Diagnosis of Fluid and Electrolyte Disorders
The diagnosis of fluid and electrolyte disorders is complicated by the often-nonspecific symptoms and physical examination findings, which patients suffering from these disorders manifest. A thorough understanding of the homeostatic mechanisms that control fluid and electrolyte balance is essential to the accurate assessment and treatment of the wide variety of pathologies the emergency physician may encounter.
Approximately 60% of an adult male’s body weight is composed of water (less in women and the elderly), of which 2/3 is intracellular and 1/3 is extracellular. Of the extracellular volume, 3/4 is in the interstitial compartment and 1/4 within the vasculature. Osmolality is maintained within a narrow range (285–295 mOsm) and is the same across all fluid compartments; it may be estimated using serum sodium, glucose, and blood urea nitrogen (BUN) values (see Appendix).
Shifts in volume, osmolality, and electrolyte concentrations can occur independently of each other and typically manifest in profoundly different ways. The severity of symptoms is usually contingent on how rapidly the shifts occur. Changes in volume are most readily apparent on the cardiovascular physical examination. Signs and symptoms attributable to changes in osmolality are primarily neurological, resulting from brain dehydration (in hyperosmolar states) or brain edema (in hypoosmolar states). Electrolyte changes are more variable and exert their effect primarily on cell membrane potential with resultant cardiac, neurologic, and musculoskeletal dysfunction. See Table 44–1 for a list of serum electrolytes and normal values.
Sodium (Na+) | 136–146 mEq/L |
Potassium (K+) | 3.5–5 mEq/L |
Chloride (Cl–) | 96–106 mEq/L |
Bicarbonate (HCO3-) | 24–28 mEq/L |
Calcium (Ca2+) | 8.5–105 mg/dL (4.2–5.2 mEq/L) |
Magnesium (Mg2+) | 1.8–3 mg/dL (1.5–2.5 mEq/L) |
Phosphate (PO43−) | 3–4.5 mg/dL (1–1.5 mmol/L) |
Management of Specific Disorders
- Hyponatremia reflects a relative inability to excrete free water
- Symptoms relate to the rate of change
- Measure urine osmolality and sodium
- Review medications and assess thyroid and adrenal function
- Initial treatment is guided by the severity of symptoms and the patient’s volume status
Hyponatremia is defined as a serum sodium below 136 mEq/L and is associated with a number of different drugs and disease processes. Hyponatremia may be hyper-, hypo-, or isotonic. Isotonic hyponatremia is the result of laboratory artifact due to a decreased water component of plasma such as may be seen in hyperlipidemia and hyperproteinemia. Newer ion-specific methods of laboratory analysis have essentially eliminated this as a cause of hyponatremia. Hypertonic hyponatremia results from the presence of solutes that do not freely cross cell membranes, such as mannitol and glucose (in the absence of insulin), and represents a hyperosmolar state. With prolonged hyperglycemia, moderation of the hyponatremia or even hypernatremia may result as an osmotic diuresis develops.
Most hyponatremia is hypotonic, representing an excess of total body water relative to total body sodium (which may be low, normal, or even high). In the absence of renal failure, increased levels of arginine vasopressin (AVP), from drugs, paraneoplastic syndromes, or other endocrine abnormalities, or rarely excess free water intake prevent the kidneys from excreting sufficient free water. Hypotonic hyponatremia can be associated with normal, increased, or decreased volume, and knowledge of the patient’s urine sodium concentration and osmolality is invaluable in determining the underlying etiology (Figure 44–1).
Symptoms are rare with sodium above 125 mEq/L and when present reflect worsening cerebral edema, ranging from headache, nausea, vomiting, and mental status changes, culminating in seizures, coma, brain-stem herniation, and death. More severe symptoms are associated with a more rapid decrease in serum sodium. The clinician should correlate the measured serum sodium with the patient’s presentation to eliminate spurious hyponatremia, such as that produced by a sample contaminated with a hypotonic infusion. In hyperglycemic states, every 100 mg/dL of glucose decreases the measured serum sodium value by 1.5–1.7 mEq/L, and the clinician should calculate the actual serum sodium accordingly.
