div class=”ChapterContextInformation”>
9. Platelets: Frozen and Freeze-Dried Current Products in Development and Regulatory Licensing Challenges
Keywords
History of plateletsCryopreserved plateletsFrozen plateletsDMSOLyophilized plateletsFreeze-dried plateletsTrehaloseParaformaldehydeUS militaryFDAAbbreviations
- ACD
Anticoagulant Citrate Dextrose Solution
- ADF
Australian Defense Force
- ARC
Australian Red Cross
- BARDA
Biomedical Advanced Research and Development Authority
- BEST
Biomedical Excellence for Safer Transfusion
- CABG
Coronary artery bypass grafting
- CBER
Center for Biologics Evaluation and Research
- CD41a
Cluster of differentiation 41a (GPIIb/IIIa)
- CD42b
Cluster of differentiation 42b (GPIb)
- cGMP
Current Good Manufacturing Practice
- CLIP
Cryopreserved Platelets Versus Liquid Platelets Trial
- CPP
Cryopreserved platelet product
- DARPA
Defense Advanced Research Projects Agency
- DMSO
Dimethyl sulfoxide
- DoD
Department of Defense
- FDA
Food and Drug Administration
- FFP
Fresh frozen plasma
- GPIb
Glycoprotein Ib
- GPIIb/IIIa
Glycoprotein IIb/IIIa
- ICH
International Council for Harmonisation of Technical Requirements for Pharmaceuticals for Human Use
- LDH
Lactate dehydrogenase
- LSP
Liquid-stored platelets
- LyPt
Lyophilized platelets
- LyPt-P
Lyophilized platelets stabilized with paraformaldehyde
- LyPt-T
Lyophilized platelets stabilized with trehalose
- MTFs
Medical treatment facilities
- NATO
North Atlantic Treaty Organization
- NHP
Non-human primate
- NIH
National Institutes of Health
- NLAF
Netherlands Armed Forces
- NZWR
New Zealand white rabbits
- PAS
Platelet additive solution
- PVC
Polyvinyl chloride
- RT
Room temperature, 20–24 °C
- US
United States
- WB
Whole blood
Early Platelet Product Development and Exploration of Alternative Storage Methods

Platelet product development timeline
In 1956, Drs. E. Klein, P. Arnold, and colleagues reported that, even though platelet counts were not elevated, both cryopreserved and lyophilized platelets were effective in controlling thrombocytopenic bleeding in children with acute leukemia and aplastic anemia [14, 15]. The potential clinical benefit of these products for the management of thrombocytopenia was shown by Stefanini and Kistner in 1958 [16]. Deaths due to hemorrhage in thrombocytopenic patients undergoing chemotherapy was reduced by platelet transfusion. Development efforts focused on improving the in vivo circulation time of platelets to reduce the frequency of transfusions. Murphy and Gardner demonstrated that storage of platelets at room temperature (RT, 20–24 °C) resulted in remarkable improvements in platelet circulation, thereby decreasing the number of transfusions needed to support thrombocytopenic patients [11, 12, 17]. RT-stored platelets thus became the standard of care; however, availability quickly became an issue, and research focused on methods to extended storage time. Plastic bags with improved gas permeability, investigations into platelet storage solutions, and improvements in platelet apheresis collection systems became the primary developmental goals.
Despite technological improvements that improved in vivo circulation, ex vivo storage of platelet components was limited to 3 days with the consequence that availability of a reliable supply of platelets remained a continual challenge. Lyophilized, cryopreserved, and refrigerated platelets had the potential to extend ex vivo storage, but development efforts lagged due to the focus on circulation time and the inability to show hemostatic benefits in some thrombocytopenic animal models [9, 11, 18]. Although it was not known at the time, the disappointing function of these products was likely due to changes in the platelet membranes and other effects of cold activation and, in the case of lyophilization, excessive damage to the structural integrity [19–23]. Effective methods to protect platelets during lyophilization were decades away, but research into potential cryoprotectants began almost immediately [18, 24–26].
