Bronchoscopy-associated hypoxemia may be minimized by providing 100% oxygen during the procedure, shortening bronchoscopy time, and frequently withdrawing the bronchoscope from the airway to allow adequate ventilation. Adequate tidal volume delivery should be monitored by observing chest excursions and exhaled tidal volumes in patients undergoing mechanical ventilation. Tidal volume and flow rates must be adjusted to provide adequate ventilation (1,33,39).
Complications associated with the administration of sedation, analgesia, and topical anesthesia include hypotension and allergic reactions, as well as hypoventilation, and hypoxemia from oversedation and respiratory depression. The overzealous use of local anesthetic agents within the airways has potential for toxicity with the rapid uptake of these agents into the systemic circulation from the bronchial mucosa (40). Lidocaine is the most commonly used airway anesthetic. The risks of toxicity are decreased with total doses of less than 4 mg/kg of body weight. The duration of airway anesthesia induced by lidocaine is approximately 20 to 40 minutes. Lidocaine in excessive doses can cause sinus arrest and atrial ventricular block, especially in patients with underlying heart disease. Other potential adverse reactions include respiratory arrest, seizures, laryngospasm, and, rarely, hypersensitivity reactions. Although not as commonly used for topical anesthesia, benzocaine has been associated with the development of methemoglobinemia (41). For reversal of benzodiazepine sedation, flumazenil, a competitive antagonist at GABA receptor, in the dose of 0.2 mg every 60 seconds up to 1 mg can be used. Naloxone is the competitive opioid antagonist which can be used for reversal of opioid-induced respiratory depression. Typical dose of naloxone is 0.1 to 0.2 mg, which can be repeated in every 2-minute interval. Ready availability of these agents is essential if bronchoscopy is being performed under conscious sedation.
Although rarely associated with bronchoscopy, dysrhythmias are more likely to occur in critically ill patients (42). Major cardiac dysrhythmias occur in 3% to 11% of all patients undergoing bronchoscopy. Hypoxemia is the major risk factor for the development of dysrhythmias (43,44).
Laryngospasm (in the nonintubated patient) or bronchospasm can occur in any patient undergoing flexible bronchoscopy, but are more common in patients with pre-existing reactive airway disease. Preoperative bronchodilator therapy significantly reduces the risk of bronchoscopy-induced bronchospasm in most patients with reactive airway disease (45).
Although transbronchial biopsy is a relatively safe procedure in patients with normal hemostasis and pulmonary vascular pressures, it is associated with a 2.7% and 0.12% risk of morbidity and mortality, respectively (46). Hemorrhage (more than 50 mL of blood) is more likely to occur in patients who undergo transbronchial biopsy; risk factors for hemorrhage include thrombocytopenia, coagulopathy, uremia, and pulmonary hypertension. Transbronchial biopsy should be restricted to nonuremic patients with platelet counts greater than 50,000 cells/μL and prothrombin times and activated partial thromboplastin times less than twice that of controls (29,46,47). The incidence rate of bronchoscopy-related hemorrhage in normal hosts approaches 1.4%. In immunocompromised hosts, the rate of hemorrhage ranges from 25% to 29%, while hemorrhage occurs in as many as 45% of uremic patients (29,48,49). Administration of desmopressin, 0.3 μg/kg, can reverse the uremic effect on platelet function, although no controlled study evaluating the safety of performing transbronchial biopsies after treatment with desmopressin exists (50). Pneumothorax occurs in fewer than 5% of nonventilated patients undergoing transbronchial biopsy. Tube thoracostomy is required in approximately half of these patients (29). A major risk factor for pneumothorax is positive pressure ventilation, especially if PEEP is used. Rates of pneumothorax after transbronchial lung biopsy in mechanically ventilated patients have been reported up to 7% and 23% (23,51,52). Fluoroscopic guidance may diminish the risk of pneumothorax. No patient should undergo bilateral transbronchial biopsy procedures during the same bronchoscopy session because of the small risk of bilateral pneumothorax.
Postbronchoscopy fever occurs in as many as 16% of patients. Bronchoscopy-related pneumonia is rare, occurring in fewer than 5%, and bacteremia is exceedingly rare (53,54). In general, endocarditis prophylaxis is not required with flexible bronchoscopy (55).
Neurosurgical patients are at risk for intracranial hypertension as a result of bronchoscopy-induced elevation of intrathoracic pressure, arterial hypertension, and hypercapnia. Bronchoscopy-associated cough or retching must therefore be avoided. Deep sedation with or without neuromuscular blockade may be utilized if bronchoscopy is deemed necessary.
DIAGNOSTIC AND THERAPEUTIC BRONCHOSCOPY
Airway Management
Flexible bronchoscopy can provide an efficient and effective means to secure a difficult airway, change an ETT, and inspect an airway during extubation (56,57). Endotracheal intubation can be technically difficult in select patient groups (Table 38.2). Intubation using flexible bronchoscopy under topical anesthesia, with or without conscious sedation, is an important technique in these patients with compromised airways, particularly if the airway is obstructed or if the trachea is extrinsically compressed by a mediastinal mass. Spontaneous ventilation keeps the airway open and assists the bronchoscopist in locating the glottis when airway anatomy is distorted (56,58). Bronchoscopic examination of the airway also identifies the nature of the obstructed airway and helps to plan for additional therapeutic maneuvers to relieve the airway obstruction.