The urgency of treatment is dictated by the severity of symptoms and how abruptly the hyponatremia is thought to have developed. Correction of hyponatremia is controversial, but in acutely symptomatic patients even a 5% increase may be physiologically significant. In the presence of severe hyponatremia (sodium < 120 mEq/L and symptoms to include mental status changes, seizure, or coma), an infusion of 3% hypertonic saline solution (513 mEq/L) should be considered (see Appendix for formulas). The rate of rise of 1 mEq/L/h should be targeted for the first 3–4 hours or until symptoms resolve; otherwise, the rate of correction should not exceed 0.5 mEq/L/h. Simultaneous use of furosemide to limit expansion of the extracellular-fluid volume may be required. A new class of AVP receptor antagonists (the “vaptans”) has been approved for the treatment of euvolemic and hypovolemic hyponatremia, but experience with these agents in the ED is lacking. Resolution of symptoms, particularly in the elderly, may lag behind correction of the serum sodium, and frequent monitoring is important to avoid overcorrection.
Consideration should be given to thyroid and adrenal insufficiency, and replacement hormones given after appropriate laboratory samples have been obtained if indicated, and potassium replacement instituted if needed. Patients who do not require urgent treatment may be treated with fluid restriction and observation. Profoundly hypovolemic patients may be treated initially with fluid resuscitation using isotonic saline to prevent cardiovascular compromise. Emergency dialysis should be considered for patients with renal failure, volume overload, and severe hyponatremia.
Osmotic demyelination, the most feared consequence of too rapid correction, is rare at a rate of correction below 10–12 mEq/L/d. Alcoholics, hypokalemic patients, burn victims, and women taking thiazide diuretics are more at risk for this consequence. Symptoms of osmotic demyelination include dysarthria, dysphagia, spastic paresis, coma, and occasionally seizures. These symptoms may be delayed for 2–6 days after correction of the serum sodium and imaging by CT may be negative up to 4 weeks. Rapid reinduction of hyponatremia may be beneficial when this disorder is suspected.
All patients with clinically significant hyponatremia should be hospitalized. ICU placement should be considered for those with severe mental status changes or seizures.
- Hypernatremia may result from the loss of pure water or hypotonic fluid, or rarely from salt gain
- Symptoms are primarily neurologic and those related to severe hypovolemia
- Measure urine osmolality and sodium concentration
- Use isotonic saline to treat volume deficits on an emergency basis, followed by hypotonic solutions to gradually replace free water deficits
Hypernatremia is defined as a serum sodium above 146 mEq/L, and unlike hyponatremia, it always represents a hyperosmolar state with cellular dehydration. It represents a relative excess of sodium to free water and may result from net water loss, either pure water or hypotonic fluid loss or sodium loading, usually accidental or iatrogenic (Table 44–2). Hypernatremia in outpatients is more common at the extremes of age or in debilitated patients. Although present in only 1% of hospitalized patients, it has a mortality approaching 60%. As with hyponatremia, the severity of symptoms is related to the rate at which they develop.
|
Symptoms from hypernatremia reflect CNS dysfunction and are more prominent with large or rapid changes in serum sodium. Infants with hypernatremia may have muscle weakness, restlessness, a high-pitched cry, lethargy, and coma. Elderly patients typically have few or no symptoms until the sodium is over 160 mEq/L. Loss of thirst and progressive decrease in level of consciousness are associated with worsening hypernatremia, but seizures are rare in the absence of aggressive rehydration. When sodium changes occur rapidly, traction on the cerebral vasculature from brain shrinkage may result in tearing with subarachnoid, intraparenchymal, and subdural bleeding. Patients may be clinically hyper-, hypo-, or euvolemic. Skin turgor may be normal despite intravascular volume depletion.
Evaluation of urine osmolality and sodium concentration is necessary to determine the underlying cause, particularly in hypovolemic patients. Hypovolemia with a urine sodium concentration less than 20 mEq/L is consistent with extrarenal losses. Urine osmolality over 400 mOsm/L represents intact renal free water conservation, while dilute urine (less than 250 mOsm/L) is associated with diabetes insipidus (see Chapter 41).