Investigators continued in vitro and animal studies to characterize cryopreserved platelets but recognized that a cryoprotectant such as glycerol and/or dimethyl sulfoxide (DMSO) was needed to improve results [18, 24–32]. Djerassi, Roy, and colleagues described a successful method of cryopreserving platelets using glycerol and DMSO [25, 26]. With these advances, studies documented preservation of morphology, viability, organelles, contractile proteins, clot retraction, lactate dehydrogenase (LDH), oxygen consumption, aggregation and release reactions, as well as measurable in vivo survival in human and animal models [33, 34]. Despite the move to RT-stored platelets and the use of directed donor HLA-compatible platelets to support alloimmunized patients, Schiffer and colleagues at the University of Maryland chose to use cryopreserved autologous platelets well in the 1980s [10, 35–40].
Challenges in Providing Room Temperature Platelet Concentrates
Platelet storage at RT was limited to 3 days until 1981, when the US Food and Drug Administration (FDA) issued a guidance allowing an extension to 5 days. In 1984, FDA amended the guidance to allow 7-day storage, but this was rescinded 2 years later due to adverse events related to bacterial contamination [41]. The 5-day storage period was accompanied by the requirement for routine bacterial testing, leaving little time for transport and storage of platelets to remote areas. The short shelf life of 22 °C stored platelets (roughly 3 days following infectious disease testing and processing) results in significant and constant pressures on availability [42, 43]. This in turn presents significant production, distribution, and logistical challenges to blood organizations [43, 44]. To cover urgent needs, hospital users maintain platelet inventory in excess of their daily demand [44]. One undesirable outcome is wastage due to expiration estimated at 7.7–12% in the USA and up to 25% in some centers [43–45].
Supplying room temperature-stored platelet concentrates to combat personnel created even greater challenges for the US military. Maintaining platelet concentrate agitation during storage and transport resulted in an additional logistical burden preventing platelet availability in austere environments. Alternative platelet-containing products like cold-stored group O WB were available and used during the Korean and Vietnam conflicts; however, the only approved WB product for use within the USA was type-specific. As the blood type of acutely bleeding civilian prehospital patients is often unknown, WB fell out of civilian use in the 1980s. Military interest in cryopreservation and lyophilization persisted due to the need for longer storage times to accommodate extended transport times over large distances [32, 46–49].
Cryopreserved Platelet Product (CPP) Characterization and Development
US Military CPP Research and Development Programs
As an alternative to RT platelets, the US Office of Naval Research sponsored a CPP research program at the US Naval Blood Research Laboratory, Boston, MA, in the 1970s. Valeri and colleagues extensively characterized CPP in DMSO and evaluated in vitro and in vivo performance prior to advancing to clinical trials [32, 48, 50, 51]. A variety of parameters were measured including oxygen consumption, aggregation, release reactions, storage duration, ultrastructural alterations, and phagocytic function followed by evaluations of circulation and hemostatic effectiveness in canine, baboon, and other animal models [32, 48, 50, 51]. The Valeri method began with freezing single units of platelets prepared from WB in 5% DMSO at a freezing rate of 1 °C/min to −40 °C, followed by storage in the vapor phase of liquid nitrogen [52]. Frozen platelets were prepared for transfusion by thawing in a 37 °C water bath, washing, and resuspending in plasma. Valeri’s group continued to develop and refine their methods ending with freezing of apheresis collected platelets in 6% DMSO that were frozen and stored at −80 °C. The units were thawed, washed, and resuspended in anticoagulant citrate dextrose (ACD) solution plasma prior to transfusion.
The culmination of this development program was a clinical trial in patients undergoing cardiopulmonary bypass surgery who were randomized to receive either CPP or RT platelets in the perioperative period. The trial was successful in demonstrating that CPP established hemostasis as effectively as control platelets and non-inferiority metric was met [53]. The results were published in 1999, but the cumulative preclinical and clinical data on the Valeri method for platelet freezing were not sufficient to obtain FDA approval. Valeri and colleagues continued development including the pivotal invention of the no-wash method , enabled by removal of the DMSO/plasma supernatant prior to freezing [54].