Bronchoscopic endotracheal intubation can be performed using either a nasal or oral approach. With the nasal approach, after preparation of the nasal mucosa with a local anesthetic such as lidocaine and a mucosal vasoconstrictor such as 1% phenylephrine, the bronchoscope is passed through the nares and situated directly above the glottic opening. It is then passed into the trachea and the ETT is passed over the bronchoscope into the trachea. The major limitation of this approach in many ICU patients with concomitant abnormalities of coagulation is epistaxis and the potential for sinusitis with prolonged nasal intubation. The development of epistaxis can significantly impair the bronchoscopic examination and can seriously hamper subsequent nasal or laryngoscopic attempts at intubation. Other difficulties associated with nasal intubation include adenoid dislocation and difficulty passing the ETT in patients with a limited diameter of the nares.
TABLE 38.2 Factors Associated with Difficult Endotracheal Intubations |
![]() |
Oral flexible bronchoscopic intubation effectively avoids these difficulties associated with nasal intubation. Topical anesthesia of the oropharynx is achieved with spraying of 4% lidocaine. Translaryngeal injection of 3 mL of 4% lidocaine through the cricothyroid membrane to provide topical anesthesia to the larynx and trachea, in addition to lidocaine sprays to the oropharynx, is favored by some practitioners although use is discouraged due to the invasive nature and need for special training. Others favor a “spray as you go” technique, with injection of lidocaine through the working channel of the bronchoscope to provide laryngeal and tracheal topical anesthesia. Alternatively, nebulization of 6 to 8 mL of 4% lidocaine is used for topical anesthesia in some institutions (56). A bite block should be in place to prevent scope damage from the patient biting. In some patients, the use of an oral intubating airway, such as the Williams Airway Intubator, the Ovassapian Airway, or the Berman Airway, may be helpful in successfully intubating the patient with a difficult airway (59). The oral intubating airway directs the flexible bronchoscopy past the tongue and directly over the larynx, facilitating endotracheal intubation. Use of these airways in the completely awake patient with inadequate topical anesthesia may be problematic due to gagging and vomiting. This is less of a problem in the sedated or unconscious patient. After exposure of the vocal cords, the bronchoscope is passed into the trachea and the ETT is then passed over the bronchoscope into the airway. In some patients, the ETT impinges on laryngeal structures despite the smooth entrance of the bronchoscope into the trachea. In this situation, the ETT may be withdrawn back over the bronchoscope, rotated 90 degrees clockwise or counterclockwise to change the position of the tube bevel relative to the larynx, and readvanced during inspiration (56). Mild to moderate conscious sedation may be used in some patients to improve patient comfort and tolerance of nasal or oral bronchoscopic intubation. Great caution should be taken in patients with highly compromised airways, however, and sedatives may need to be completely avoided.
In addition to the oral intubating airway, other airway adjuncts have been used in combination with the flexible bronchoscope for intubation of patients with a difficult airway. A special facial mask with a diaphragm for the bronchoscope has been developed for the critically ill and for use in the operating room (60). The mask is useful for bronchoscopic intubation in sedated or comatose patients with limited respiratory reserve, providing a tight seal for assisted ventilation during the procedure. The intubating laryngeal mask airway (LMA) has also been used successfully in combination with the flexible bronchoscope (61).
Flexible bronchoscopy allows for ETT changes in patients with ETT cuff leaks, inadequate ETT internal diameters, and nasotracheal tube–associated sinusitis. Before bronchoscopy, the oropharynx should be suctioned thoroughly. For oral tracheal intubation, the ETT should be shortened 2 to 3 cm at its proximal end and advanced over the bronchoscope before its placement in the pharynx. The bronchoscope tip is advanced to the level of the cuff of the existing ETT, and secretions are aspirated through the suction channel. If necessary, the cuff is deflated and the bronchoscope advanced into the tracheal lumen. The ETT is then withdrawn with the cuff fully deflated, the bronchoscope tip advanced to the carina, and the new ETT advanced over the bronchoscope into the trachea. Adequate positioning of the tube 3 to 4 cm proximal to the carina is confirmed by visual inspection, and the cuff is inflated. After intubation, a chest radiograph is not required to confirm adequate placement of the ETT (62). Tube changes by the oral or nasal routes are possible. Contralateral nasal reintubation, however, may be difficult because of the lateral displacement of the septum by the existing nasotracheal tube.
Percutaneous dilatational tracheostomy (PDT) has become a well-accepted method for performing bedside tracheostomy in the ICU. While not universally used, flexible bronchoscopy is routinely used in performing PDT (63). Bronchoscopy facilitates proximal positioning of the ETT prior to introducing the guidewire needle into the trachea, reducing the risk of ETT impalement by the needle, and facilitates reintubation if the ETT is dislodged out of the airway. Bronchoscopic visualization ensures that the guidewire needle is introduced in the appropriate interspace in a midline position and that the needle does not penetrate the membranous posterior tracheal wall, thereby decreasing the risk of misplacement of the tracheostomy tube and creation of a false paratracheal passage. Bronchoscopy also provides feedback to the operator during dilator passage so that pressure on the posterior wall is minimized and the potential for posterior wall tears is reduced.