In cases of circulatory compromise, volume deficits should be treated initially with isotonic saline. Otherwise, only hypotonic solutions should be used. In hypernatremia that has developed over a period of hours, rapid correction (∼1 mEq/L/h) does not increase the risk of convulsions or brain damage. When the rate of development is unknown, a rate of correction no greater than 0.5 mEq/L/h, or approximately 10 mEq per day, is recommended to avoid cerebral edema (see Appendix).
Hospitalization is recommended for all symptomatic patients and patients with a sodium greater than 150 mEq/L. Patients with mild hypernatremia, an intact thirst mechanism, and close follow-up may be discharged home.
Over 98% of the body’s potassium is intracellular, making it the predominant intracellular electrolyte. Because of this, it is often difficult to make extrapolations regarding total potassium stores from serum potassium concentrations. Potassium is sensitive to changes in serum pH caused by the accumulation of mineral acids. Intracellular acidosis promotes the extrusion of potassium with resultant hyperkalemia. In addition to aldosterone, circulating hormones such as insulin and catecholamines also affect the movement of potassium between the serum and intracellular compartments. Symptoms related to changes in serum potassium are related to changes in cell membrane potential, affecting primarily the heart and muscles.
- Usually associated with diuretic therapy
- Symptoms are primarily neuromuscular and cardiac
- Assess acid–base status
- Replace potassium orally whenever possible; IV potassium may be given with caution in severe cases with appropriate monitoring
Hypokalemia, defined as a serum potassium below 3.5 mEq/L, is the most common electrolyte disturbance and may represent decreased total body stores of potassium or a shift of potassium into cells. A decrease in total body potassium is most often secondary to diuretic therapy but may also be from increased GI or renal losses, or, in the setting of alcoholism or malnutrition, decreased intake (see Table 44–3). Concurrent digitalis therapy exacerbates the cardiac effects of hypokalemia. Potassium deficiency commonly coexists with other electrolyte abnormalities, particularly magnesium, and may remain refractory to therapy until the other abnormalities are corrected.
|
Symptoms of hypokalemia range from mild weakness, ileus, and rhabdomyolysis to paralysis and lethal arrhythmias (see Table 44–4). The severity of symptoms is often related to how quickly the decrease in serum potassium develops. Obtaining a venous pH may be helpful in determining initial treatment. EKG changes are present in over 80% of people with a potassium below 2.7 and may progress rapidly from decreased T wave amplitude to torsades de pointes (see Table 44–5).
On average, a decrease of 0.3 mEq/L in serum potassium is associated with a 100 mEq deficit in total body stores. Oral replacement using potassium chloride (20–40 mEq every 4 hours) is the preferred method for patients with mild symptoms who are able to tolerate oral intake. Higher doses should be avoided because of the risk of esophageal and gastric irritation or perforation in the presence of strictures. Potassium phosphate preparations may be used for patients with known phosphate deficiency, and potassium bicarbonate may be helpful in patients with severe metabolic acidosis.
For more severe symptoms and in patients with a serum potassium below 2.5, IV replacement is indicated. The concentration of potassium in IV solutions should not exceed 60 mEq/L (20 mEq/L if infusing through a peripheral line). More concentrated solutions are associated with greater pain at the infusion site and sclerosis of the veins. An IV infusion of 20 mEq/h is expected to produce an increase in the serum potassium of 0.25 mEq/h. In rare instances such as acute myocardial infarction with significant ventricular ectopy or in patients with paralysis of the respiratory muscles, rates of infusion as high as 40–100 mEq/h or more concentrated solutions may be indicated. Potassium should not be given in dextrose-containing solutions, as this may stimulate insulin release and result in worsening hypokalemia. All patients receiving IV potassium should have continuous cardiac monitoring, and it should never be given as a “push” because of the risk of precipitating life-threatening hyperkalemia.
All patients with a serum potassium below 2.5 mEq/L should be hospitalized in a monitored setting. Patients with less severe hypokalemia may be discharged with oral replacement therapy and close follow-up.
