14.1.2 Indications for FFP Use in Critical Illness
The use of FFP in critical illness has increased in recent years, although the evidence base supporting its clinical effectiveness is surprisingly lacking. Recommen-dations in national guidelines [3] are largely based on expert opinion rather than conclusions from well-conducted, randomised trials. Accepted potential indications in critically ill patients include the following:
1.
Replacement of multiple coagulation factor deficiencies associated with severe bleeding, disseminated intravascular coagulation and acute traumatic coagulopathy. The treatment of major haemorrhage, in which FFP is administered as part of a protocolised response to massive blood loss, is dealt with in a separate chapter.
2.
Correction of coagulopathy in non-bleeding patients.
3.
Prophylaxis prior to invasive procedural instrumentation (e.g. central line insertion) in coagulopathic patients.
4.
Specific indications, e.g. plasma exchange in thrombotic thrombocytopenic purpura and Guillain-Barre syndrome, treatment of C1-esterase inhibitor deficiency.
The use of FFP for prophylactic correction of coagulopathy prior to procedures is discussed in more detail below.
14.1.3 Situations in Which FFP Transfusion Is Inappropriate
1.
Reversal of warfarin anticoagulation. In the absence of bleeding, excessive anticoagulation as a result of warfarin administration should be reversed with intravenous or oral vitamin K. In the presence of bleeding and a prolonged INR in a patient taking warfarin, prothrombin complex concentrate is recommended rather than FFP, which is only partially effective in reversing over-warfarinisation.
2.
Correction of single-factor deficiencies. In this situation, single-factor concentrate is available and should be used.
3.
Volume expansion. There is no evidence to support the routine use of FFP as a colloid solution, and the risk/benefit balance and cost-effectiveness have never been explored in adequately powered randomised controlled trials.
In addition, FFP is absolutely contraindicated in congenital IgA deficiency in the presence of anti-IgA antibodies and relatively contraindicated in pulmonary oedema.
14.1.4 FFP Use During Critical Illness
Observational studies indicate that approximately 30 % of critically ill patients experience an episode of INR prolongation, although in the majority of cases (70–75 %) the worst INR is less than 2.5, and abnormalities are limited to a single abnormal test result [1, 7]. Despite this, 30 % of episodes of prolonged INR were associated with FFP prescription (10–15 % of all ICU admissions). Typically, 50 % of FFP prescriptions are given to non-bleeding patients, of which half are administered prior to a procedure and half to treat coagulopathy despite no procedure. Observational studies show wide variation in practice, and in response to surveys, clinicians indicate wide variation in beliefs about use of FFP in the absence of bleeding. Clinicians appear more likely to administer pre-procedural FFP when patients have liver disease and other coagulation abnormalities (low platelets; prolonged APTT) or are receiving concurrent red cell transfusions [8, 9].
Observational studies indicate wide variation in FFP dose between clinicians. Concurrent bleeding is associated with higher clinical doses. However, many clinicians prescribe smaller doses than recommended in current guidelines (see below).
14.2 Tests to Assess the Risk of Bleeding
The most commonly used laboratory assay of coagulation factor activity is the prothrombin time (PT). This provides a measure of the extrinsic pathway of coagulation and represents the time taken for plasma to clot after the addition of tissue factor (Factor III). The PT may be standardised to calculate a prothrombin ratio or international normalised ratio (INR). PT ratio is calculated as PT ratio = PT/MNPT and INR as INR = (PT/MNPT)ISI, in which MNPT and ISI are the local, laboratory-specific mean normal PT and international specificity index, respectively. Calculating INR therefore provides an adjustment for different thromboplastin sensitivities between different laboratories.
Despite a lack of robust evidence, an INR of greater than 1.5 is frequently recommended as the threshold for considering FFP transfusion and is present in most guidelines. This cut-off is associated with impending haemostatic failure and represents a fall in the activity of some coagulation factors to less than 50 % of normal. As can be seen from Fig. 14.2, there is significant functional reserve in normal coagulation factor levels, and even at an INR of 1.5, there may be normal haemostasis as measured by concentrations of individual factor levels. Significant bleeding risk is thought to be increased only when factor levels are less than 30 % of normal ranges. However, this number relates to a single-factor deficiency and may not hold for conditions characterised by multiple factor deficiencies.
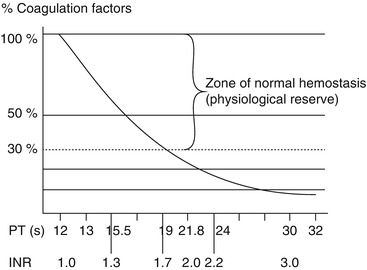
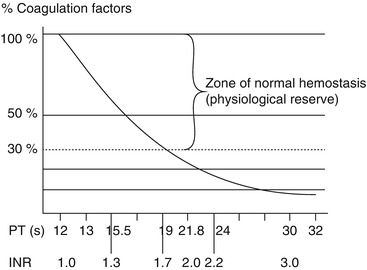
Fig. 14.2
Relationship between coagulation factor concentration and PR/INR (Taken from Yazer et al. [4])
There are several well-recognised disadvantages to making FFP transfusion decisions based on PT or INR. In vitro laboratory coagulation tests poorly reflect the complex in vivo haemostatic milieu [5], and there are relatively few studies that support a link between a prolonged PT/INR and bleeding [6]. The long lead time between drawing a sample from a patient to receiving the INR test result (typically in the order of 45 min in many hospitals) means that in a rapidly changing clinical situation, the INR result no longer reflects the patient’s current haemostatic status by the time the result is available. Transfusion of FFP in coagulopathic patients, especially those without bleeding, typically results in no change or only a modest improvement in INR when currently recommended doses of 10–15 mL/kg are administered. For example, an 11 mL/kg transfusion of FFP in adults reduced median INR by only 0.2 [7]. Observational data indicates that correction of INR (or equivalent) rarely occurs when the INR is in the 1.5–2.5 range; larger corrections are typically observed at progressively greater derangements. However, correction is typically short lived and limited to less than 24 h.
