21 Dan Miulli The body is composed of 50 to 70% water and requires euvolemia to survive optimally and to overcome illness. The understanding of sodium and water balance is key to managing the patient in the neurosurgical intensive care unit (NICU). Although water content is different in individuals, in general, men have a higher percentage of body fluid, and in either sex, the amount decreases with age. Fifty-five to 75% of the body’s total fluid is intracellular, and 25 to 45% is extracellular. The extracellular fluid is located in intravascular plasma (25%) and interstitial spaces (75%). Therefore, in a 70 kg man, of the 48 L of total body fluid, 8/12 is intracellular (32 L), 3/12 is interstitial (12 L), and 1/12 is intravascular (4 L). Fluid balance is extremely complicated, governed by antidiuretic hormone (ADH) (also known as arginine vasopressin [AVP]), aldosterone, and the natriuretic peptides: brain (BNP), atrial (ANP), and C-type (CNP). The main control of fluid balance is the tonicity made mostly by sodium, with the minor constituents chloride and bicarbonate ions of the extracellular fluid adding to the remainder. Potassium, magnesium, and phosphate constitute intracellular ions and tonicity. The hormones mentioned react to as little as a 1 to 2% change in vascular tonicity. ADH/AVP is made in the magnocellular part of the supraoptic nuclei of the hypothalamus and conveyed to the posterior pituitary, where it is secreted. It binds to the distal renal collecting tubules to stimulate water reabsorption and to make concentrated urine in response to opioids, barbiturates, and car-bamazepine, as well as high osmolality, hypovolemia, stress, hypoglycemia, and pain. ADH/AVP is a potent vasoconstrictor. In addition to affecting renal tubule cells, it affects brain cells. ADH/AVP V receptors appear to control fluid entry into the brain cell by activation of aquaporin-2, allowing fluid into the cell, which, if increased excessively, increases brain edema and infarction.1 Aldosterone is released in response to baroreceptors sensing hemorrhage, decreased intravascular volume, or decreased blood pressure. The baroreceptors stimulate rennin angiotensin, leading to aldosterone release and causing sodium resorption followed by water retention. Natriuretic peptides not only cause renal sodium loss and fluid loss, but they also reduce water and sodium in areas of brain edema by directly reducing brain cell water and brain capillary permeability.2,3 ANP increases cerebral blood flow and causes significant cerebral vasodilation. It is also produced in the hypothalamus and is found in the median eminence, midbrain, choroid plexus, and spinal cord.4 BNP fibers are found along the carotid, middle cerebral, posterior communicating, and anterior cerebral arteries (Table 21–1).5 Normally, a person is able to drink and will become thirsty when the osmoreceptors in the anterior hypothalamus become stimulated ~295 mOsm/kg. However, when the patient is comatose from stroke, trauma, tumor, infection, or other devastating neurologic disease, the physician must prescribe appropriate fluids. The average dose of IVF can be approximated several ways using a general or graduated, weight-based formula (Table 21–2). There are several physiological circumstances that require increased fluids above the normal amount (Table 21–3).4
Fluid Management
Need for Intravenous Fluids
Hormone Control of Fluids
Hormone | Fluid action | Vessel action |
ADH/AVP | Water reabsorption increased | Vasoconstriction |
Aldosterone | Sodium reabsorption increased | Vasoconstriction |
Natriuretic peptide | Renal sodium loss | Vasodilation |
ANP, BNP, CNP | Renal and brain water loss |
ADH, antidiuretic hormone; ANP, atrial natriuretic peptide; AVP, arginine vasopressin; BNP, brain natriuretic peptide; CNP, C-type natriuretic peptide.
Daily Fluid Requirements* 100 mL/kg for the first 10 kg in 24 hours, then 50 mL/kg for the next 10 kg in 24 hours, then 20 mL/kg for remainder weight in kg in 24 hours age <60 years 15 mL/kg for remainder weight in kg in 24 hours age >60 years * For 70 kg male, age 55: (1000 + 500 + 1000)/24 = 104 cc/hour Hourly Fluid Requirements* 4 cc/hour for the first 10 kg 2 cc/hour for the next 10 kg 1 cc/hour for remainder weight in kg * For 70 kg male, age 55: 110 cc/hour Daily Fluid Requirements, Average* Average 35 cc/kg, elderly 30 cc/kg * For 70 kg male, age 55: 2450/24 = 102 cc/hour |
If these conditions are not taken into consideration, a patient will become hypovolemic. Volume status can be approximated using the following clinical criteria:
- Heart rate: >110 beats/minute
- Systolic blood pressure (SBP): <90
- Dry mucosal surface
- Crackling heard in lungs
- Evidence of skin edema or turgor
- Central venous pressure (CVP): <6 to 8 mm Hg
- Urine specific gravity: <1.010 or >1.030
- Urine color: clear or amber
- Urine output: <0.5 cc/kg/hour
- Daily weight change: increase or decrease of 1 kg
- Input and output, 8 hours: <500 cc (24 hours: <1500 cc)
Fever: 12.5% for each 1°C above normal Sweating: 10 to 25% Hyperventilation: 10 to 60% |
Osmolality, Osmolarity, and Tonicity
Just knowing the fluid balance of the patient or even the cell will not allow proper maintenance. The amount of solutes concentrated in fluid is also vital. Osmolality is a measure of the number of osmotically active particles measured in osmoles or milliosmoles per kilogram of solvent (mmol/kg or mOsm/L). A highly concentrated osmotic solution will have a high osmolality. That is, it will have more osmotically active particles per unit of solvent than a solution with a low osmolality.
Osmolarity is the total quantity (concentration) of dissolved substances in a solution both penetrating and not penetrating the semipermeable membrane.
Tonicity reflects only the concentration of nonpenetrating solutes in the extracellular space compared with the nonpenetrating solute concentration inside the cell.
