invasive candidiasis.
The overwhelming majority of studies of nonculture diagnostics have focused on candidemia. Nonculture tests are unlikely to be more sensitive than blood cultures for diagnosing primary candidemia, but may shorten turn-around times. A potential role of nonculture tests is in the diagnosis of blood culture–negative, deep-seated candidiasis (34,36). Assays may accomplish this goal by detecting Candida antigens or DNA that are released from deep tissue sites or that persist in blood after viable cells are eliminated (37). Indeed, two recent studies assessed the role of BDG among prospectively enrolled patients with deep-seated candidiasis (34,38), in both studies, the sensitivities of BDG (65% and 67%, respectively) were higher than blood culture (21% and 7%, respectively). Along the same line, PCR has also been shown to be superior to BDG in diagnosing deep-seated candidiasis. In a prospective study of 24 patients with deep-seated candidiasis in whom samples were collected at the same time for testing, the sensitivities were 88% with PCR, 62% with BDG, and 17% with blood culture (34). These studies suggest that nonculture diagnostics may be useful adjuncts to blood cultures, and identify some patients who are currently undiagnosed.
Treatment
Invasive Candidiasis. The major antifungal agents and the current guidelines for management of invasive candidiasis are summarized in Tables 89.2 and 89.3, respectively (39). In general, the selection of an antifungal agent for the treatment of invasive candidiasis should take into account the severity of illness of patients, the target organ involved, history of recent azole or echinocandin use, previous episodes of candidiasis, and antifungal drug intolerance. Echinocandin should be the first-line treatment for critically ill patients with invasive candidiasis. This class of antifungal exhibits potent candidacidal activity and is highly efficacious in the treatment of invasive candidiasis in randomized comparative trials (40). It also has favorable tolerance and safety profile. The only limitation is the need for intravenous administration. The three echinocandins—anidulafungin, caspofungin, and micafungin—are considered therapeutically equivalent. Fluconazole can be considered an acceptable alternative to echinocandin for noncritically ill patients with no recent azole exposures and who are considered unlikely to be infected with azole-resistant Candida isolates. Transition from an echinocandin to fluconazole can be safely done for patients who are clinically stable and who are infected with fluconazole-susceptible Candida isolates. Voriconazole offers little advantage over fluconazole during initial therapy for invasive candidiasis, but has a role as step-down oral therapy for selected cases of invasive candidiasis due to C. krusei. Amphotericin B formulations can be considered an acceptable alternative for patients not able to tolerate an echinocandin or azole agents, or who are infected with multi–drug-resistant Candida species. Lipid formulations of amphotericin B are better tolerated than amphotericin deoxycholate and have less nephrotoxicity.
All patients with candidemia should have an ophthalmologic examination to rule out retinal involvement. In addition, all vascular catheters should be removed if possible. Candida spp tend to form biofilms on catheters, which can render otherwise susceptible isolates resistant to antifungal agents.
Candida Recovered from Urine or Sputum/Bronchoalveolar Lavage. As mentioned above, Candida spp are part of endogenous flora and frequent colonizers of mucosal surfaces. In the ICU setting, urine and sputum are the two most common sites of colonization.
Candida in the Urine. Candida spp are now the most common organisms recovered from the urine of surgical ICU patients. The risk factors include urinary catheters, old age, and receipt of antibacterial agents. Unlike the assessment of bacteriuria, colony counts and urine analysis are not helpful in deciding whether candiduria is of clinical importance (41,42). Many studies have demonstrated that asymptomatic candiduria in the low-risk patient is of little clinical relevance and should not be treated. In a small subset of patients, candiduria is a marker for invasive candidiasis. Treatment is indicated for symptomatic patients and those who are neutropenic, have undergone a urologic manipulation, or received a kidney transplant. Treatment entails removal of the urinary catheter and therapy with a systemic antifungal agent (fluconazole or amphotericin B) for 7 to 14 days. Echinocandins do not penetrate well, and voriconazole and isavuconazole do not accumulate in active forms in the urine, thus should not be used for renal candidiasis. In the event that catheter removal is not possible, changing the catheter might be of benefit and should be performed. For cystitis due to fluconazole-resistant Candida spp, an alternative treatment is amphotericin deoxycholate (50 mg/mL) bladder irrigation for 5 days.