Flexible bronchoscopy can be extremely useful in the placement of a double-lumen ETT. If a right-sided tube is used, adequate positioning of the tube with the tracheal port proximal to the carina and bronchial port proximal to the right upper lobe orifice can be confirmed by using a small-diameter (3.5-mm outer diameter) flexible bronchoscope to inspect the airway through each lumen (64,65). Positioning of left-sided tubes is not as problematic given the longer length of the left mainstem bronchus relative to the right mainstem bronchus and less likelihood of obstructing the left upper or left lower lobe. In general, bronchoscopic confirmation of proper bronchial port positioning should be performed after all double-lumen ETT intubations, given the significant rate of malpositioning with a blind technique (66).
Flexible bronchoscopy provides an excellent opportunity to inspect the airways at the time of extubation in patients at risk for airway compromise, including those intubated for inhalation injury, trauma, subglottic stenosis, and laryngeal edema. The bronchoscope is advanced through the ETT to its most distal aspect, and the ETT and bronchoscope are withdrawn slowly together to allow inspection of the airway. If bronchoscopy confirms persistent mucosal edema or airway obstruction, the ETT can be readvanced over the bronchoscope into the tracheal lumen and secured, with extubation postponed until a later time.
Atelectasis
Segmental or lobar atelectasis presents radiographically as a parenchymal density associated with a combination of shift of an interlobar fissure, crowding of vessels or bronchi, ipsilateral mediastinal shift, or elevation of the diaphragm. Complete lung atelectasis will produce opacification of the hemithorax and usually ipsilateral mediastinal shift. Atelectasis is most commonly due to mucous plugging; however, in patients who do not improve after chest physiotherapy, endobronchial obstruction due to endobronchial tumor, foreign body, or blood clot should be excluded by bronchoscopy. Predisposing conditions for mucous plugging and atelectasis include inadequate inspiratory effort (pain, sedation, and muscle weakness), immobility, obesity, excessive airway secretions, pre-existing airway disease, and endobronchial obstructing lesions. Lobar or whole lung atelectasis produces hypoxemia by right-to-left vascular shunting and ventilation/perfusion mismatching. The clinical significance of atelectasis is directly related to its extent and to the pre-existing pulmonary reserve of the patient.
Much of the evidence supporting the role of flexible bronchoscopy in the treatment of atelectasis is anecdotal. Success rates for bronchoscopy range from 19% to 89% (67). One randomized trial comparing bronchoscopy to aggressive chest physiotherapy and nebulizer therapy found no advantage for bronchoscopy, although the study methodology has been criticized (68–70). Patients with whole lung or lobar atelectasis tend to respond better than those with segmental atelectasis. With the exception of large, obstructing central airway mucous plugs, the radiographic response to successful removal of secretions is delayed from 6 to 24 hours. Therapeutic bronchoscopy is, in general, indicated for patients with life-threatening whole lung or lobar atelectasis and for patients who have not responded to chest physiotherapy measures. Chest physiotherapy should be continued after successful bronchoscopy to prevent new airway obstructions. Instillation of saline or a dilute 10% solution of acetylcysteine through the working channel may help to clear thick, tenacious secretions. Acetylcysteine is a bronchial irritant, however, and may exacerbate bronchospasm in patients with reactive airway disease. Typically 10 to 20 mL aliquots of saline are used as the irrigant to facilitate clearing of mucous plugs. If saline irrigation fails, then instillation of acetylcysteine or rhDNase (Pulmozyme) may be considered (71–73). In some patients, holding continuous suction while withdrawing the bronchoscope through the ETT allows removal of large mucous plugs that cannot be suctioned directly through the working channel. Extremely tenacious mucous plugs may require the use of biopsy forceps or a foreign body basket to be successfully extracted. Blood clots may similarly be removed with saline irrigation. Instillation of acetylcysteine may be helpful in more difficult to remove blood clots. The use of biopsy forceps or a foreign body basket may be needed to remove blood clots that cannot be removed with irrigation and suction extraction.
Selective intrabronchial insufflation by the flexible bronchoscope, preceded by suctioning of mucus from large airways, has been used in patients with refractory atelectasis (67). One study in a surgical ICU using air insufflation for lobar collapse reported an overall effectiveness of 82%, with 92% effectiveness when collapse was less than 72 hours’ duration (74). Although only minor clinically insignificant complications have been described, selective positive pressure insufflation does carry the potential risk of barotrauma (75–77).
Hemoptysis
Flexible bronchoscopy plays a central role in the evaluation of hemoptysis. Bronchoscopy should be considered in all critically ill patients with hemoptysis, regardless of the degree of hemoptysis, to localize the site of bleeding and attempt to determine the underlying etiology. Localization of the site of bleeding is important to guide temporizing therapy such as angiographic embolization and definitive therapy such as surgical resection. Early rather than delayed bronchoscopy should be performed to increase the likelihood of localizing the source of bleeding. Bronchoscopy performed within 48 hours of bleeding onset successfully localized bleeding in 34% to 91% of patients, depending on the case series, as compared to successful localization in 11% to 52% of patients if bronchoscopy was delayed (78). Bronchoscopy performed within 12 to 24 hours may provide an even higher yield.