The intracellular space is a large reservoir, accounting for 8/12, or 32 L, of fluid, and can easily control the solutes in the extracellular volume of 3/12, or 12 L An iso-osmotic cell has an osmolarity of 300 mOsm; that is, it contains 300 mOsm of penetrating and nonpenetrating solutes. When the extracellular fluid also contains 300 mOsm of penetrating and nonpenetrating solutes, the extracellular solution is isotonic, and the cell will not change fluid volume. When the extracellular solution contains 250 mOsm (equivalent to lactated Ringer’s solution) of penetrating and nonpenetrating solutes, the extracellular fluid is hypotonic, and the cell will swell as the fluid rushes into the large cellular reservoir in an attempt to make the extracellular space isotonic. If the extracellular solution contains 921 mOsm concentration (equivalent to 3% saline) of penetrating and nonpenetrating solutes, the extracellular solution is hypertonic, and the cell will shrink; fluid will rush out in an attempt to make the extracellular space isotonic.
In reality, the cell contains different amounts of penetrating and nonpenetrating solutes compared with the interstitial and intravascular fluid. A cell may have 300 mOsm of nonpenetrating solutes and 20 mOsm of penetrating solute such as urea, causing it to be hyperosmotic (320 mOsm). If the extracellular fluid has 300 mOsm of nonpenetrating solutes, the extracellular solution is isotonic, and the cell will not change fluid volume. The tonicity is always the relationship of the nonpenetrating solutes across the cellular membrane. Even though the cell’s osmolarity is 320 mOsm (300 mOsm nonpenetrating and 20 mOsm penetrating solutes), the extracellular solution is isotonic because it has the same concentration of nonpenetrating solutes as the cell. There are multiple osmotically active components, or osmoles, of solute concentrated in body fluid, expressed as mOsm/L. The intracellular penetrating solute concentration, such as urea and ethanol, does not affect fluid movement because the penetrating solutes will equilibrate across the semipermeable membrane.6
Component | Intracellular fluid (mOsm/L) | Extracellular fluid (mOsm/L) |
Sodium | 5–15 | 135–145 |
Potassium | 140–150 | 3.5–5.0 |
The nonpenetrating particles or solutes making up the tonicity of the intravascular fluid are inorganic ions of sodium, chloride, bicarbonate, and potassium, as well as organic osmolytes of amino acids, sorbitol, and methy-lamines, among others. These osmoles do not easily cross the cell membrane, are restricted to the extracellular or intracellular compartments by the semipermeable cellular membrane, and therefore drive the fluid shifts or the tonicity of the fluid. Compounds such as ethanol, urea, and other solutes easily cross the intravascular boundary and therefore do not significantly add to the tonicity of the fluid, although they do add to the osmolarity.
Sodium is the major component of intravascular fluid whose regulation is closely tied to the volume of the same intravascular fluid. It is the main determinate of tonicity or effective osmolality, which determines where fluid will move. The normal plasma osmolality is 280 to 290 mOsm/L (although some sources suggest as low as 275 to as high as 295 mOsm/L) and is closely controlled by thirst osmoreceptors in the anterolateral hypothalamus. Intracellular components consist of potassium and other tightly regulated anions, leading to an osmolality of 300 mOsm/L, driving fluid into the cell. Tonicity affects fluid movement. The normal concentrations of intra- and extracellular components in osmoles are given in Table 21–4.
From the average extracellular concentrations, the daily requirements of those same constituents can be deduced (Table 21–5).
The Blood–Brain Barrier and Intravenous Fluids
The blood–brain barrier has an effective pore size of 8 angstroms (Å) and is impermeable to sodium, ions, water-soluble compounds, and protein, but is permeable to lipophilic substances and gases. The majority of substances are actively transported across the brain capillary endothelial cell to the brain interstitial space. The cerebrospinal fluid (CSF) flows along a gradient to the ventricle and is absorbed through the arachnoid villi, where a pressure differential allows it to pass into the venous sinus. Likewise, the larger reservoir, the cellular fluid, is actively transported into the brain interstitial space and follows the same pathway. Ninety-one percent of the circulating fluid is in the intracellular and interstitial spaces; this is the area where fluid and solute need to be regulated.
The optimal treatment would be to actively remove solutes out of the cell into the brain interstitial space, through the ventricles, and into the blood. This would be accomplished with high-tonicity, low-osmolality fluid flowing from the brain capillary endothelial cell into the interstitium, increasing the tonicity of the interstitial fluid with nonpermeable sodium. Low molecular weight solutes would then leave the damaged swollen brain cell, followed by water. The damaged cell contains increased osmoles of H+ and K+. Therefore, if ion exchange mechanisms are functioning, lower interstitial solutes of H+, K+, and others would simultaneously draw the cellular toxins out of the cell into the CSF, followed by water, then drained out of the ventricle into the venous system; simulating CSF ventricular dialysis.
Inhibiting CSF formation or decreasing intravascular volume does not decrease intracellular and interstitial edema. Decreasing edema is accomplished by removing CSF.
During times of trauma and disease, the cell may be swollen in an initial response to dilute the solute toxins. There is additional swelling when fluid increases in the brain interstitial space in an attempt to dilute those increased solutes that entered through the broken blood–brain barrier. If the interstitial fluid does not drain out of the ventricle, the brain will swell, compressing blood vessels and leading to further ischemia.
The brain initially attempts dialysis by opening the blood–brain barrier, allowing water and solutes to enter the brain interstitium attempting to have a hypo-osmolar, hypertonic solution, so that solutes may leave the cell, followed by the increased cellular water.