Candida in the Sputum. Specimens from the airways—sputum, tracheal aspirates, and BAL—are frequently contaminated with oropharyngeal flora, including Candida spp. Despite the frequency with which Candida spp are isolated from the respiratory tree of ICU patients, primary Candida pneumonia is extremely rare; cases are generally encountered among neutropenic hosts. The diagnosis of Candida pneumonia requires evidence of parenchymal invasion by hyphae on a biopsy specimen. Antifungal therapy should not be instituted in response to Candida isolates recovered from respiratory samples. In fact, strategies of not identifying or reporting Candida spp in respiratory samples decrease length of stay, hospital costs, and unnecessary antifungal therapy, without any negative effects on the accurate diagnosis of Candida pneumonia or patient outcome (43).
Controversies
Given the nonspecific clinical manifestations, low yield of blood cultures, and high mortality rates of invasive candidiasis, investigators have studied three treatment strategies in the absence of a definitive diagnosis:
- Prophylactic strategy: Administration of an antifungal agent at a period of high risk to prevent candidiasis.
- Preemptive strategy: Administration of an antifungal agent to treat suspected invasive candidiasis based on particular warning signs.
- Empiric therapy: Administration of an antifungal agent in persistently febrile patients without a known source or with no response to appropriate antibacterial agents.
Prophylactic Strategy. The role of prophylactic antifungal therapy is controversial, as results from several clinical trials are contradictory. The most popular antifungal agent used for prophylaxis is fluconazole, given its benign side effect profile and good absorption. Five meta-analyses showed that antifungal prophylaxis (mostly fluconazole) reduced invasive candidiasis (44–47), but only one analysis showed reduction in mortality (47). It should be pointed out that only a small subset of patients is at sufficient risk for invasive candidiasis to justify this strategy. Thus, universal prophylaxis to all ICU patients is not warranted. To date, the specific patient populations that would benefit most are not clearly defined. However, populations in which antifungal prophylaxis may be useful include patients with gastric perforation, gastrointestinal dehiscence and/or leakage, status-post multiple abdominal procedures, surgical treatment for pancreatic infection, and liver transplant recipients with significant risk factors for invasive candidiasis (choledochojejunostomy, prolonged operative time, receipt of substantial amount of blood products, Candida infection within the preceding 3 months, and living donor transplant).
TABLE 89.2 Available Systemic Antifungal Agents |
![]() |
TABLE 89.3 Recommended Antifungal Agents against Invasive Candidiasis | |||
![]() |
Preemptive Strategy. There have been two randomized trials evaluating preemptive echinocandin antifungal approaches among ICU patients. The first trial utilized a clinical prediction rule to identify medical–surgical ICU patients with invasive candidiasis (48). There was a trend toward a reduction in proven or probable invasive candidiasis in the caspofungin arm of the trial (9.8%, 10/102) versus the control arm (16.7%, 14/84; p = 0.14); however, there was no difference in mortality between the two groups. The second trial targeted high-risk surgical ICU patients with intra-abdominal infection for randomization to micafungin or placebo (49). Although patients in the micafungin arm had significantly reduced Candida colonization indices, there were no differences in the rates of invasive candidiasis, mortality, or organ failure between the 2 arms. Taken together, these studies demonstrate that precise ICU subgroups that may benefit from preemptive antifungal therapy against invasive candidiasis are not yet identified.
Empiric Strategy. Empiric administration of antifungal agents, although widely employed by some practitioners, has not been validated in clinical trials. A randomized controlled trial evaluating empiric fluconazole among medical–surgical ICU patients with persistent fevers despite broad-spectrum antibiotics failed to show significantly reduced rates of invasive candidiasis (5% in the fluconazole arm and 9% in the placebo arm) or improved outcomes. This trial suggests that empiric antifungal therapy solely based on nonspecific clinical features is unlikely to be of benefit.