Massive hemoptysis is defined as expectoration of blood exceeding 200 to 1,000 mL over a 24-hour period, with expectoration of greater than 600 mL in 24 hours as the most commonly used definition (79). In practice, the rapidity of bleeding and ability to maintain a patent airway are critical factors, and life-threatening hemoptysis can alternatively be defined as the amount of bleeding that compromises ventilation. Only 3% to 5% of patients with hemoptysis have massive hemoptysis, with the mortality rates approaching 80% in various case series. Most patients who die from massive hemoptysis do so from asphyxiation secondary to airway occlusion by blood and blood clots, not exsanguination. The causes of massive hemoptysis are listed in Table 38.3. Infections associated with bronchiectasis, tuberculosis, lung abscess, and necrotizing pneumonia are commonly responsible for the massive bleeding. Other common causes include bronchogenic carcinoma, mycetoma, invasive fungal diseases, chest trauma, cystic fibrosis, pulmonary infarction, coagulopathy, and alveolar hemorrhage due to granulomatous polyangiitis (Wegener granulomatosis) and Goodpasture syndrome.
TABLE 38.3 Potential Causes of Massive Hemoptysis |
![]() |
Airway patency must be ensured in patients with massive hemoptysis. While preparing for intubation and bronchoscopy, the patient may be positioned in the lateral decubitus position with the bleeding side down. Most patients with massive hemoptysis will require intubation and mechanical ventilation. While intubation generally preserves oxygenation and facilitates blood removal from the lower respiratory tract, the ETT may become obstructed by blood clots with inability to oxygenate and ventilate the patient. The largest possible ETT should be inserted to allow for use of bronchoscopes with a 2.8- to 3.0-mm working channel for more effective suctioning and to allow for better ventilation with the bronchoscope in the airway for prolonged periods of time. In severe cases, the mainstem bronchus of the nonbleeding lung can be selectively intubated under bronchoscopic guidance to preserve oxygenation and ventilation from the normal lung.
Some authors have recommended the use of a double-lumen ETT to isolate the normal lung and permit selective intubation. While double-lumen ETTs have been used successfully in the airway management of massive hemoptysis, there are a number of potential pitfalls. First, placement of a double-lumen ETT is difficult for less experienced operators, particularly with a large amount of blood in the larynx and oropharynx. Second, the individual lumens of the ETT are significantly smaller than a standard ETT and are at significant risk of being occluded by blood and blood clots. Lastly, positioning of the double-lumen ETT and subsequent bronchoscopic suctioning of the distal airways require a small pediatric bronchoscope with working channels of 1.2 to 1.4 mm. Adequate suctioning of large amounts of blood and blood clots through such bronchoscopes is extremely problematic. In one series of 62 patients with massive hemoptysis, death occurred in four of seven patients managed with a double-lumen ETT due to loss of tube positioning and aspiration (80). Related to this rationale, the use of double-lumen ETTs for airway management in massive hemoptysis is not recommended. As an alternative to selective mainstem bronchial intubation or intubation with a double-lumen ETT, an ETT that incorporates a bronchial blocker, such as the Univent tube, may be used.
Endobronchial tamponade with flexible bronchoscopy can prevent aspiration of blood into the contralateral lung and preserve gas exchange in patients with massive hemoptysis. Endobronchial tamponade can be achieved with a 4-French Fogarty balloon-tipped catheter. The catheter may be passed directly through the working channel of the bronchoscope or the catheter can be grasped by biopsy forceps placed though the working channel of the bronchoscope prior to introduction into the airway. The bronchoscope and catheter—with the latter held in place adjacent to the bronchoscope by the biopsy forceps—are then inserted as a unit into the airway. Care must be taken not to perforate the catheter or balloon by the forceps. The catheter tip is inserted into the bleeding segmental orifice, and the balloon is inflated. If passed through the suction channel, the proximal end of the catheter is clamped with a hemostat, the hub cut off, and a straight pin inserted into the catheter channel proximal to the hemostat to maintain inflation of the balloon catheter. The clamp is removed, and the bronchoscope is carefully withdrawn from the bronchus with the Fogarty catheter remaining in position, to provide endobronchial hemostasis (81–83). The catheter can safely remain in position until hemostasis is ensured by surgical resection of the bleeding segment or bronchial artery embolization. Right heart balloon catheters have been used in a similar fashion (84). A modified technique for placement of a balloon catheter has been described using a guidewire for insertion. A 0.035-in soft-tipped guidewire is inserted through the working channel of the bronchoscope into the bleeding segment. The bronchoscope is withdrawn, leaving the guidewire in place. A balloon catheter is then inserted over the guidewire and placed under direct visualization after reintroduction of the bronchoscope (85). The use of endobronchial blockers developed for unilateral lung ventilation during surgery may hold promise for management of massive hemoptysis in tamponading bleeding and preventing contralateral aspiration of blood (86). The Arndt endobronchial blocker is placed through a standard ETT and directly positioned with a pediatric bronchoscope. Suctioning and injection of medications can be performed through the lumen of the catheter after placement. The Cohen tip-deflecting endobronchial blocker is also placed through a standard ETT and directed into place with a self-contained steering mechanism under bronchoscopic visualization. At this time, there is limited published experience with these blockers in the setting of massive hemoptysis, although the authors have successfully used them for this application. The prolonged use of endobronchial blockers may cause mucosal ischemic injury and postobstructive pneumonia and their use beyond 24 to 48 hours is discouraged.