International CPP Research and Development Programs
The Netherlands Armed Forces (NLAF) also experienced considerable challenges in supplying platelets to austere environments. Having learned from the Valeri laboratory, the NLAF chose to augment standard blood products with CPP and other frozen blood products rather than rely on a walking blood bank for fresh WB and apheresis platelet products. The NLAF began using frozen 6% DMSO platelets reconstituted in thawed plasma with thawed deglycerolized red cells and thawed fresh frozen plasma (FFP) in their deployed medical treatment facilities (MTFs) in 2001 [55]. They published their experience in 2016 after reviewing 4 years of hemovigilance and combat casualty outcomes data collected in Afghanistan beginning in 2006. Results were excellent, with only 1 observed mild transfusion reaction in 3060 transfused products. Twenty-four-hour and hospital stay mortality (14% compared to 44% in historical controls, p = 0.005) decreased significantly in massive transfusion patients. The authors concluded that the use of frozen products, including CPP, in massive transfusion is safe and effective [55].
Rapid dissemination of these findings was likely facilitated during the recent conflicts in Iraq and Afghanistan, because exchange of medical information was common among the North Atlantic Treaty Organization (NATO) coalition forces [56]. In Australia, the University of Queensland, the Australian Red Cross (ARC), the Australian Defense Force (ADF), and others are collaborating to expedite a CPP development program [57–59]. In addition to potential military applications, providing a platelet product with storage times of years, rather than days, could provide hemostatic platelets in smaller rural hospitals that cannot sustain an RT platelet concentrate inventory. One-third of Australia’s population lives in remote areas where providing emergency services and blood products is challenging [60]. The recognition that longer-stored, potentially more hemostatic platelet-derived products are needed for both civilian and military centers mirrors similar viewpoints recently expressed by physicians in the USA and Europe [42, 61].
The ARC has studied CPP using various preparations in platelet starting material. The major differences included the initial storage solution (70% platelet additive solution, or PAS, versus 100% plasma), the final reconstitution fluid (70% PAS versus 100% plasma), and method of collection (apheresis versus buffy coat preparation) [62–66]. ARC reported differences in factor activity, protein content, microparticles, and other biological response modifiers in these CPP compared to standard RT platelets [64]. They noted that fibrinogen was higher in CPP, whereas factors V and VIII activity were decreased. The clinical significance of these findings regarding in vivo CPP function and outcomes was difficult to estimate given that these platelets are primed for activation and in vitro studies are difficult to correlate to clinical findings. The ARC investigators found that thrombin generation and clotting activity were faster, while clot strength was similar to fresh [64–66]. As reported by others, aggregation was attenuated compared to Day 2, but comparison was not made to aggregation after Day 3, when RT platelets typically respond poorly to agonists.
Reade and colleagues recognized the limitations of currently available clinical data for CPP. The University of Queensland, the ARC , and the ADF collaborated on a randomized, controlled multicenter clinical trial in a surgical bleeding population, Cryopreserved Platelets Versus Liquid Platelets (CLIP) Trial (ACTRN12612001261808) [57]. The investigators hypothesize that CPP will be at least as effective and safe as conventional RT platelets in the management of active bleeding related to surgery. The primary endpoints are protocol feasibility in this setting and safety and acceptability [57]. Clinical efficacy as measured by 28-day mortality, blood loss, transfusion requirement, and thromboembolism will be assessed as secondary endpoints. Results are not yet available.
The US Army has sponsored a development program for CPP. Based on the Valeri no-wash method, a Current Good Manufacturing Practice (cGMP) manufacturing process has been developed [67]. Briefly, gamma-irradiated (25Gy) apheresis platelets in plasma from either the Trima apheresis system (Terumo BCT, Inc., Lakewood, CO, USA) or Amicus apheresis system (Fresenius-Kabi, Lake Zurich, IL, USA) are brought to 5.6 to 6.7% effective DMSO concentration by adding 27% sterile DMSO/saline concentrating by centrifugation at 1250× g for 10 min, removal of supernatant to a final volume of 20–35 mL in a freezing bag (Cryostore 500, OriGen Biomedical, Austin, TX, USA), placed into a polyvinyl chloride (PVC) overwrap bag, and stored in a cardboard freezing box. The box is placed flat on the floor of a chest-type mechanical 80 °C freezer. CPP are stable for up to 24 months at ≤ −65 °C. To prepare for transfusion, CPP is thawed in a 37 °C water bath for 8 min. After a 30-min rest period, 0.9% NaCl at 20–24 °C is added slowly in aliquots of 10 mL and 15 mL while gently mixing. The resuspended CPP contains approximately 2.0 × 1011 to 3.6 × 1011 irradiated platelets in a volume of ≥45 mL to ≤60 mL and approximately 1250–2530 mg residual DMSO. Thawed diluted CPP units are stable at RT for up to 4 h.