In summary, optimal approaches for incorporating clinical prediction rules and/or nonculture diagnostics into management strategies remain undefined. An important step moving forward is the identification of a biomarker with substantial negative predictive value to safely discontinue empiric antifungal therapy among patients at low-to-moderate risk of IC (pretest likelihood of 3%–10%) and positive predictive value to trigger preemptive therapy among patients at moderate-to-high risk of IC (pretest likelihood about 10%–35%) (36). In clinical settings where pretest likelihood for IC is high (>25%–35%), the optimal strategy might be universal prophylaxis. On the other hand, in clinical settings where pretest likelihood is very low (<3%), routine nonculture testing or prophylactic or preemptive therapy might not be necessary (36).
Infections Caused by Aspergillus Species (Aspergillosis)
Aspergillus spp are less common human pathogens in the ICU than Candida spp but cause greater morbidity and mortality. These molds are ubiquitous in the environment; in normal hosts, they are generally saprophytes that colonize the bronchopulmonary tree. Most infections caused by Aspergillus spp originate from the inhalation of fungal spores into the lungs. Cases of direct skin inoculation of Aspergillus have been described in association with the insertion of intravenous devices or the taping of arm boards to the extremities. Patients with severe burns can also develop local burn wound infections. Regardless of the portal of entry, any local form of aspergillosis can disseminate to various sites if host immune function is impaired.
Almost any organ may be involved in disseminated aspergillosis, including integument (onychomycosis, cutaneous aspergillosis), ear (otomycosis), respiratory tract (sinusitis, pneumonia, empyema), heart (endocarditis, myocarditis), gastrointestinal tract (GI, hepatosplenic aspergillosis), central nervous system (CNS) (cerebral aspergillosis, meningitis), eye (endophthalmitis), bone (osteomyelitis, mediastinitis), and so forth. The lungs and sinuses are the two most common primary sites of aspergillosis; the CNS is the most common secondary site.
The four classical clinical syndromes of pulmonary aspergillosis are presented in Table 89.4. This section will focus on the two syndromes most commonly encountered in ICU settings: chronic necrotizing pulmonary aspergillosis (CNPA) and invasive pulmonary aspergillosis (IPA). In both of these diseases, Aspergillus spp invades tissue and blood vessels, causing necrosis and possibly disseminating to the brain and elsewhere. Of note, entities similar to allergic aspergillosis and invasive aspergillosis (IA) are also found in the sinuses.
TABLE 89.4 Clinical Spectrum of Aspergillosis |
![]() |
Pathophysiology
The major risk factors for IA include neutropenia and other defects of the immune system (Table 89.5). IA is estimated to occur in 5% to 13% of patients who have undergone hematopoietic stem cell transplant (HSCT), 5% to 25% of patients who have received SOT, and 10% to 20% of patients receiving intensive chemotherapy for leukemia. Other well-recognized risk factors for IA are chronic granulomatous disease and other immunodeficiencies, cystic fibrosis, the use of high-dose corticosteroids, and other conditions associated with cell-mediated immune defects such as acquired immunodeficiency syndrome (AIDS) and the use of TNF-α-inhibitors to treat rheumatologic and other autoimmune diseases. The disease is not as common in patients with less profound immunosuppression and exceedingly uncommon in immunocompetent hosts. Nevertheless, Aspergillus has been increasingly recognized as a pathogen of critically ill patients without the classical risk factors that are summarized in Table 89.5 (50). Indeed, fatal IA has been reported among patients with no known immunosuppression who develop sepsis and multiorgan dysfunction syndrome (MODS). It is speculated that sepsis displays a biphasic immunologic pattern: an early hyperinflammatory phase followed by a compensatory anti-inflammatory response syndrome/immunoparalysis state (51). Patients colonized with Aspergillus might develop IA during sepsis-induced immunoparalysis state. IPA can also complicate influenza (52–54), and has a predilection for patients with underlying comorbid conditions. Corticosteroid therapy during hospitalization has been linked to worse outcomes in influenza-associated IPA (54). Influenza A causes necrosis of columnar ciliated epithelium, rendering the airways susceptible to secondary opportunistic pathogens; it also suppresses phagocytosis and killing of organisms by alveolar macrophages. With worsening pneumonia following influenza A, Aspergillus should be added to the list of potential secondary pathogens such as Staphylococcus aureus and Streptococcus pneumoniae, particularly if there is no evidence of secondary bacterial pneumonia.