Additional bronchoscopic techniques may be useful as a temporizing measure in patients with massive hemoptysis. Bronchoscopically administered topical therapies such as iced sterile saline lavage or topical 1:10,000 or 1:20,000 epinephrine solution may be helpful (87). Direct application of a solution of thrombin or a fibrinogen–thrombin combination solution has been used (88). The use of bronchoscopy-guided topical hemostatic tamponade therapy using oxidized regenerated cellulose mesh has recently been described (89). Although anecdotal, one of us (MJ) has had success with topical application of 5 to 10 mL of a 1-mEq/mL (8.4%) sodium bicarbonate solution.
For patients who have hemoptysis due to endobronchial lesions, particularly endobronchial tumors, hemostasis may be achieved with the use of neodymium-yttrium-aluminum-garnet (Nd:YAG) laser phototherapy, electrocautery, or cryotherapy via the bronchoscope.
Diagnosis of Ventilator-Associated Pneumonia
For the diagnosis of VAP, the use of bronchoscopic modalities remains controversial and is often institution dependent. Although commonly attributed to pneumonia, the chest radiographic finding of alveolar infiltrates in the ICU patient can represent a broad differential diagnosis, requiring a wide range of therapies. The use of standard clinical criteria for the diagnosis of pneumonia such as new pulmonary infiltrates, hypoxemia, leukocytosis or leukopenia, fever, and pathogenic bacteria in respiratory secretions has been associated with a significant rate of misdiagnosis (90). Bacterial colonization of the upper airways and ETT can confound the reliability of the Gram stain and cultures from tracheal aspirates obtained in the intubated patient. Concern about the inaccuracy of clinical approaches to the diagnosis of VAP and the possibility of antibiotic overprescribing with a clinical strategy has led some investigators to postulate that bronchoscopic methods such as PSB and BAL would improve the diagnosis of VAP and treatment outcomes (21,22).
The methodology for performing PSB and BAL quantitative cultures is outlined in a previous section of this chapter. PSB and BAL should be performed in the most abnormal segment as determined by radiographic studies or where endobronchial abnormalities are most pronounced. Alternatively, samples may be obtained from the right lower lobe, as this is the most commonly affected area on autopsy studies. A quantitative culture result of more than 104 CFU/mL is considered diagnostic for pneumonia with BAL, while more than 103 CFU/mL is considered diagnostic for pneumonia with PSB. An evidence-based review of 23 prospective studies of BAL in suspected VAP showed a sensitivity of 42% to 93% with a mean of 73% ± 18%, and a specificity of 45% to 100% with a mean of 82% ± 19% (91). In 12 studies, the detection of intracellular organisms in 2% to 5% of recovered cells was used to diagnose pneumonia, with a mean sensitivity of 69% ± 20% and a specificity of 75% ± 28% (91). An advantage of looking for intracellular organisms is the ability to obtain information of high predictive value in a rapid time frame without waiting for the results of cultures to define the presence of pneumonia, although not the specific identity of the etiologic pathogen (90).
In a review of studies evaluating PSB, the sensitivity ranged from 33% to 100% with a median sensitivity of 67%, while the specificity ranged from 50% to 100% with a median specificity of 95% (92). It is unclear if BAL is superior to PSB or vice versa in the diagnosis of VAP. In a meta-analysis of 18 studies on PSB (795 patients) and 11 studies on BAL (435 patients), there was no difference in the accuracy of the two tests (93). BAL does offer an advantage in that additional microbiologic tests beyond routine bacterial cultures, as well as cytologic analysis, can be performed on the sample if an infectious process is suspected other than typical bacterial pneumonia. PSB may potentially have a greater complication rate compared with BAL, but this has not been formally compared.
Despite studies of BAL and PSB showing a greater accuracy than tracheal aspirates, the routine use of bronchoscopy for establishing the diagnosis of VAP remains controversial (90,94). This controversy is in part due to critiques in study methodologies and in part due to some studies showing a benefit in patient outcomes while others have not. One prospective, nonrandomized study noted a difference in mortality between patients managed with an invasive bacteriologic strategy (19%) versus those managed with a clinical strategy (35%) (95). One large, prospective randomized trial did show an advantage to the quantitative bronchoscopic approach when compared with a clinical approach in a multicenter study of 413 patients suspected of having VAP. Compared with patients managed clinically, those receiving invasive management had a lower mortality rate on day 14 (16% vs. 25%; p < 0.02), but not on day 28, and lower mean sepsis-related organ failure assessment scores on days 3 and 7. At 28 days, the quantitative culture group had significantly more antibiotic-free days (11 ± 9 vs. 7 ± 7 days; p < 0.001), but only a multivariate analysis showed a significant difference in mortality (hazard ratio 1.54; 95% confidence interval [CI] 1.10–2.16) (96). No differences in mortality were observed in three randomized single-center studies when invasive techniques (PSB and/or BAL) were compared with either quantitative or semiquantitative endotracheal aspirate culture techniques (97–99). However, these studies included few patients (58, 83, and 95, respectively), and antibiotics were continued in all patients, even those with negative cultures, thereby negating one of the potential advantages of the bacteriologic strategy. A meta-analysis of these randomized controlled trials noted that an invasive approach did not alter mortality (odds ratio 0.89, 95% CI 0.56–1.41), although invasive testing affected antibiotic utilization (odds ratio for change in antibiotic management after invasive sampling 2.85, 95% CI 1.45–5.59) (100).