- Hyperkalemia is a true emergency
- Suspect hyperkalemia in patients with renal failure, diabetes, or those taking potassium supplements
- Symptoms are primarily neuromuscular and cardiac
- EKG findings may progress rapidly from peaked T waves to ventricular fibrillation
- Beware of spurious hyperkalemia
- Treatment stabilizes cardiac membranes, shifts potassium into cells, and removes potassium from the body
Hyperkalemia, defined as a serum potassium greater than 5.0 mEq/L, is more rare but more immediately life threatening than hypokalemia. It may rarely result from increased potassium intake but more commonly results from decreased renal excretion or transmembrane shift of intracellular potassium (see Table 44–6). Drugs that interfere with the renin-angiotensin-aldosterone system, such as ACE inhibitors or spironolactone, may result in hyperkalemia, as may drugs such as trimethoprim and triamterene that block secretion of potassium in the distal collecting duct. Digitalis toxicity may result in hyperkalemia through inhibition of the distal-collecting duct Na+/K+ ATP-ase.
|
Pseudohyperkalemia is frequently encountered and should be suspected when patients with normal renal function and no other evident precipitating cause for true hyperkalemia present with marked elevation in measured serum potassium. Hemolysis, thrombocytosis, marked leukocytosis, prolonged tourniquet time, and long delays between obtaining the lab specimen and analysis may result in release of intracellular potassium within the specimen; analysis of plasma potassium through a specimen collected in a heparinized tube may help differentiate true versus pseudohyperkalemia, with a difference of greater than 0.3 mEq/L suggestive of pseudohyperkalemia.
Symptoms attributable to hyperkalemia are nonspecific and include muscular weakness, fatigue, paresthesias, palpitations, and cardiac arrhythmias. EKG changes may progress rapidly from peaked T waves to QRS widening and ventricular fibrillation (see Table 44–7 and Figure 44–2); however, the presence of EKG changes poorly sensitive for a serum potassium over 6.0 mEq/L and may be absent in up to 80% of patients with significant hyperkalemia. Hyperkalemia is commonly associated with metabolic acidosis, and a venous blood gas may be helpful in guiding treatment. Evaluation of renal function and serum electrolytes is helpful in identifying contributing causes.
Figure 44–2.
Correlation between serum potassium concentration and EKG findings. The correlation is approximate and depends on serum pH and concentrations of other ions (particularly calcium and magnesium). (Reproduced, with permission, from Schroeder SA [ed.]: Current Medical Diagnosis & Treatment 1992. Appleton & Lange, Norwalk, CT, 1992.)
Emergency treatment of hyperkalemia is warranted for true hyperkalemia exceeding 6.0 mEq/L or in the presence of any EKG changes consistent with hyperkalemia. The goals of therapy are aimed at stabilizing membrane potential, moving potassium intracellularly, and eliminating potassium from the body. Caution should be exercised in patients with conditions such as diabetic ketoacidosis (DKA), in which total body potassium may actually be low and concurrent correction of the acid–base disorder with overly aggressive therapy for hyperkalemia may result in hypokalemia.
Patients with EKG changes or potassium over 7.0 should receive IV calcium, which directly antagonizes the effect of potassium on cardiac membrane potential. Both calcium gluconate and calcium chloride are available as 10% solutions, although calcium chloride contains three times as much available calcium and is more irritating if it extravasates and may worsen acidosis. For these reasons, calcium gluconate is preferred; 10 mL may be given IV over 2–5 minutes and repeated in 10 minutes if there is no effect on the EKG. Effects may be seen within 1–3 minutes and last 30–60 minutes. In patients with hyper-kalemia associated with digoxin toxicity, calcium administration should be avoided since calcium potentiates the myocardial toxicity of digoxin. Hypertonic saline may be used in hyperkalemic patients with significant hyponatremia as well.
Ten units of regular insulin may be given IV along with 50 mL of D50 (to avoid precipitating hypoglycemia, if necessary) to promote cellular uptake of potassium, with onset within 20 minutes and peak effect between 30 and 60 minutes. Ten to twenty milligrams of nebulized albuterol (or 0.5 mg IV) may also be used, with a slightly delayed onset and peak effect compared to insulin. Infusion of sodium bicarbonate alone has a variable effect and should not be used as monotherapy (particularly in dialysis patients), but it has been shown to potentiate the potassium-lowering effects of insulin and β-agonist therapy.