Critically ill patients with Child C liver cirrhosis and those with chronic obstructive pulmonary disease (COPD) treated with systemic and/or inhaled corticosteroids are recognized to be at risk for IA (50,55–61). Low doses of steroids and brief courses of high-dose steroids have been shown to cause an accelerated course of IPA in patients with underlying lung disease such as COPD, asthma, sarcoidosis, and berylliosis (62). IPA complicating COPD has also been rarely described outside the setting of steroid use (61,63). Finally, diabetes, end-stage heart failure, malnutrition, alcohol abuse, severe burns, prolonged ICU stays, and near drowning have all been described as host factors for IA (50,57,64).
Although there are over 100 species of Aspergillus, only a few cause diseases in humans. The most common species causing invasive infection is Aspergillus fumigatus; A. flavus, A. terreus, A. nidulans, A. lentulus, and A. niger are less common causes of invasive disease. Avicularia versicolor is usually considered an environmental contaminant. However, with the expansion of the immunosuppressed population, the list of esoteric species implicated in clinical infection continues to increase; thus, even previously “nonpathogenic” species can cause true disease in the right clinical setting.
Diagnosis
Culture and Histopathology. The diagnosis of IA is problematic. Since Aspergillus spores are ubiquitous, they are common colonizers of the bronchopulmonary tree. A definitive diagnosis, therefore, requires histologic evidence of tissue invasion by hyphal elements, as well as culture of the organism. It should be noted that the sensitivity of tissue culture and biopsy in diagnosing IA is low (e.g., 50% and 30% for bronchoalveolar lavage fluid [BALF] culture and lung biopsies, respectively). Moreover, recovery of Aspergillus from the blood is extremely rare, with a sensitivity of approximately 5% in cases of IA. In immunocompromised hosts, a positive culture from a respiratory sample (sputum or BAL) is highly associated with invasive pulmonary disease.
TABLE 89.5 Common Predisposing Factors to Invasive Aspergillosis | ||
![]() |
The Clinical and Laboratory Standards Institute (CLSI) has developed standardized methods for performing susceptibility testing on filamentous fungi. However, MICs should be interpreted with caution, as interpretive breakpoints that correlate with clinical outcomes do not currently exist. Susceptibility testing of Aspergillus spp and other molds should therefore not be routinely performed, but reserved for cases where there is clinical failure despite optimal antifungal therapy, or for epidemiologic purposes.
Nonculture Diagnostics. Nonculture diagnostic markers, if sufficiently sensitive and specific, should theoretically allow for the early, rapid diagnosis of fungal infections, thus resulting in the earlier initiation of antifungal therapy. Several markers exist for the diagnosis of IA. We will focus on galactomannan (GM), BDG, and PCR.
GM, a heteropolysaccharide cell wall component of Aspergillus, can be detected in the blood and BALF. A double-sandwich enzyme-linked immunosorbent assay (ELISA) (Platelia Aspergillus enzyme immunoassay, Bio-Rad Laboratories, Hercules, CA, USA) has been approved by the FDA for the diagnosis of IA. The cut-off value for positivity recommended by the manufacturer and FDA is an optical density index of 0.5. However, the best cut-off for defining and positive result continues to spark debate (65,66). The performance of serum and BALF GM varies widely across different clinical settings, and has been most extensively studied in neutropenic patients with hematologic malignancy and HSCT recipients. In two large meta-analyses, the pooled sensitivity and specificity of serum GM for patients with proven or probable IA were 71% and 82% to 89%, respectively (67,68). However, these values showed marked variability across patient populations, study designs, and definitions of IA. The serum GM test is clearly superior in neutropenic patients with hematologic malignancy and HSCT recipients in whom the sensitivity was 70% and 82%, respectively, while the specificity was 92% and 86%, respectively (67). It performs poorly in SOT recipients with IA, in whom the sensitivity and specific are 22% and 84%, respectively (67).