The blood–brain barrier, which is formed by the cerebral capillary endothelial cells, contains channels regulated by aquaporins through which water flows somewhat passively down an osmotic gradient. The cerebral capillary endothelial cells are different in function and regulation from those of the brain arteriole or venule. The other brain fluid barrier, albeit much smaller in area, is between the blood and CSF and consists of the choroid plexus epithelium. Tight gap junctions, low pinocytic activity, and specific energy-dependent membrane transporters regulate the passage of substances into and through the capillary endothelial cells to the brain interstitial fluid. Substances can freely diffuse into the capillary endothelial cells if they are gases, lipid soluble, and have a molecular weight less than 400 to 600 daltons (Da). However, most substances, such as choline, glucose, glutamate, and lactate, are associated with either a carrier-mediated transport system or solutes such as cationized albumin, insulin, insulin-like growth factor (IGF), and transferrin. Others are associated with a receptor-mediated transport system. Both systems transport substances through the capillary endothelial cell into the brain interstitial space.
The intact blood–brain barrier only mildly restricts the passive diffusion of water from a cellular hypotonic solution to an interstitial hypertonic space through aquaporin-regulated channels. In the adult, the blood–brain barrier regulates most common IVF types. However, the brain damaged by trauma, ischemia, tumors, and increased pressure does not have an intact blood–brain barrier, nor is the barrier intact with changes in normal brain homeostasis that occur with edema, hyperventilation, mannitol infusion, hyperthermia, hypotension, hypertension, or tumor necrosis factor secretion. During these times of stress, when the blood–brain barrier is open, vasogenic edema has begun, and the brain is at further risk of damage if the incorrect therapeutic fluids are used. The damaged brain does not benefit from dehydration hypovolemia but from a euvolemic to slightly hypertonic state.7,8 In addition to vasogenic edema from an opened blood–brain barrier, the more common cellular cytotoxic edema forms in an effort to combat further injury, diluting the cellular toxins accumulated because of decreased blood and plasma flow (e.g., hydrogen ions and potassium). Therefore, dehydration hypovolemia becomes deleterious for combating cytotoxic edema. Euvolemia must be maintained to flush the toxins from the cell. The cell must maintain an osmotic gradient in the brain interstitial space, which allows the transport of solutes and water out of the cell.
Since 1919, the deleterious effects of hypotonic solution have been known.9 Decreasing the IVF 1 mEq/L will decrease the osmotic pressure 38.6 mm Hg; likewise, decreasing the IVF 5 mEq/L (10 mOsm/kg) will decrease the osmotic pressure 193 mm Hg. This change in fluid drive is minimal under normal homeostasis but will aggravate the swelling and increase the intracranial pressure (ICP) in an opened blood–brain barrier, a condition that occurs with tumors, ischemia, and trauma. The best fluid approximates or is slightly above normal sodium concentration and normal tonicity without having an additional glucose load. The calculated mOsm/L of individual fluids is shown in Table 21–6; however, it should be noted that the actual measured osmolality is ~20 mOsm/kg water less.10 Administering hypertonic saline decreases intracellular volume from 32.0 to 30.6 L, increases interstitial volume from 12.0 to 13.2 L, and increases intravascular volume from 4.0 to 4.4 L Normal saline has no significant change on intracellular volume, increases interstitial volume from 12.00 to 14.25 L, and increases intravascular volume from 4.00 to 4.75 L D5W increases intracellular volume from 32 to 34 L, increases interstitial volume from 12.00 to 12.75 L, and increases intravascular volume from 4.00 to 4.25 L11 Therefore, normal saline should be used for resuscitation and any time there is brain damage or the potential for brain damage. D5W, half-normal saline, and lactated Ringer’s solution should be avoided. Hypertonic saline must be considered during times of increasing cerebral edema (Table 21–6).
Prescribing additives to IVFs must be done with care. Hyperglycemia at the time of primary or secondary injury aggravates ischemic insults and worsens neurologic outcome by increasing lactic acidosis. The condition is not from previously unknown or untreated hyperglycemia; rather, it is usually from reaction to trauma, stress, glucocorticoid administration, sepsis, or other causes.
Hyperglycemia causes a dose-dependent increase in the severity of ischemic and severe head injury. In one study, glucose >150 mg/dL had worse outcome than glucose < 150 mg/dL12 Hyperglycemia-induced lactic acidosis increases neurologic damage, increases infarct size, and causes secondary injury, a condition that appears to be a major cause of decreased outcome in patients with severe head injury.13,14
Therefore, under conditions of suspected brain injury, as seen with ischemia, tumors, and trauma, do not give IV solutions that would worsen edema, such as those containing dextrose, low tonicity, and low sodium.15 Start fluid maintenance with IV normal saline to keep the patient euvolemic to slightly hypervolemic.
Hypovolemia
Hypotension must be avoided at all costs, because a single episode of hypotension doubles the morbidity and mortality associated with severe head injury. Hypovolemia decreases cardiac output and nervous tissue perfusion. It may be seen with a blood urea nitrogen/creatinine (BUN/Cr) ratio greater than 15/20:1 or any other number of clinical conditions. Also, it does not improve outcome after brain injury.
The body attempts to correct hypovolemia by upregulation of norepinephrine, ADH, rennin-angiotensin II, and aldosterone, each increasing sodium reabsorption and water retention. Sodium absorption is increased in the renal proximal tubule cells by rennin-angiotensin II, and reabsorption of sodium occurs at the distal tubule and collecting duct by the stimulation of aldosterone. Norepinephrine decreases glomerular filtration rate-enhancing sodium reabsorption at the proximal tubule. However, these hormones also affect brain cell water absorption in a similar fashion, further increasing water retention in the brain interstitium and cell. Therefore, not only trauma patients but also patients with cerebral ischemia, vasospasms, and spinal cord injuries require fluid resuscitation. Euvolemia to slight hypervolemia improves outcomes in head trauma. Each effect of hypotension further worsens the effects of trauma. Daily maintenance fluids should be administered to compensate for the normal loss, for example, in a 70 kg man of 1200 to 1500 mL/day and to maintain a urine output of 0.5 to 1.0 cc/kg/hour. If there are questions concerning volume status that cannot be determined using clinical and laboratory data presented in this chapter, then a Swan-Ganz catheter should be inserted to attempt to obtain normal16,17 or optimal values in the management of head injury (Table 21–7).18
Patients with spinal cord injury have decreased sympathetic tone, leading to vasodilation, bradycardia, hypotension, and decreased perfusion. These patients must have adequate fluid resuscitation with euvolemia and vasopressors to restore sympathetic tone. They should not be overhydrated, which would cause hyponatremia, pulmonary edema, and worsening of spinal cord edema.