Performing microbiologic cultures of pulmonary secretions for diagnostic purposes after initiating new antibiotic therapy can lead to false-negative results and is likely of little value regardless of the manner in which the secretions are sampled. Studies have demonstrated that culture positivity at 24 and 48 hours after the onset of antimicrobial treatment is markedly diminished (101,102). The decrease in positive cultures after initiation of antibiotic therapy appears to affect PSB more so than BAL. If patients have been treated with antibiotics but did not have a change in antibiotic class prior to bronchoscopy for a suspected new episode of VAP, the yield of BAL and PSB appears to be similar to that in patients who have not received antibiotics (103). If an invasive bronchoscopy strategy is used to establish a diagnosis of VAP, BAL, PSB, or both should be performed prior to administration of antibiotics or administration of new antibiotics if the patient was previously on antimicrobial therapy.
Diagnosis of other Respiratory Infections
Flexible bronchoscopy is an essential modality in evaluating the critically ill, immunocompromised patient with pulmonary infiltrates. These patients are at risk for fungal, viral, protozoal, mycobacterial, and atypical bacterial pulmonary infections. Critically ill patients who have no underlying immunocompromised condition, such as acquired immunodeficiency syndrome (AIDS), leukemia, neutropenia, hematopoietic stem cell/bone marrow transplant, or solid organ transplant, may also develop respiratory infections other than bacterial pneumonias, such as fungal and viral infections. In addition to these patients, those who present with acute respiratory failure and apparent community-acquired pneumonia, but fail to respond appropriately to antibiotic therapy, may benefit from bronchoscopy to evaluate for more unusual infections and noninfectious causes of acute respiratory failure with infiltrates. Bronchoscopy is not recommended for routine community-acquired pneumonia.
BAL, as compared to PSB, provides the opportunity for more extended microbiologic studies and for cytology and additive value of PSB to BAL is likely minimal. It is unclear if the addition of transbronchial biopsy to BAL improves diagnostic accuracy in all immunocompromised patients with pulmonary infiltrates but it potentially has a role in lung transplant recipients and recipients of hemopoietic stem cell transplants. Transbronchial biopsy may increase the yield for the diagnosis of infectious etiologies such as invasive viral and fungal infections, and may assist in establishing an alternate noninfectious cause of infiltrates in these patients (104–106). The benefits versus risks, including life-threatening pneumothorax and bleeding, need to be individualized in the critically ill immunocompromised patient.
In immunocompromised and critically ill patients who are suspected to have an atypical infection, BAL fluid should be sent for cytopathology to evaluate for viral cytologic changes, as well as Gomori methenamine silver staining (GMS) to evaluate for fungal organisms and Pneumocystis jirovecii. Alternatively, Papanicolaou, Giemsa, toluidine blue O, or direct fluorescent antibody staining may be used for detection of Pneumocystis.
For Pneumocystis in AIDS patients, BAL has a sensitivity rate in diagnosing Pneumocystis pneumonia of approximately 85% to 90%, and for transbronchial biopsy, the diagnostic yield approaches 87% to 95%. When BAL and transbronchial biopsy are performed in AIDS patients with Pneumocystis pneumonia, the diagnostic yield is 95% to 98% (107–110). Given the high yield of BAL and the potential risk of transbronchial biopsy in critically ill patients, transbronchial biopsy is not recommended in AIDS patients for diagnosing Pneumocystis. In immunocompromised patients without HIV, the yield of BAL is lower, and transbronchial biopsies to establish a diagnosis may be required. Although not used routinely in clinical practice, PCR for Pneumocystis on BAL specimens may increase diagnostic rates (111).
The criteria for proven fungal disease except for endemic mycoses, based upon the European Organization for Research and Treatment of Cancer/National Institute of Allergy and Infectious Diseases Mycoses Study Group (EORTC/MSG) criteria, rely upon visualization of fungal organisms on histopathologic or cytopathologic specimens or culture positivity from a normally sterile site (112). The criteria for probable invasive fungal disease except for endemic mycoses, in addition to radiographic criteria, include detection of mold on BAL/PSB cytology or culture. For the diagnosis of pulmonary fungal infections in immunocompromised patients, BAL fluid should be sent for fungal stains and cultures in addition to cytology stains. However, BAL cytology, microscopic examination, and culture tend to have low sensitivity. As noted, demonstration of fungal organisms on transbronchial lung biopsies is diagnostic of invasive fungal diseases, however, many patients with hematologic malignancies and recipients of hematopoietic stem cell transplants will not be candidates for transbronchial biopsies due to thrombocytopenia; the utility of biopsies in these patient populations is controversial. If transbronchial biopsies are obtained, the samples should be sent for culture in addition to histology. Some fungi, such as Mucor and Rhizopus, are difficult to grow in culture, and diagnosis relies on BAL cytology or biopsy specimens. Aspergillus is the most commonly encountered pulmonary fungal infection; BAL cytology and culture are diagnostic in approximately 50% to 60% of cases of invasive pulmonary aspergillosis. The diagnostic yield from BAL appears to be increased with the use of galactomannan antigen and PCR testing in small studies (113–115), however, a recent large single-center prospective cohort showed poor sensitivity and specificity of BAL galactomannan assay in diagnosis of invasive fungal diseases (116). Although duration of antifungal treatment prior to bronchoscopy has not been reported in all studies, the use of mold-active antifungal therapy for 2 to 3 days prior to bronchoscopy may decrease the sensitivity of BAL galactomannan (117,118). The presence of [1,3]-beta-D-glucan in BAL may be suggestive of pneumocystis, but the low positive predictive value limits its use in the diagnosis of invasive fungal pneumonia (119). Antigen testing for histoplasmosis and blastomycosis on BAL samples is now available (120).