GM testing in BALF is more sensitive than in serum (67–69). The increased sensitivity may come at the price of decreased specificity, particularly in lung transplant recipients, in whom positive GM tests cannot differentiate between invasive disease and airway colonization (70,71). There are limited data investigating the use of GM in nonclassic hosts, including the critically ill and patients with COPD. In general, the serum test performs poorly in these patients (sensitivity of 42%–51%) (72), whereas GM within BALF is more sensitive (88%) (59).
False-positive GM results have been reported with the use of antibiotics such as amoxicillin–clavulanate, colonization with other molds (Penicillium, Fusarium, Paecilomyces, Histoplasma, Blastomyces), and the use of Plasmalyte in BAL. Notably, current formulations of the antibiotic piperacillin/tazobactam, which was historically linked to false-positive GM assays, no longer contain GM and are thus unlikely to lead to false-positive results (73). Both serum and BAL GM can be rendered falsely negative with the receipt of mold-active antifungal therapy.
There is limited information on the diagnostic performance of BDG specifically for IA, but a retrospective study of patients with malignancy demonstrated a sensitivity and specificity of 88% and 90%, respectively. Sensitivity among ICU patients and SOT recipients is lower (66%). As mentioned above, BGD is limited by low specificity (about 44%) and positive predictive value (10%–12%); combining both BG and GM assays or higher BDG cut-off values may result in greater accuracy in the diagnosis of IA.
Although PCR assays are promising diagnostic tests for IA, their use in clinics remains limited because few assays have been standardized and validated, and the role of PCR testing in patient management is not established. Overall, Aspergillus PCR is more sensitive than culture in blood and respiratory fluids. In a meta-analysis, the sensitivity and specificity of serum or whole-blood PCR assays for IA were 84% and 76%, respectively (74). The sensitivity of Aspergillus PCR on BAL fluid was higher than within blood, but in many instances its specificity was lower. The lower specificity in BAL might be due to the fact that lungs are often colonized by Aspergillus, and that PCR is not able to differentiate colonization from disease or to distinguish different Aspergillus spp. The high negative predictive value of BAL PCR (usually ≥95%) suggests a role in ruling out IPA.
Radiology. In neutropenic patients and bone marrow transplant recipients, high-resolution CT scan of the chest has become an important adjunct to the diagnosis of IPA. One or more nodules surrounded by halo signs (ground glass opacity or haziness) are early findings of angioinvasive mold infections; cavitation with air-crescent sign is a late finding (75). Although these lesions are highly suggestive of IPA in high-risk patients, it should be emphasized that other infections (other fungi, Nocardia, and so forth) can also present with halo signs. In one study, classic CT scan findings led to the earlier diagnosis of IPA, more timely administration of antifungal therapy, and improved outcome.
Of note, halo signs have not been validated outside patients with hematologic malignancies and HSCT recipients. In SOT recipients, for instance, ground glass opacification, peribronchial consolidation, macronodules, and mass-like consolidations are commonly seen in IPA, and the halo and air-crescent signs rarely occur (76). Similar findings have been observed in COPD patients, or those who are critically ill but otherwise immunocompetent, with IPA, who develop nonspecific consolidations, nodules, and cavities but almost never have halo or air-crescent signs (55,60,72).
Treatment
Voriconazole is the first-line therapy against IA, as it has been proven superior to conventional amphotericin B. Isavuconazole might be an alternative therapeutic agent as it was shown to be noninferior to voriconazole for the primary treatment of suspected invasive IA in a randomized comparative study (77). In that study, isavuconazole was better tolerated than voriconazole, with fewer study drug–related adverse events. To date, there have not been head-to-head comparisons of voriconazole versus lipid formulations of amphotericin B. Therapy is generally prolonged for at least 6 to 12 weeks or until the primary infection is resolved. The role of other systemic antifungal agents is summarized in Table 89.6 (78,79). Alternative therapies include lipid formulations of AmB. Echinocandins are effective in salvage therapy (either alone or in combination) against IA, but we do not recommend their routine use as monotherapy for the primary treatment of IA (80,81). The combination of an echinocandin and voriconazole for primary therapy should be considered in the setting of severe disease, especially in patients with hematologic malignancy and those with profound and persistent neutropenia (82).