Normal values (mm Hg) | Optimal values (mm Hg) | |
CVP | 0–8 | 6–8 |
PAD | 6–16 | 12–16 |
PCWP | 6–14 | 10–14 |
CVP, central venous pressure; PAD, pulmonary artery diastolic pressure; PCWP, pulmonary capillary wedge pressure.
Intravenous Fluids in Extremes of Pathological Conditions
When intensive care management of the neurologically injured patient begins to fail with standard fluids and treatment, additional or different IVFs may be required. When ICP or edema remains elevated, despite initial measures, mannitol or hypertonic saline may be needed.
Hypertonic solutions have been shown to lower ICP19–21 and improve cerebral perfusion pressure (CPP),22–25 whereas isotonic fluids do not improve ICP, as can be deduced from changes in intracellular, interstitial, and intravascular volumes, as noted above.26 In a study by Vasser et al,27 hypertonic saline reduced ICP in severe head injured (SHI) patients. Furthermore, 7.5% sodium chloride (NaCl) improved survival when compared with standard treatment in patients with a GCS of 3 to 8. Wade et al28 performed a meta-analysis of SHI patients who receive hypertonic saline and demonstrated that they are twice as likely to survive as those who receive standard therapy. Hypertonic saline is more beneficial than mannitol in refractory intracranial hypertension29,30 by creating a driving force that pulls water out of the brain cells and interstitium. Hypertonic saline has an increased concentration of sodium and chloride. In an attempt to decrease hyperchloremic metabolic acidosis, the hypertonic solution may be made with a balanced mixture of sodium chloride and sodium acetate.
There is considerable debate regarding resuscitation with colloids. However, if a 1 to 2 L fluid bolus of normal saline does not stabilize the patient or 20 cc/kg in children per advanced trauma life support (ATLS) guidelines, then colloid should be given. Crystalloids such as normal saline remain intravascular for a maximum of 2 hours, and their effect on pulmonary wedge pressure and cardiac index is small. Colloids contain high molecular weight solutes that do not readily cross the capillary wall, remain intravascular for longer periods of time, and require smaller infused volumes. Some investigators have demonstrated that colloids exacerbate pulmonary edema, decrease cardiac output, and compromise the immune system. Others believe that colloids reduce secondary brain injury. Of the colloids, blood may be the best alternative for fluid resuscitation, attempting to maintain a hemoglobin and hematocrit of 10 and 33%, respectively.31–36 This allows maximum oxygen-carrying capacity and best viscosity.37–40 Hemodilution to a hemoglobin and hematocrit of 10 and 33%, respectively, has been studied in the management of cerebral vasospasms, in an attempt to improve viscosity and oxygen-carrying capacity as well as cerebral blood flow.41,42 Hematocrit lower than 30% decreases oxygen-carrying capacity beyond the improvements made because of increased viscosity. Even in ischemic stroke patients, a hematocrit of 30% optimizes infarct volume, being worse for values ≥35% and <26%.43,44 Anemia, where the hematocrit is less than 25 to 30%, increases cerebral vasodilation, ICP, and edema.45
Several substances in addition to blood have been used to increase volume, such as albumin, Plasmanate (Bayer Pharmaceuticals, Yakutin, Japan), Normosol (Abbott Laboratories, Abbott Park, IL), and hetastarch. Each volume expander has risks; however, the best expander remains blood. Plasminate appears to be better than lactated Ringer’s solution and causes fewer lung problems when compared with other fluids.46,47 Normosol may have better red blood cell (RBC) protection than Plasminate.48 Hetastarch, although it expands volume well, does have the side effect of bleeding, significantly elevating partial thromboplastin time. It therefore should not be used when hemorrhage has occurred or may occur.49 Albumin 5, 20, or 25% may50,51 or may not improve outcome when used in the trauma patient and may in fact increase pulmonary extravascular fluid.52 Although 5% albumin is oncotically and osmotically equivalent to plasma, its use remains controversial. The Saline Versus Albumin Fluid Evaluation (SAFE) study53 concluded that, in patients in the NICU, use of 4% albumin or normal saline for fluid resuscitation results in similar outcomes at 28 days. The authors looked at 6997 randomized patients. For the entire study population, there were no significant differences in deaths and number of days spent in the NICU, hospital, on ventilator, or in renal replacement therapy. However, the subgroup analysis of patients with trauma and brain injury trended toward an increased relative risk of death. There was insufficient power to make significant claims. The actual number of patients (59 of 241) receiving albumin with trauma and head injury compared with those receiving saline (38 of 251) was small (p = .009). In the analysis of death in all patients with trauma, 81 of 596 were in the albumin group, and 59 of 590 deaths were in the saline group (p = .06); however, once again, the power was too low to support significant claims. Patients with head injury constituted only 7% of the study population. The investigators concluded that there needs to be further study of trauma and head injury patients.
