Abstract
Critical illness during pregnancy poses significant challenges driven by complex interactions between physiological changes, pre-existing conditions, and healthcare disparities. In high-income countries, increasing maternal age and comorbidities complicate obstetric care by triggering an unprecedented rise in cardiac disease during pregnancy, while infections like influenza and COVID-19 are important causes of maternal adult respiratory distress syndrome. Extracorporeal membrane oxygenation (ECMO) gained prominence as a vital intervention, providing respiratory and/or cardiac support, for varying indications between antenatal and postpartum periods. The physiological changes of pregnancy demand close attention and adjustment of ECMO parameters, along with multidisciplinary collaboration. While potential complications such as bleeding and thromboembolism require vigilant management and optimal management of ECMO support in pregnancy still needs to be elucidated, ECMO nevertheless yields favorable maternal and fetal outcomes.
1
Critical illness during pregnancy
Severe maternal morbidity and mortality are important concerns in obstetric care, reflecting the complexity of pregnancy-related physiologic changes and their interplay with pre-existing conditions, socioeconomic considerations, and healthcare system disparities [ ]. The global landscape of maternal health demonstrates a dichotomous progression, with ongoing reductions in maternal mortality among some regions, while other regions may see stagnation or even worsening outcomes [ ]. In high-income countries, trends in maternal mortality are driven at least in part by rising maternal age as well as a higher degree of maternal comorbidity (e.g., hypertensive disorders of pregnancy, diabetes, obesity) [ , ]. Coupled with the physiological demands of pregnancy, these comorbid conditions predispose patients to a spectrum of severe illness. Ranging from severe pre-eclampsia and eclampsia to acute cardiac events and sepsis, such conditions necessitate advanced critical care interventions [ ].
Estimating the incidence of maternal critical illness or mortality is challenging, owing to heterogeneity in definitions, reporting practices, and healthcare infrastructure across different geographical regions [ ]. Nonetheless, present estimates indicate that the challenge is significant. The incidence of severe maternal morbidity is approximately 2.5% in the US and UK [ ], while obstetric critical illness accounts for 12.1% of all intensive care unit (ICU) admissions among women aged 16–50 years in the UK [ ]. The leading causes for obstetric ICU admission are hypertensive disorders of pregnancy, hemorrhage, sepsis, and thromboembolic conditions [ , ]. In maternal critical illness, the timely and effective utilization of extracorporeal membrane oxygenation (ECMO) may serve as a crucial intervention to prevent “failure to rescue,” the inability to prevent death following a severe complication. By providing respiratory and/or cardiac support, ECMO serves as a bridge to recovery or stabilization, and eventual resolution of underlying pathology or definitive management.
The past 20 years have seen a rapid rise in the utilization of ECMO for pregnant patients. As demonstrated in a systematic review of 358 patients supported by ECMO during pregnancy until 2019, there were only 21 case reports and case series published from 1974 until 2004 [ ]. Subsequently, the number of publications rose precipitously – in the last 5 years studied in the systematic review, there were over 100 reports published.
The indications for initiating ECMO during pregnancy and in the postpartum period highlight the dynamic and evolving nature of maternal critical care needs. The spectrum of conditions requiring ECMO support varies significantly between the antenatal and postpartum phases, and reflect the unique physiological and clinical challenges encountered during these distinct stages of maternity care [ ]. In the antenatal period, acute respiratory distress syndrome (ARDS), cardiac failure, and cardiac arrest account for the majority of cases. In the immediate postpartum period within the first 24 h, cardiac arrest, cardiac failure, and amniotic fluid embolism become the predominant indications for ECMO. The immediate postpartum phase is a particularly critical period where acute cardiac events may be precipitated by the interplay of physiological changes of pregnancy with the stresses of labor and delivery [ ]. Beyond 24 h postpartum, ARDS, peripartum cardiomyopathy, and cardiac failure rise to the forefront as leading indications for ECMO.
2
Extracorporeal membrane oxygenation (ECMO)
The ECMO circuit comprises several essential components ( Table 1 ; Fig. 1 ) [ ]. A variety of parameters require regular assessment and adjustment to optimize ECMO support while mitigating complications ( Table 2 ); adaptations for pregnant patients are summarized in Table 3 . The two primary modalities of ECMO in common practice, veno-venous ECMO (VV-ECMO) and veno-arterial ECMO (VA-ECMO) will be discussed below.