Direct elimination of potassium from the body by methods other than dialysis is the slowest method of reducing serum potassium and should not be used alone in the presence of significant hyperkalemia. Sodium polystyrene sulfonate may be given orally or as an enema in a dose of 25–50 g. The use of sorbitol as a laxative has been associated with intestinal necrosis and perforation, particularly in postoperative patients, and other laxatives may be preferable. Patients with intact renal function may benefit from therapy with potassium-losing diuretics. Dialysis should be considered in patients with renal failure or unstable patients, following institution of the above measures.
All patients with hyperkalemia requiring treatment should be admitted to a monitored setting.
Calcium is required for a large number of physiologic processes ranging from nerve conduction to blood coagulation. Calcium is commonly reported in mg/dL, which may be converted to mmol by dividing by 4 or to mEq by dividing by 2. Ninety-eight percent of calcium is bound to bone, and the regulation of extracellular calcium concentrations within the narrow range of 8.5–10.5 mg/dL is complex. Calcitonin, parathyroid hormone (PTH), vitamin D, phosphate, and calcium itself interact through a number of interlocking feedback loops to exert effects on the renal tubules, intestine, and bones.
Serum calcium is approximately 40% protein bound and 10% complexed with organic anions, leaving only 50% ionized. This is the physiologically active portion of serum calcium, which is highly sensitive to changes in pH. Rapid changes in pH may result in symptomatic hypo- or hypercalcemia even with normal serum calcium. As the serum pH increases, more calcium is bound, leaving a lower fraction available for use. Conversely, acidemia increases the amount of calcium available, with a change of 0.1 in pH roughly corresponding to a change in ionized calcium of 0.05 mmol/dL. Also, 1 mg/dL of albumin binds 0.8 mg/dL (0.2 mmol/dL) of calcium, which may be used to estimate expected changes in serum calcium in the setting of hypoalbuminemia; however, there is wide variability, and direct measurement of ionized calcium is preferable to calculation whenever hypo- or hypercalcemia is suspected.
- Occurs often in critically ill patients, although rarely life-threatening in itself
- Usually asymptomatic until severe
- Neuromuscular and respiratory symptoms predominate
- Often associated with disorders of magnesium and phosphate
- Always check serum phosphate before replacing calcium IV
- Use caution in giving IV calcium to patients taking digoxin
Hypocalcemia is defined as an ionized calcium less than 1.1 mmol/L, although symptoms are uncommon until the ionized calcium falls below 0.7 mmol/L. Patients with low serum calcium may have a normal ionized calcium. Hypocalcemia may develop gradually from decreased intake or absorption and is commonly associated with decreased PTH and vitamin D levels. It may also be seen with “hungry bone” syndrome, which results after parathyroidectomy for hyperparathyroidism. Hyperphosphatemia and hypomagnesemia commonly coexist with hypocalcemia. More acute changes in calcium may result from shifts in serum pH and the increased concentration of organic anions, such as citrate in the case of massive transfusion or fatty acids in acute pancreatitis. Up to 50% of critically ill patients, particularly those in sepsis, will have hypocalcemia.
Symptoms of acute hypocalcemia are primarily neuromuscular, including circumoral numbness, paresthesias, and carpopedal spasm, progressing to tetany, laryngospasm, and seizures (see Table 44–8). Skeletal muscle fasciculations, Chvostek’s and Trousseau’s signs, and hyperreflexia may be evident on physical examination. Hypocalcemia that develops more slowly may manifest primarily with weakness, irritability, and neuropsychiatric symptoms. Prolongation of the QTc interval without changes in morphology or other intervals is the most common EKG finding in hypocalcemia. Torsades de pointes with hypocalcemia is less common than with hypokalemia or hypomagnesemia.
|
Evaluation of the patient with hypocalcemia should include measurement of other serum electrolytes (particularly magnesium, phosphate, and potassium), renal function, serum albumin, and a venous or arterial blood gas to evaluate the patient’s acid–base status.