Mannitol has been in the neuroscience armamentarium for some time. Mannitol improves viscosity and therefore circulation. It does, however, open the blood–brain barrier and, with multiple doses, will cross into the interstitial space and increase its osmotic pull of fluids into the tissue, causing hypotonicity, contrary to the intended desire of therapy. It works on the microvasculature in several minutes, vasoconstricting, decreasing viscosity, increasing cerebral blood flow,54–59 and acting as a free radical scavenger. Mannitol works best at high ICP, low CPP at vasodilation when autoregulation is preserved. Its plasma expansion is equal to 7.5% NaCl. The systemic capillary is permeable to water, which moves into the intravascular space to dilute mannitol, while an intact brain is not freely permeable to water to the same extent as the systemic capillary or the damaged blood–brain barrier. As an osmotic diuretic, mannitol draws in systemic water, causing hemodi-lution and improving shear rate and RBC deformity. The osmotic effect is delayed 15 to 30 minutes, and persists 90 minutes to 6 hours. Mannitol has a small osmotic effect in the brain, only changing the brain water content 2 to 6%. It becomes less effective after multiple doses, such as >3 or 4 doses in 24 hours. If given at higher rates, mannitol moves across the defective blood–brain barrier into tissue, exacerbating edema.60,61 Mannitol can be used when there is suspected increased ICP, such as in the emergency room.
Guidelines for the Management of Severe Head Injury62 states that
[m]annitol is effective therapy for the control of increased ICP. Intermittent bolus is probably better than continuous infusion therapy. Dose ranges are 0.25–1 gram/kg.
OPTION: Where mannitol is being used prior to ICP monitoring, where it is not titrated, you should have evidence of raised ICP present (e.g., signs of transtentorial herniation or progressive neurologic deterioration). Serum osmolarity should be kept <320 mOsm, because when greater the incidence of renal failure is increased. Euvolemia should be maintained and a Foley catheter inserted.
Diuretics
Mannitol may be the most appropriate agent for diuresis in the short term. Another agent is furosemide, which acts by inhibiting distal tubule reab-sorption of sodium and water. It can also reduce ICP and CSF production. The dose varies with the degree of diuresis required, from as little as 5 mg IV every 4 to 6 hours to as high as 80 to 160 mg every 6 hours.
Acetazolamide is a carbonic anhydrase inhibitor reducing CSF production from the choroid plexus while vasodilating. It can be used during conditions of excess CSF production, which by itself causes an increased ICP. It should not be used during times of intracellular and interstitial edema. The usual dose is 125 to 250 mg tid/qid to a maximum of 2 g/day. Side effects include metabolic acidosis that should be checked with chemistry panel or arterial blood gases (ABG). Other side effects include paresthesias, altered taste, renal calculi, and nausea.
Calculation of Fluid Balance
Fluid balance determination may be a difficult task in the NICU because of medications and concomitant injuries. It is important to determine fluid input and output exactly. The patient must be weighed daily using the same scale and the same clutter of material, such as sheets, blankets, pillows, sequential compression devices, and monitors. Although bothersome to the nursing staff, the most exact measurement would be to weigh without these items.
There is no concrete means to determine fluid balance. Swan-Ganz measurement may be confounded by cardiac and pulmonary changes. However, on a daily basis, the fluid balance can be determined from clinical exam and laboratory tests. The clinical exam for volume status must be part of the daily examination of the NICU patient.64–66
Although Table 21–8 simplifies the calculation of fluid balance, the true determination is not simple.
Specific States of Fluid and Electrolyte Imbalance
Specific states of fluid and electrolyte imbalance can be calculated using the following equation:
Osmolality (mOsm/L) = 2 ×(Na mEq/L + K mEq/L) + (BUN/2.8)
+ (glucose/18).
There are three states of hyponatremia (Na < 135 mmol/L), the most common being hypotonic hyponatremia and the least common being isotonic and hypertonic (Table 21–9).
Isotonic hyponatremia (serum mOsm 280–290) is caused by hyperlipi-demia and hyperproteinemia that increase plasma volume and dilute sodium. The expected amount of sodium reduction is equal to the current protein concentration minus 8 g/dL multiplied by 0.25. A very large protein concentration is needed to change sodium concentration significantly. Treatment consists of correcting the underlying disorder.
Hypertonic hyponatremia (serum mOsm > 290) is more commonly seen with diseases of hypertonicity, such as hyperglycemia, or after hypertonic administration of glucose, mannitol, or glycine, causing dilution of sodium. The expected amount of sodium reduction is equal to 1.45 mmol/L for each 100 mg/dL increase in blood glucose above 200 mg/dL Treatment consists of correcting the underlying disorder.
Hypotonic hyponatremia (serum mOsm < 280) is the most common type seen in the NICU patient and itself consists of three subtypes: isovolemic, hypovolemic, and hypervolemic.
Isovolemic hypotonic hyponatremia occurs after consumption of large amounts of water in patients with mild renal impairment, potassium loss from diuretics, or gastrointestinal (GI) loss, as well as in those with tuberculosis, bronchogenic tumors, cirrhosis, aspergillosis, stress, severe pain, acute intermittent porphyria, and those receiving positive pressure ventilation. It is also associated with the use of carbamazepine, phenothi-azines, antidepressants, chloropropramide, oxytocin, thiazide diuretics, sulfonylureas, and opiates, and with syndrome of inappropriate antidiuretic hormone (SIADH). (Information regarding the diagnosis, symptoms, and treatment of SIADH is given below.) Increasing intrathoracic pressure causes aortic baroreceptors to sense hypotension and respond with water retention. Most causes are treated with fluid restriction of 800 to 1500 mL/day. Lower sodium levels can be treated with sodium tablets (2 g) 3 or 4 times per day or 3% NaCl IV solution. The use of each depends on the clinical condition.