CPP In Vitro Phenotype
In vitro phenotype for 5-day LSP and CPP
5-day LSP | CPP | |
---|---|---|
Platelet concentration (×103/μl) | 1521 ± 144 | 6295 ± 816 |
Microparticles (% of platelet events) | 6 ± 3 | 42 ± 9 |
Phosphatidylserine exposure (% of platelet events) | 7 ± 4 | 73 ± 4 |
Aggregation (ADP + EPI) (%) | 75 ± 24 | 20 ± 13 |
Aggregation (collagen) (%) | 83 ± 14 | 16 ± 12 |
TGA peak thrombin (nM) (SD) | 72.3 (10.3) | 159.6 (25.7) |

Transmission electron microscopy of LSP and CPP and CPP supernatant reveal the increased activation morphology of CPP. Transmission electron micrograph of (a) LSP at 7000×, (b) CPP at 7000×, and (c) the CPP supernatant following high centrifugation to visualize microparticles at 19,500×. Bar = 2 μm in a and b and 500 nm in c. (From Dumont et al. [67], with permission of John Wiley and Sons)
Transfused platelet recovery and survival
Platelet recovery (%) | Platelet survival (d) | |
---|---|---|
Fresh | 63 ± 9 | 8.6 ± 1.1 |
CPP | 33 ± 10 | 7.5 ± 1.2 |
P | <.0001 | <.0001 |
% of fresh | 52 ± 12 | 89 ± 15 |

Micrographs of platelet adhesion under flow conditions (Baumgartner model). Microscopic fields of perfusion experiments for platelet-depleted whole blood substituted with 200 × 109 platelets/L of 5-day LSP (a) or CPP (b) reveals CPP with decreased platelet coverage compared to LSP under shear (22 ml at 600/s for 10 min). (From Cid et al. [68], with permission of John Wiley and Sons)
CPP Recovery and Survival
Autologous recovery and survival of CPP in healthy subjects have been evaluated compared to fresh autologous platelets transfused simultaneously following the method recommended by the Biomedical Excellence for Safer Transfusions (BEST) Collaborative, with some modifications for CPP [67, 69, 70]. As expected with an activated platelet product, CPP recovery was 52% of fresh platelets (Table 9.2); however, they circulated for 7.5 days or 89% of fresh platelets [70]. This is consistent with good clinical outcomes previously reported when similar CPP products were used for hypoproliferative thrombocytopenia as discussed above. CPP recoveries are lower and could result in the need for increased transfusions, but it is also possible that CPP may allow physicians to manage patients with a lower platelet count due to the improved hemostatic potential of this product. Further studies are needed to evaluate CPP safety and clinical utility, and these are currently in progress.