To evaluate for viral infections, multiplex PCR is available and widely used for a comprehensive panel of respiratory viruses and atypical bacteria including Legionella pneumophila, Chlamydia pneumoniae, Mycoplasma, and Bordetella pertussis, and has significantly improved in detection of a pathogen (121,122). BAL cytology may demonstrate characteristic intracytoplasmic inclusions, but sensitivity is lacking. Immunofluorescence and PCR for cytomegalovirus (CMV) may be performed on BAL specimens (123).
If there is suspicion for tuberculosis or nontuberculous mycobacteria, BAL samples should be sent for acid-fast bacillus stains and mycobacterial culture. BAL samples of sputum smear–negative patients with tuberculosis are smear positive in 12% to 42% of patients and culture positive in 66% to 95% (124). In one-third to one-half of initially sputum smear–negative patients, bronchoscopy specimens yield the only positive source of mycobacterial tuberculosis (125–127). Nucleic acid amplification testing (NAAT) on BAL specimens for detection of mycobacterial tuberculosis and nontuberculous mycobacterial infection is routinely used in some institutions (128,129). NAAT for the diagnosis of tuberculosis is very sensitive and specific in patients with BAL smear-positive samples. In a meta-analysis of NAAT on BAL samples that are smear negative, the pooled sensitivity was 54% (130). Thus, a negative NAAT on a BAL sample that is smear negative does not exclude the diagnosis of tuberculosis.
For atypical bacterial infections, additional stains and cultures may be required. Legionella requires specific culture media; a direct fluorescence antibody stain is also available for Legionella. Some laboratories utilize specific media if Nocardia is suspected, and this agent can often be identified with a combination of Gram stain and modified Ziehl–Neelsen stains, and are observed as delicately branched, weakly gram-positive, variably acid-fast bacilli. Methenamine silver stains may demonstrate the organisms in tissue specimens.
Diagnosis of Noninfectious Pulmonary Infiltrates
Although most helpful in excluding infectious etiologies for pulmonary infiltrates in ICU patients, bronchoscopy with BAL and/or transbronchial biopsy may be able to establish the etiology of noninfectious infiltrates in some patients (131,132). In some cases, surgical lung biopsy will be required to make a definitive diagnosis. The appearance of the BAL and a cell count and differential on the BAL fluid can be helpful in suggesting a diagnosis. A bloody BAL that does not decrease, or increases in the degree of blood return with serial fluid aliquots, is diagnostic of alveolar hemorrhage. This can be confirmed with iron staining that demonstrates hemosiderin-laden alveolar macrophages. BAL fluid that has a milky or whitish, cloudy appearance with flocculent debris that settles to the bottom of the container is suggestive of pulmonary alveolar proteinosis. Additional support for this diagnosis is provided with a positive periodic acid–Schiff (PAS) stain. The diagnosis of pulmonary alveolar proteinosis can be confirmed with transbronchial or surgical lung biopsy. A BAL with a differential count greater than 25% eosinophils is virtually diagnostic of eosinophilic lung disease. In the patient with acute respiratory failure, this finding will most commonly be due to acute eosinophilic pneumonia, although parasitic lung infections such as strongyloidiasis rarely have a similar presentation. A finding of over 25% lymphocytes on BAL differential is suggestive of sarcoidosis, hypersensitivity pneumonitis, drug reaction, or viral infection.
Transbronchial biopsy can confirm the above diagnoses in most cases. In addition, transbronchial biopsy may be able to establish other noninfectious diagnoses of pulmonary infiltrates in the ICU including idiopathic interstitial pneumonia and graft versus host disease (GvHD) in stem cell or bone marrow transplant patients, leukemic infiltrates, drug-induced pneumonitis, bronchiolitis obliterans organizing pneumonia/cryptogenic organizing pneumonia (BOOP/COP), bronchoalveolar carcinoma, lymphangitic carcinomatosis, and acute rejection after lung transplantation.
Traumatic Airway Injury
The classic signs of tracheobronchial disruption include shortness of breath, massive subcutaneous emphysema, persistent pneumothorax despite chest tube insertion, and a large air leak after tube thoracoscopy. On occasion, however, only subtle signs exist, even in the presence of significant injury. Flexible bronchoscopy should be performed early in any patient with chest trauma in whom airway injury may have occurred (133). Signs and symptoms of tracheobronchial injury are listed in Table 38.4. Tracheobronchial disruption rarely occurs as an isolated injury (134); a history of a rapid deceleration injury, such as a motor vehicle accident with the patient’s chest striking the steering wheel or dashboard, is typical. The pathogenesis of tracheobronchial rupture in blunt chest trauma is caused by shearing, wrenching, or compressive forces, acting alone or in concert. Rapid deceleration results in shearing forces, acting predominantly at the distal trachea near the carina where the relatively fixed trachea joins the more mobile distal airways (135,136). If the trachea and mainstem bronchi are crushed between the chest wall and vertebral column and the glottis closed, airway pressure suddenly increases, and resultant rupture of the airway may occur (134).