Component (absolute value or daily change) | ![]() | ![]() |
Hgb (12–16) | < 10 or L1.5 or > | > 15 or G1.5 or > |
HCT (37–54) | < 30 or L4 or > | > 50 or G4 or > |
Na (134–145) | < 134 or L5 or > | > 145 or G5 or > |
BUN (7–18) or (only give 1 or 2 pts) | < 10 or L4 OR | > 18 or G4 or > |
BUN (7–18) | < 5 or L7 (2 pts) | |
Creatinine (0.7–1.3) | < 0.7 or L0.3 or > | > 1.3 or G0.3 or > |
Serum osmolarity (280–300) | < 280 or L15 or > | > 300 or G15 or > |
Heart rate | > 110 or G30 or > | |
SBP | < 90 or L35 or > | |
Orthostatics | Positive | |
Mucosa | Mucosa dry | |
Lungs | Crackles | |
Skin | Edema | Turgor (2 pts) |
CVP (0–8 mm Hg) (* ![]() | > 9 or G5 or > | < 4 or L5 or > |
Urine specific gravity (1.010–1.030) | < 1.010 or L0.020 or> | > 1.030 or G0.020 or > |
Urine color | Clear | Amber |
Urine output | < 0.5 cc/kg/hr (2 pts) | |
Weight change daily | Gain > 2.2 lb | Lose > 2.2 lb |
I&Os 8 hours | +500 | −500 |
I&Os 24 hours or | +1000 or | −1000 or |
(only give 1 or 2 pts) | +1500 or > (2 pts) | −1500 or > (2 pts) |
Current hemodynamic parameters | ||
Cardiac output 4–7 | Cardiac index 2.8–4.2 | MAP |
PCWP 6–14* 10–14V | PAD 6–16* 12-16 ![]() | SVR 770–1500 |
Note: Of a total of +17 points for positive overload, overload occurs at greater than 5 points. Of a total of −22 points for negative dry, dry occurs at less than −5 points. To determine fluid status, add positive values in column 2 and negative values in column 3. |
Optimal value for the treatment of head injury.18
BUN, blood urea nitrogen; CVP, central venous pressure; HCT, hematocrit; Hgb, hemoglobin; I&Os, inputs and outputs; Na, sodium; SBP, systolic blood pressure.
2. Assuming no significant effects of vasoconstrictors or severe cardiopulmonary changes, the values of pulmonary artery diastolic pressure (PAD) below may be used to adjust fluids. Numbers preceded by + or − indicate change from previous reading.
If PAD > 17or > +5, decrease fluids 25%. If PAD < 10 or < −5, increase fluids 50%.
If PAD > 20 or > +8, decrease fluids 50%. If PAD < 8 or < −8, increase fluids 100%.
If PAD > 23 or > +10, give diuretic, and decrease fluid 75%.
If PAD < 6 or < −10, increase fluids 100%, and give fluid bolus 300% over 1 hour; if no response, give 100% bolus colloid.
Hypervolemic hypotonic hyponatremia is the subtype of edema seen in congestive heart failure, cirrhosis, and transurethral prostatic resection (TURP). Most causes are treated with fluid restriction of 800 to 1500 mL/day and can be augmented with loop diuretic because of the higher afterload. Lower sodium levels can be treated with sodium tablets 2 g 3 or 4 times per day or 3% NaCl IV solution. The use of each depends on the clinical condition.
Hypovolemic hypotonic hyponatremia occurs when loss of fluids rich in sodium, such as bile, sweat, and fluids found in the pancreas, small intestine, and lung, are replaced with D5W or 0.45% NaCl. The most common clinical condition of this subtype seen in the NICU patient is the syndrome of cerebral salt wasting (CSW). (Information regarding the diagnosis, symptoms, and treatment of CSW is given below.) Renal loss of sodium and low volume in CSW are opposite the condition of SIADH. CSW is seen in subarachnoid hemorrhage (SAH) of any type and is possibly due to the brain releasing natriuretic factor from the hypothalamus in an attempt to increase cerebral blood flow and cause cerebral vasodilation during times of vasospasm. Natriuretic peptides not only cause renal sodium loss and fluid loss, but they also reduce water and sodium in areas of brain edema. Natriuretic peptide fibers are found along the carotid, middle cerebral, posterior communicating, and anterior cerebral arteries, areas of highest vasospasm. CSW occurs 3 to 7 days after initial SAH, concomitant with a time of vasospasm occurrence.
Diagnosis of SIADH
The diagnosis of SIADH includes the following parameters:
- Normal or elevated intravascular volume
- Serum sodium < 134 mEq/L
- Low serum osmolality (<280 mOsm/L)
- High urine osmolality, high or normal urinary sodium (>18 mEq/L)
- Normal renal, adrenal, and thyroid function
Hyponatremia in SIADH occurs due to bronchogenic tumors, meningitis, trauma, increased ICP, tumors, and craniotomy.
Symptoms of SIADH
Symptoms of SIADH include headache, confusion, lethargy, nausea/vomiting, muscle cramps, depressed deep tendon reflexes, and seizures; these can lead to coma and death.
Treatment of SIADH
Treatment of SIADH involves slowly elevating the sodium level and restricting fluids to an amount less than urine output. In the adult, fluid intake should be restricted to 800 to 1500 mL/day. If severe SIADH is present, use 3% NaCl and furosemide. (The furosemide causes excretion of dilute urine.) If there is chronic SIADH, as seen in some alcoholics, then elevate the sodium level slowly, 8 to 10 mEq/L per 24 hours, to prevent central myelinolysis of the pons or other areas. In a chronic alcoholic patient, stop 3% NaCl when the serum sodium level reaches 125 mEq/L to prevent a relative hyperosmolar condition in this specific type of patient. The rebound relative hyperosmolar state may be the pathophysiology involved in central myelinolysis. Other treatments include phenytoin or demeclocycline 150 to 300 mg PO every 6 hours to inhibit ADH.
Diagnosis of CSW
The diagnosis of CSW includes the following parameters:
- Serum sodium < 134 mEq/L
- Low serum osmolality (<280 mOsm/L)
- Urine osmolality normal or elevated
- High urinary sodium (>18 mEq/L)
- Low CVP, pulmonary capillary wedge pressure (PCWP), and pulmonary artery diastolic pressure (PAD)
- Low vascular volume
- Dehydration
Symptoms of CSW
The symptoms of CSW include headache, confusion, lethargy, nausea/vomiting, muscle cramps, depressed deep tendon reflexes, and seizures; these can lead to coma and death.