Component | Description | |
---|---|---|
Cannulas | Drainage cannula | Inserted into a large vein, such as the right internal jugular or femoral vein, to withdraw deoxygenated blood from the patient. |
Return cannula | Positioned typically in the superior vena cava in VV-ECMO, or in the femoral artery or axillary artery in VA-ECMO, to reintroduce oxygenated blood back to the patient. | |
Blood pump | Ensures continuous blood flow throughout the ECMO circuit. Typically, centrifugal pumps are favored to minimize blood trauma. | |
Ultrasonic flow probe | Placed around the return cannula, measures actual blood flow and detects microbubbles (via an implemented bubble detector). | |
Oxygenator | Facilitates gas exchange by infusing oxygen into the blood and removing carbon dioxide. Comprises a semipermeable membrane enabling gas exchange between the patient’s blood and the “sweep” gas. | |
Heat exchanger | Regulates blood temperature before it returns to the patient, promoting normothermia. | |
Tubing | Connects the various elements of the ECMO circuit. Typically constructed from biocompatible materials, and often heparin-coated, designed to facilitate blood flow without causing damage or clotting. | |
Pressure sensors | Strategically placed within the circuit to monitor inline pressure, aiding in maintaining proper flow and preventing complications such as clot formation or air embolism. | |
Gas flow control | Manages the flow of oxygen and other gases into the oxygenator, enabling adjustments to the oxygen concentration delivered to the patient and facilitating CO 2 removal. |

Parameter | Description | |
---|---|---|
Flow rate | Quantifies the volume of blood circulating through the ECMO circuit per unit of time, typically measured in liters per minute (L/min). Modulating flow rate is critical for maintaining adequate perfusion and oxygenation. Flow can be automatically stopped upon detection of bubbles. | |
Sweep gas | Oxygen concentration | Determines oxygen delivery to the oxygenator, regulating blood oxygen partial pressure. |
Flow rate | Influences CO 2 removal efficiency. | |
Temperature | Crucial for maintaining normothermia. Target temperature typically 37° Celsius. | |
Hemoglobin and hematocrit levels | Aids in assessing oxygen-carrying capacity. ECMO adjustments may optimize oxygen delivery, employing a “goal-directed ECMO” approach. | |
Circuit pressures | Vital to mitigate excessive stress on blood cells. Monitoring helps to manage circuit- or patient-related complications (e.g., hypovolemia, tamponade, thrombi, tubing kinks, cannula position, etc.) | |
Oxygen saturation | Arterial and venous | Evaluates oxygenation and perfusion adequacy. |
Carbon dioxide levels | Reflects efficiency of CO 2 removal, crucial for optimizing respiratory support. | |
pH | Assesses acid-base balance, guiding adjustments in sweep gas flow rate or other parameters to maintain physiological pH levels. | |
Anticoagulation | Monitoring necessary to balance risks of circuit thrombosis with hemorrhage. Typically achieved with intravenous heparin targeting anti Xa levels of 0.2–0.5 IU/mL, ACT of 160–220s, or aPTT of 45–80 s. |
Parameter | Goals |
---|---|
ECMO flow rate | Initial ECMO flow between 5 and 6 L/m 2 /min, to accommodate increased cardiac output. |
PaO 2 | Target >70 mmHg. |
PaCO 2 | Target between 28 and 32 mmHg, caution with rapid correction of hypercarbia. |
pH | Maintain >7.40. |
Cannulation | Exercise care not to increase venous back pressure, which may decrease uterine blood flow. |
Positioning | Maintain left uterine displacement when supine. Proning may be beneficial in ARDS, |
Fetal monitoring | Consider starting around 24 weeks gestation. Can be performed intermittently or continuously, though latter approach requires presence of skilled personnel and resources in the event of emergencies (which may require bedside cesarean delivery). |
In cases of acute respiratory failure, VV-ECMO is commonly initiated to improve oxygenation and address hypercapnia refractory to conventional gas exchange interventions (e.g., non-invasive ventilation, mechanical ventilation). Serving as a transient respiratory support, VV-ECMO mitigates the risks associated with ventilator-induced lung injury and predominantly serves as a bridge-to-recovery intervention. A critical step before initiating VV-ECMO is to thoroughly evaluate the reversibility of the precipitating condition. It is also noteworthy that VV-ECMO does not provide active circulatory support, thus it is imperative for patients undergoing VV-ECMO to possess normal cardiac function, with or without inotropic support. The implementation of VV-ECMO can be accomplished through various cannulation strategies. In the prevalent approach, deoxygenated blood is actively withdrawn by a centrifugal pump through a femoral vein outflow cannula. Subsequently, after traversing an oxygenator, oxygenated blood is reintroduced into the right atrium via a return cannula. Alternatively, a dual-lumen cannula may instead be inserted through the internal jugular vein, allowing for the drainage of deoxygenated blood from superior and inferior cava, followed by the administration of oxygenated blood through a return flow spout in the right atrium.