As the use of point of care, whole-blood viscoelastic tests of coagulation (e.g. ROTEM®/TEG®) increases, it may be possible to recommend thresholds for FFP transfusion based on the results of these technologies. By providing a faster turnaround and allowing the quantification of the interaction between coagulation factors, platelets and red cells in whole blood (rather than utilising plasma only, as with PT and APTT), these tests have several attractive advantages. It is now commonplace to make blood product transfusion decisions in the resuscitation of major trauma based on viscoelastic ROTEM®/TEG® results. There is as yet limited evidence for translating this to non-bleeding patients in intensive care units.
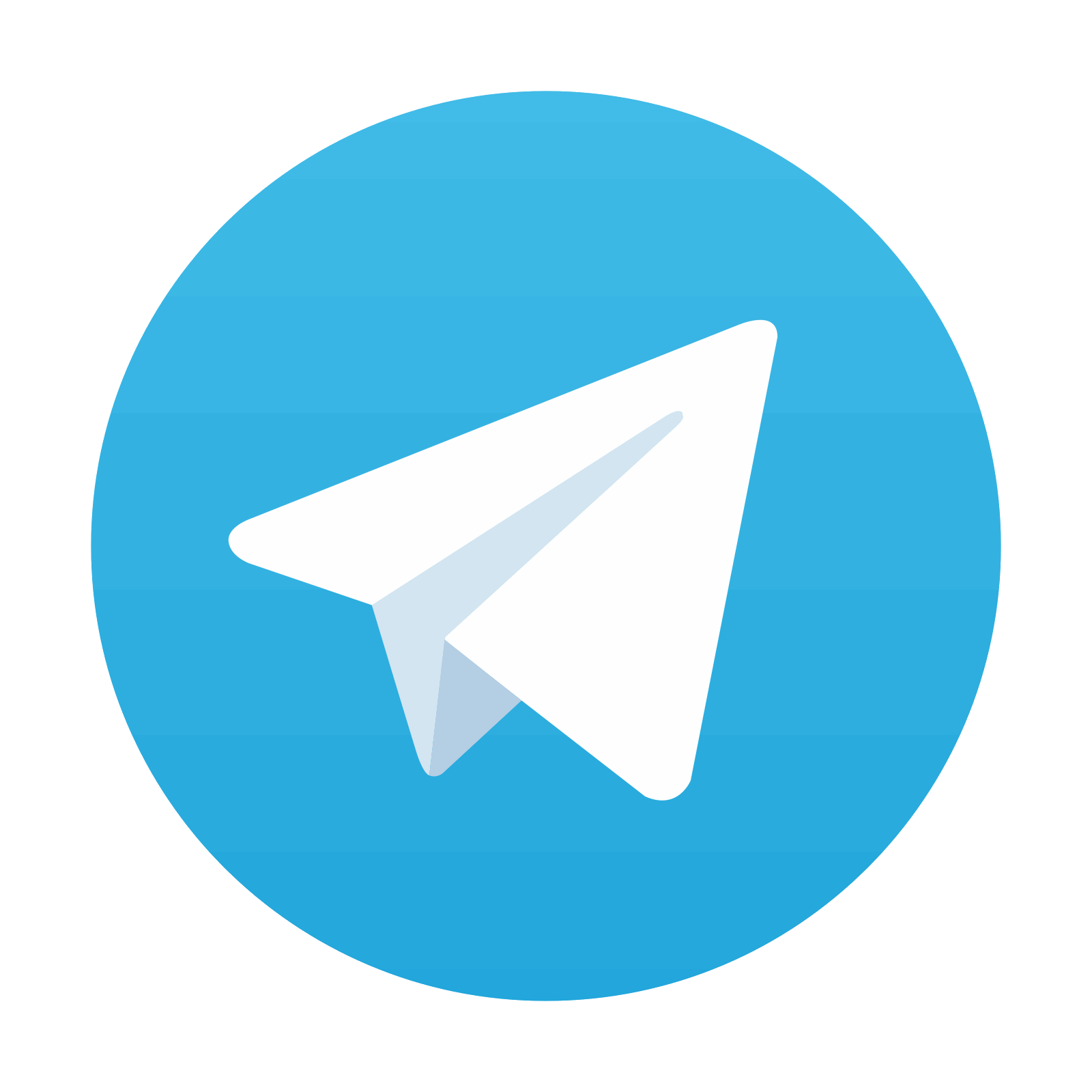
Stay updated, free articles. Join our Telegram channel

Full access? Get Clinical Tree
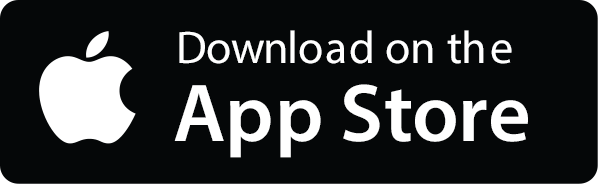
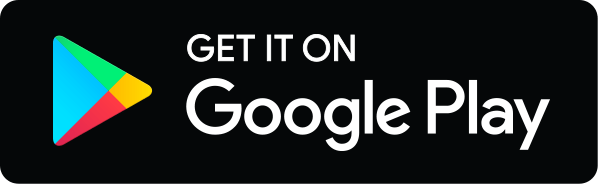