CPP Phase 1 Dose Escalation

CPP dose escalation. Platelet count increments (left axis) and corrected count increment (right axis) for Phase 1 CPP dose escalation trial. (From Slichter et al. [71], with permission of John Wiley and Sons)
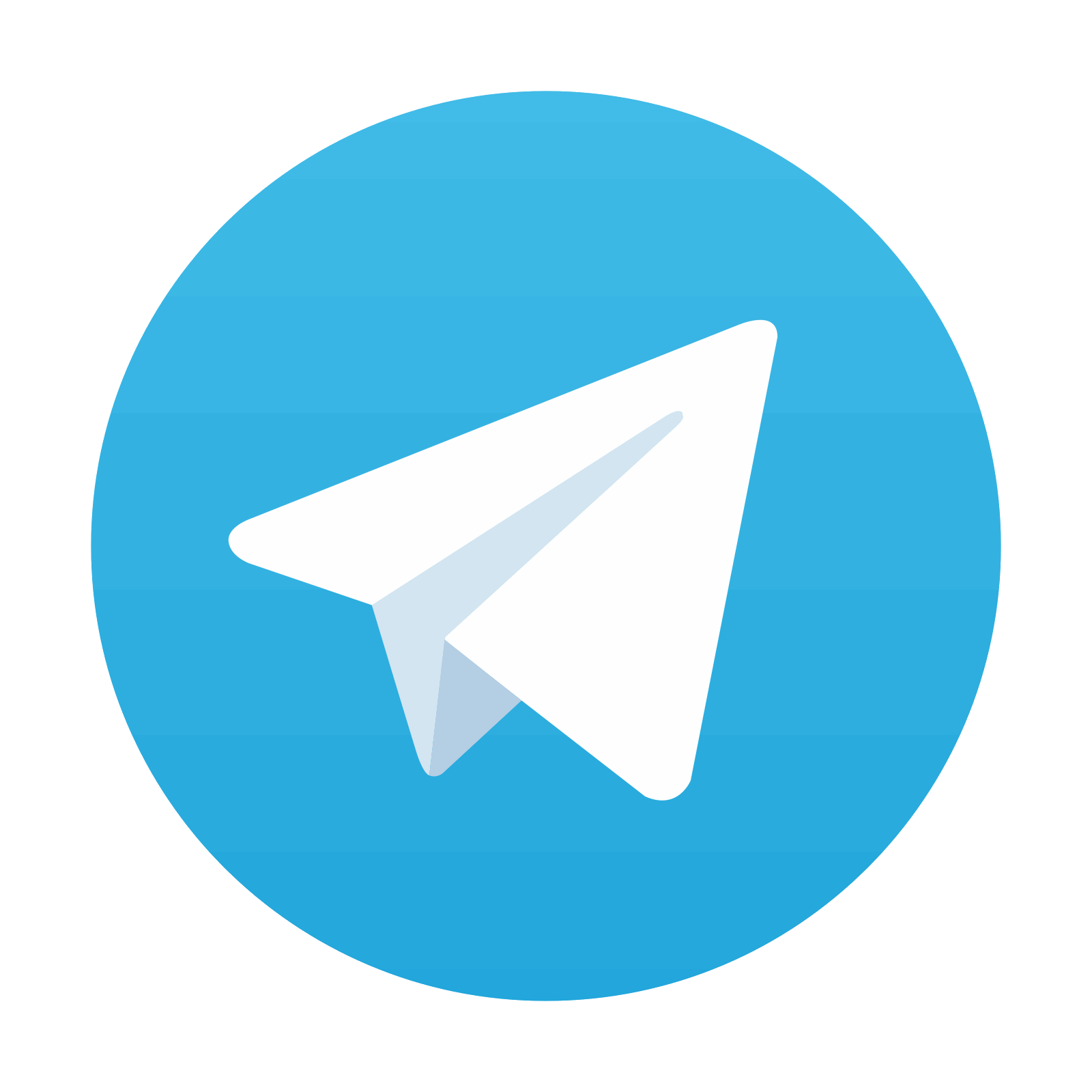
Stay updated, free articles. Join our Telegram channel

Full access? Get Clinical Tree
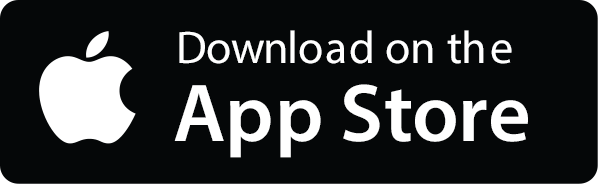
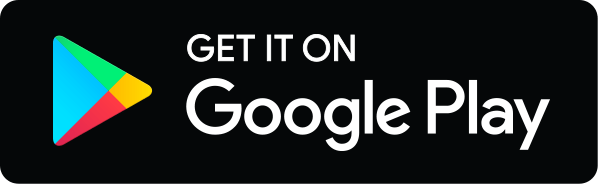