Treatment of CSW
In treating CSW, volume replacement should include 3% NaCl IVF at 10 to 50 cc/hour and, if appropriate, salt tablets 2 to 3 g tid/qid. Fludrocortisone acetate increases sodium absorption but usually is not needed.
CSW usually ends within 2 to 3 weeks, at which time salt replacement should be discontinued.
Hypernatremia
Hypernatremia occurs when serum sodium is > 150 to 155 mEq/L. However, symptoms do not present until later, when serum sodium is > 160 mEq/L and serum osmolality is > 330 mOsm/L. At this point, the patient may exhibit confusion, lethargy, and seizure. Serum sodium > 180 mEq/L can be associated with increased rates of mortality, but the mortality may be the result of a life-ending process, not necessarily of the hypernatremia.
To determine the amount of fluid needed to correct the hypernatremia, the total body water (TBW) deficit should be calculated. Normal TBW averages 60% but ranges from 50 to 70%, more in younger men and less in older women.
Fluid deficit in liters = (Na current – Na normal 140 mEq/L/140 mEq/L)
×(TBW: normal 60% of body weight [kg])
If current sodium in, for example, a 70 kg man is 170 mEq/L, the free water deficit would be
(170 – 140)/140 ×(0.6 ×70)
30/140 ×42 = 8.99 L
The deficit of free water must be replaced slowly, usually one half over 24 hours and the remainder over 1 or 2 days.
As with hyponatremia, there are three states: isovolemic, hypervolemic, and hypovolemic. Isovolemic hypernatremia is seen with sweating, use of isotonic fluids to replace hypotonic losses, and diabetes insipidus with sufficient fluid replacement. In diabetes insipidus, there is an 85% loss of ADH capacity. It is seen as a familial condition; occurs idiopathically, post-trauma, and with craniopharyngioma, lymphoma, neurosarcoidosis, meningitis, autoimmune diseases, and aneurysm; is linked to the administration of mannitol, phenytoin, furosemide, hydrochorothiazide, and ethanol; is seen in brain death or impending brain death; and is associated with Wegener’s granulomatosis and lymphocytic hypophysitis.
Hypervolemic hypernatremia results from the use of hypertonic solutions and mineralocorticoid excess. Hypervolemic hypernatremia is seen in hypotonic fluid loss such as in burn patients and with diarrhea, vomiting, and nasogastric suctioning. It is also seen in adrenal insufficiency, chronic renal failure, and after diabetes insipidus has started without correction. The osmotic diuresis from mannitol use or with hyperglycemia results in urine that is iso-osmotic or hyperosmotic, not hypo-osmotic as in diabetes insipidus.
When administering mannitol, the amount of osmolarity added to the serum from mannitol can be determined from the difference of the calculated serum osmolarity [mOsm/L = 2 × (Na mEq/L + K mEq/L) + (BUN/2.8) + (glucose/18)] compared with the measured serum osmolality.
Diagnosis of Hypernatremia
The diagnosis of hypernatremia includes the following parameters:
- High urine output (>250 mL/hour more than input fluids)
- Large water loss in urine relative to sodium loss, with resultant high normal or above normal serum sodium
- Low urine specific gravity (<1.005)
- Low urine osmolarity (50–150 mOsm/L)
- High serum osmolality (>290–295 mOsm/L)
After craniopharyngioma or pituitary surgery, there can be different types of diabetes insipidus. For example, there can be a transient phase, which occurs 12 to 36 hours postop; a prolonged phase, in which sodium remains abnormal for months or permanently; and a triphasic phase, in which injury to the pituitary reduces ADH for 4 to 5 days. This is then followed by cell death, liberating ADH for 4 to 5 days, during which time the diabetes inspidus resolves. The final phase is defined by the absence of ADH, either short-term or prolonged.
Symptoms of Hypernatremia
Symptoms of hypernatremia include confusion, loss of consciousness, tonic-clonic seizures, and rhabdomyolysis. There is no evidence that it causes intracranial hemorrhage.
Treatment of Hypernatremia
Treatment of hypernatremia includes
- Desmopressin (DDAVP) IV 1 to 5 μg (works for 8–20 hours) or
- Arginine vasopressin intramuscularly (IM), SQ. 12.5 μg (works for 4–8 hours) or
- Desmopressin (DDAVP) intranasally 1 to 5 μg (works for 12–20 hours)
Check laboratory tests and urine specific gravity every 6 hours.
Replace urine output greater than input with 0.45 normal saline, or use GI system through a feeding tube to replace with free water. Do not give free water IV if it can be avoided. The rapid correction can cause cerebral edema and permanent neurologic damage. One half of the water deficit as calculated above should be corrected in 24 hours and the remainder over 1 to 2 days; the rate of correction should not exceed 10 to 12 mmol/L/24 hours in an attempt to prevent further cerebral edema.
Other Electrolyte Imbalances
Magnesium
This cation occurs both as protein bound and as a free divalent ion. Magnesium is lost with acidosis, diuresis, extracellular fluid expansion, diarrhea, alcoholism, and phosphate depletion. Low magnesium (<1.5 mg/dL) is associated with seizures, confusion, and cardiac disturbances.63 Treatment consists of 25% magnesium sulfate 2 to 4 g every 4 hours. Magnesium may block the N-methyl-D-aspartate (NMDA) receptor, decreasing the neuroexci-totoxic reaction that occurs with brain injury; may help decrease seizures; and may help decrease cerebral vasospasm.
Calcium
Free ionized calcium is controlled by the parathyroid hormone (PTH) and vitamin D. PTH stimulates the release of calcium from the bones, reabsorption from the kidneys, and absorption from the GI tract. Hypocalcemia (<8.5 mEq/L) may be seen with phenytoin use, albumin, alkalosis, low magnesium, sepsis, pancreatitis, vitamin D deficiency, hypoparathyroidism, and rhabdomyolysis. Low serum albumin will decrease total calcium but will not affect ionized calcium. Therefore, ionized calcium should be measured, not calculated. Low calcium is associated with neuronal irritability such as seizures, confusion, paresthesias, hypotension, arrhythmias, sinus tachycardia segment prolongation, apnea, stridor, and no response to norepinephrine or dopamine. If calcium is suspected to be low, then the magnesium level should be checked and, if low, corrected. Ionized calcium levels must be checked, and low levels should be treated with 100 to 200 mg in 50 to 100 mL D5W bolus, followed by 1 to 2 mg/kg/hour infusion for 6 to 12 hours.