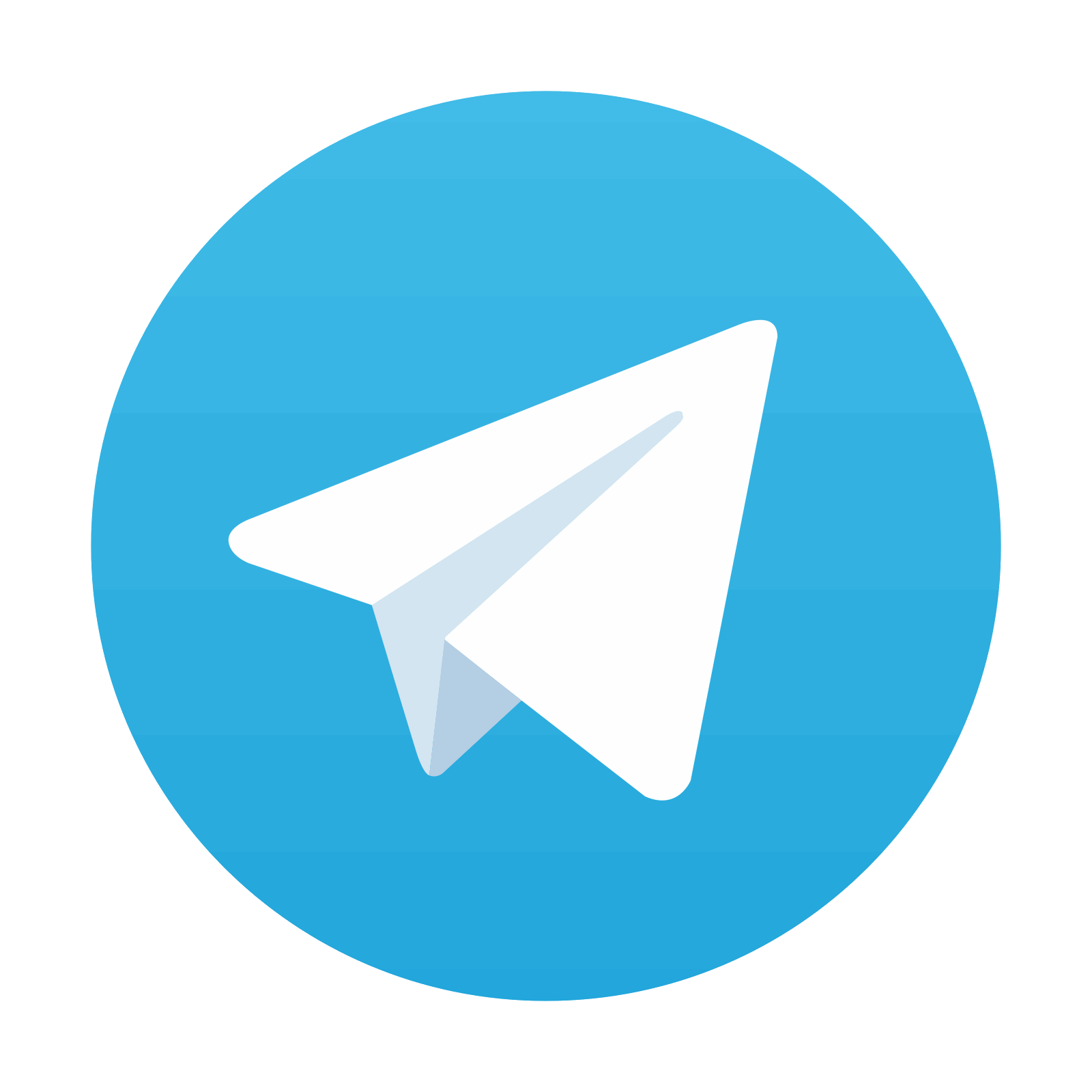
Stay updated, free articles. Join our Telegram channel

Full access? Get Clinical Tree
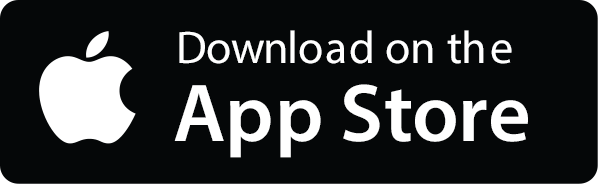
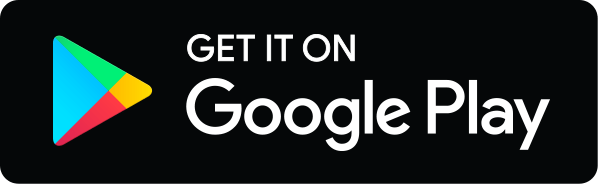