In patients with tracheal or bronchial disruption, early bronchoscopy can reliably detect the site of airway injury (137–142). Prompt diagnosis and surgical correction of tracheobronchial disruption produces a better outcome, as delay in diagnosis is usually detrimental to the patient (134). Patients with partial tracheal or bronchial disruption may be relatively asymptomatic and present with a paucity of physical findings. Delays in diagnosis unfortunately are common and have been associated with decreased frequency of successful repair. Failure to diagnose disruption may result in a delayed stricture formation at the site of injury, resulting in dyspnea, distal atelectasis, and chronic recurrent infections.
TABLE 38.4 Signs and Symptoms of Tracheobronchial Injury |
![]() |
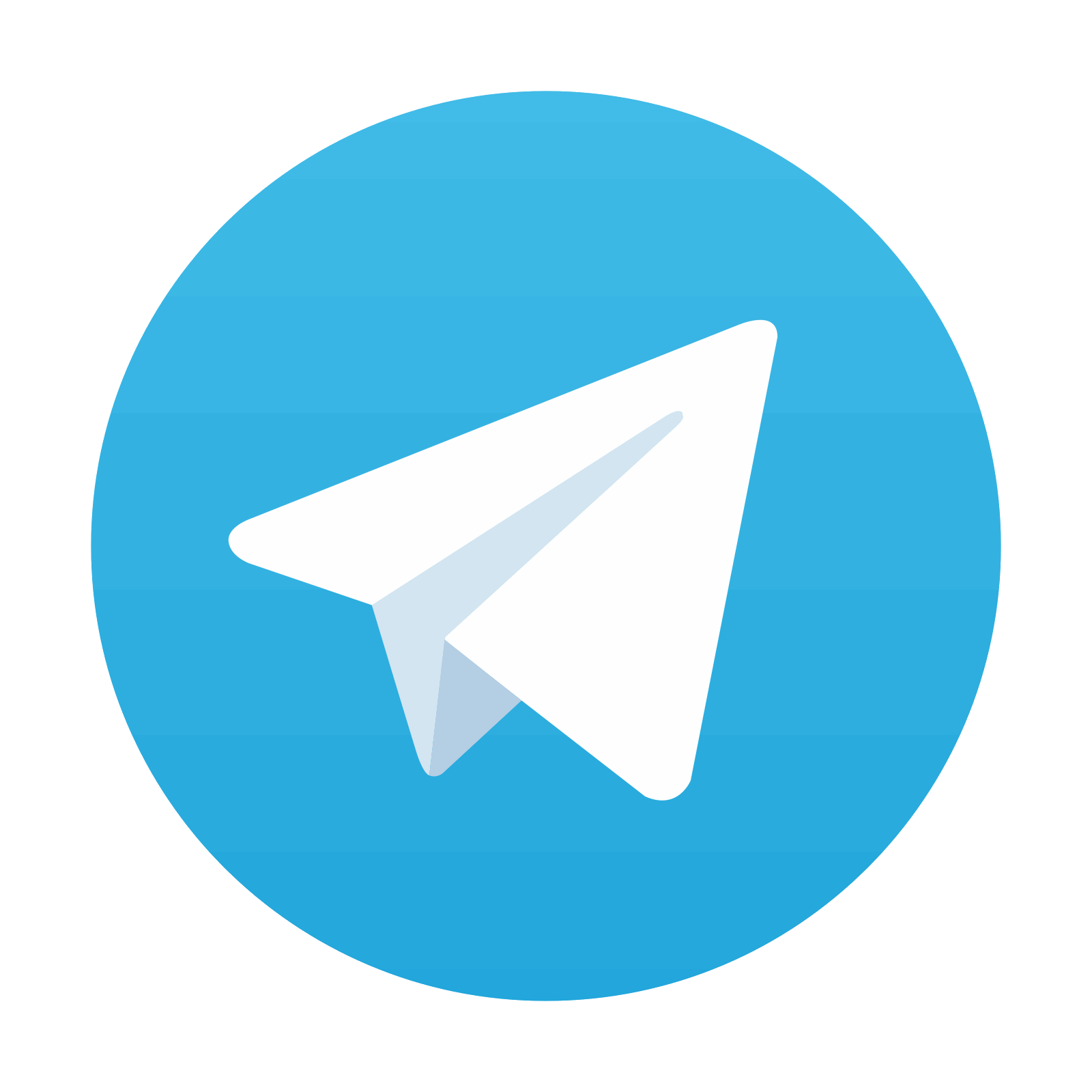
Stay updated, free articles. Join our Telegram channel

Full access? Get Clinical Tree
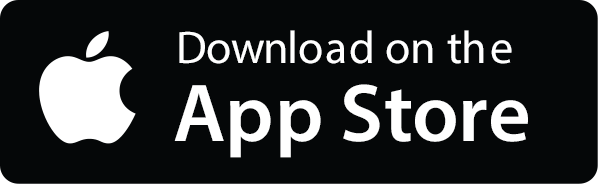
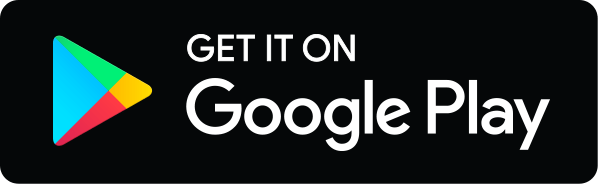