Potassium
Potassium is an intracellular cation whose release by the kidney is controlled by aldosterone. Only 0.4% of total body potassium is present in the plasma; therefore, intracellular stores can effectively replenish extracellular pools. During times of brain damage, cellular acidosis causes potassium to leave the cell for the intravascular space. Other causes include diabetic ketoacidosis, angiotensin converting enzyme inhibitors, β-blockers, digoxin, heparin, nonsteroidal anti-inflammatory drugs (NSAIDs), trimethoprim-sulfamethoxazole, rhabdomyolysis, and rewarming after hypothermia. Hyperkalemia (K+ > 5.5 mmol/L) produces paralysis, muscle weakness, dysesthesia, and cardiac disturbances due to neuroexcitability of cellular membranes. Treatment of hyperkalemia consists of 10 mL calcium gluconate 10% solution containing 93 mg of elemental calcium. Calcium antagonizes the cardiac muscle toxicity for 20 to 60 minutes. Sodium bicarbonate, as well as insulin and glucose infusion, causes enhanced activity of Na+–K+ adenosinetriphosphatase, moving K+ into the cell. This helps stabilize the neurologic and neuromuscular toxicity. However, sodium bicarbonate may bind calcium, making its action ineffective. Cation exchange resins such as polystyrene sulfonate (Kayexalate) removes 1 mEq K+ from the body for each gram given. The usual dose is 20 to 50 g dissolved in 100 to 200 mL 20% sorbitol given every 3 hours, up to 5 doses per day. However, should there be any suggestion of renal failure, hemodialysis should be instituted as soon as possible.
< div class='tao-gold-member'>
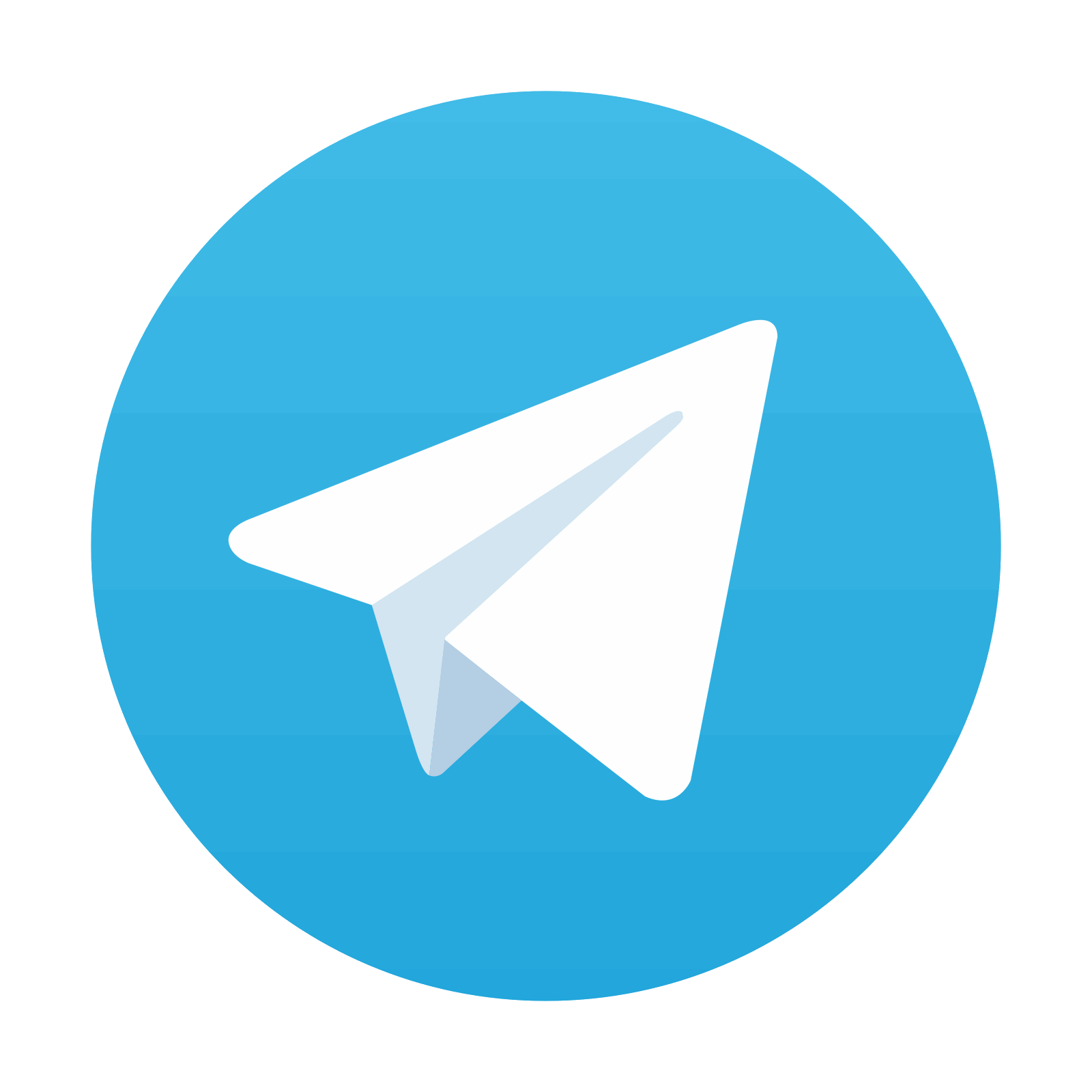