TABLE 89.6 Recommended Antifungal Agents against Aspergillosis | ||
![]() |
Therapeutic drug monitoring (TDM) should be performed once the steady state has been reached to enhance therapeutic efficacy and minimize toxicities potentially attributable to the azoles (itraconazole, voriconazole, and solution formulation of posaconazole). Whether TDM is helpful or necessary with the extended release or intravenous formulations of posaconazole or for isavuconazole is not known at the current time.
Debridement of the involved sinuses or primary cutaneous aspergillosis should be performed in conjunction with systemic antifungal therapy. Recent data show that combined antifungal therapy and surgical resection of single lesions from the lungs or CNS might clear the infection faster than antifungal therapy alone, improve outcome, and prevent reactivation during consecutive chemotherapy courses (85–89). The procedures are generally well tolerated and associated with low rates of complications and mortality.
VIRAL INFECTIONS
Recent years have seen the emergence of unexpected viral diseases with high case fatality rates, including Hantavirus pulmonary syndrome (HPS), West Nile virus (WNV) encephalitis, severe adult respiratory syndrome, Middle East respiratory syndrome coronaviruses (MERS-CoV), and avian influenza. There are several reasons for critical care physicians to be familiar with a range of viral infections and to consider these in their differential diagnoses (90). First, there is a small window of time to effectively intervene with antiviral agents in many of these diseases. Second, the timely identification of persons with potentially infectious viral diseases has significant public health implications and may reduce the risk of transmission to other persons. Third, in the era of long-distance travel, clinicians must recognize previously unfamiliar diseases. Finally, viruses such as those causing hemorrhagic fevers are possible agents of bioterrorism.
In general, viral infections can be diagnosed by several means:
- Serologic tests: The antibody response to viral antigens can be detected in the serum of patients with viral infections. An IgM response usually indicates recent exposure to a virus, whereas the presence of IgG reflects past exposure. Serologic testing, in general, does not provide real-time results to guide clinical management.
- Culture: Several types of cells are available for growing viruses, and no single cell line is appropriate for all of them. Therefore, it is helpful for the laboratory to know which virus the clinician suspects.
- Pathology: Histologic examination of biopsy and autopsy tissues may demonstrate changes that are typical of certain viruses (e.g., DNA viruses usually produce inclusions in the cytoplasm).
- Detection of viral antigens: Viral antigens can be detected in tissues by direct or indirect immunofluorescence using appropriate antibodies.
- Amplification of viral nucleic acids: Small copy numbers of viral DNA and RNA can be detected by PCR and reverse transcription-PCR (RT-PCR), respectively. Real-time amplification methods permit simultaneous detection and quantification of viral nucleic acids.
Table 89.7 lists the leading viruses that might be encountered in the ICU, as of the writing of this chapter. We will review major viral illnesses encountered in critically ill patients, their diagnosis, and treatment. A review of human immunodeficiency virus (HIV) medicine is covered in Chapter 91.
TABLE 89.7 Viral Pathogens Most Likely to Be Encountered in the ICU | ||
![]() |
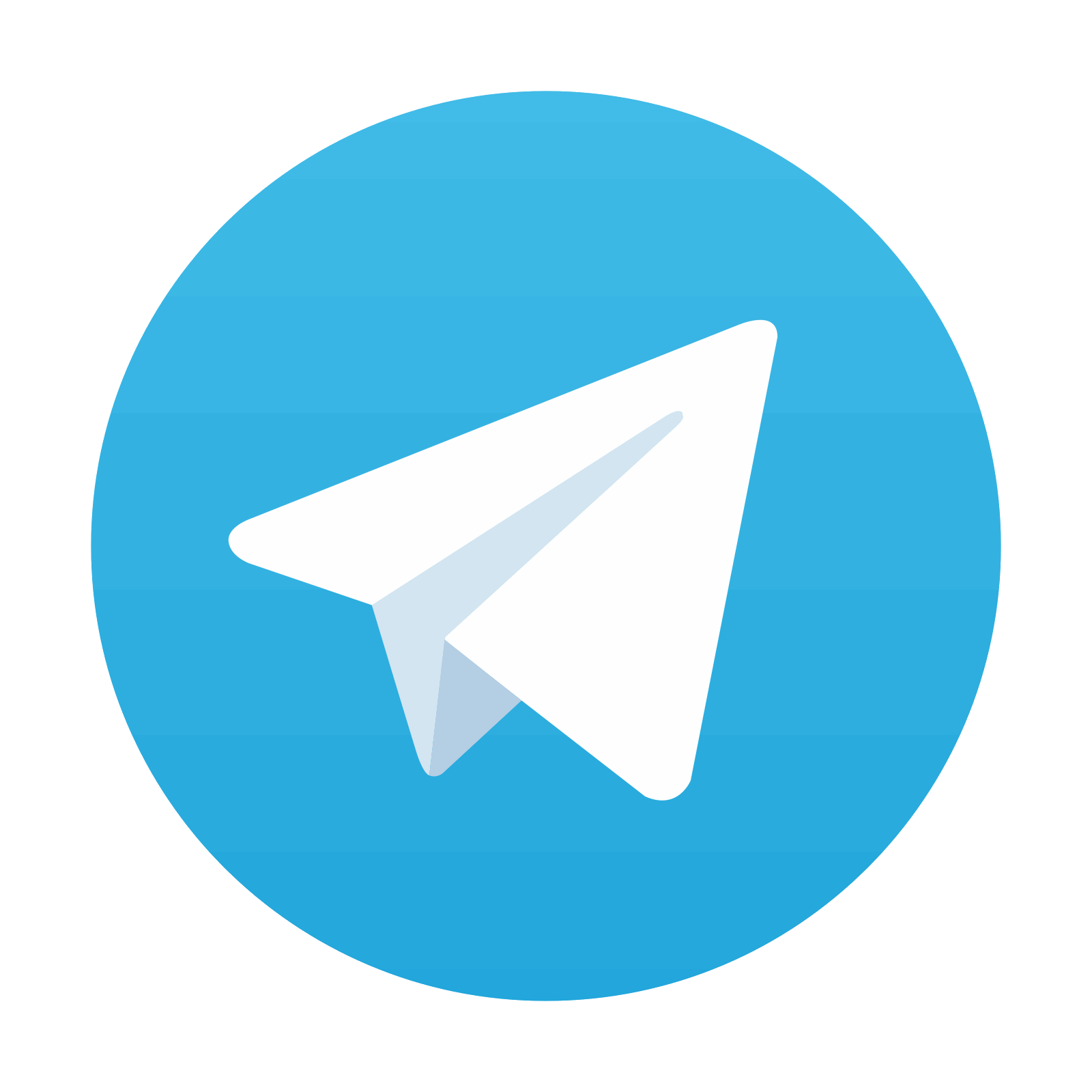
Stay updated, free articles. Join our Telegram channel

Full access? Get Clinical Tree
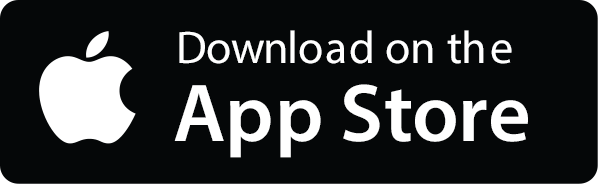
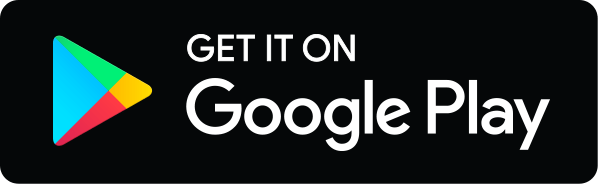