In contrast to VV-ECMO, VA-ECMO not only furnishes respiratory assistance but also delivers comprehensive mechanical circulatory support. The size of the cannulas and capacity of the oxygenator determine the achievable flow rates, with a theoretical maximum blood flow up to 10 L/min. The versatility of VA-ECMO allows for it to be used as a bridge-to-recovery, bridge-to-decision, or bridge-to-transplant strategy in patients with acute heart failure refractory to medical therapy. In VA-ECMO, the venous cannula typically accesses the femoral vein to withdraw deoxygenated blood from the patient, while the arterial cannula is inserted into either the femoral or axillary artery, facilitating the return of oxygenated blood to the patient’s circulatory system.
3
Physiologic changes of pregnancy
The physiological changes of pregnancy require special attention and appropriate adjustment of ECMO parameters [ ]. Paramount to the management of this unique population is the recognition of the physiologic needs of the fetus and its dependence on uteroplacental function.
Cardiac output increases by 30–40% during pregnancy, and the maximum increase is attained around 24 weeks’ gestation. Maternal heart rate increases by the end of the first month of pregnancy and plateaus at an increase of 10–15 beats per minute by 28–32 weeks’ gestation. Stroke volume increases by mid-first trimester and progressively increases through the second trimester. Accordingly, increased ejection fraction is also demonstrated on echocardiography. Filling pressures (i.e., central venous pressure, pulmonary capillary wedge pressure, left ventricular end-diastolic pressure) do not change despite the increased cardiac dimensions, due to myocardial remodeling during gestation. Blood pressure rarely increases in uncomplicated pregnancy, instead systolic and diastolic blood pressures decrease by approximately 8% and 20% at 20 weeks’ gestation and then gradually return to baseline through the third trimester. Systemic vascular resistance decreases by approximately 20% due to the low resistance uteroplacental vascular bed as well as hormonal modulation of maternal vascular tone. Immediately postpartum, there is an acute albeit transient increase in maternal circulating volume (i.e., “autotransfusion”) from sustained myometrial contraction, removal of the low-resistance placental circulation, and relief of inferior vena cava obstruction.
Metabolic requirements are greater in pregnancy. As a consequence, oxygen consumption and carbon dioxide production are increased as pregnancy progresses. Minute ventilation increases by 50%, caused by increases in both tidal volume (40%) and respiratory rate (15%). Enhanced ventilation results in the decrease of PaCO 2 to 30–32 mmHg. However, the pH remains in normal range at 7.4 with compensatory metabolic acidosis from enhanced renal excretion of bicarbonate. Lower basal levels of a serum bicarbonate buffer during pregnancy may result in an exaggerated metabolic acidosis if a parturient develops diabetic ketoacidosis or sepsis. Functional residual capacity, expiratory reserve volume and residual volume are decreased as pregnancy progresses. Total lung capacity remains within normal range. Increased renal blood flow during pregnancy increases glomerular filtration fraction, thereby decreasing serum urea (8–9 mg/dL; 1.3–1.5 mmol/L) and creatinine (0.5–0.6 mg/dL; 44–54 mmol/L).
Hematological adaptations in pregnancy result in hypercoagulability. Levels of clotting factors I (fibrinogen), VII, VIII, IX, X, and XII are elevated during pregnancy. Platelet production and thrombopoietin levels are increased, and platelet aggregation measured in vitro is likewise increased. However, indices of platelet destruction also increase in pregnancy. Therefore, the overall effect of these changes is variable. Prospective observations have reported a statistically significant fall in platelet count as pregnancy progresses, with 7.6% of parturients having a count less than 150,000 and 1% less than 100,000 at term gestation [ ]. Endogenous anticoagulants, such as protein S, are decreased in normal pregnancy and there is acquired resistance to activated protein C during pregnancy, enhancing the prothrombotic state. Fibrinolysis is impaired in normal pregnancy due to placentally derived plasminogen activator inhibitor (PAI) but returns to normal following delivery of the placenta. Overall indices of coagulation such as thromboelastography indicate that normal pregnancy is a hypercoagulable state, beginning in the first trimester, increasingly so in labor, and returning to normal approximately two weeks postpartum [ ].
4
ECMO during pregnancy
4.1
Organizational considerations
When considering ECMO therapy in patients with potentially viable fetuses, an experienced multidisciplinary team approach involving obstetricians, maternal fetal medicine specialists, neonatologists, anesthesiologists, intensivists, cardiothoracic surgeons, cardiologists, perfusionists, pharmacists, and specialized critical care and obstetrics nurses is crucial. Support from additional medical and surgical specialists may be needed. Where the outcomes of ECMO support as a salvage therapy depend on timely initiation, early transfer to a specialized ECMO center should be considered in critically ill parturients not responding to conventional management.
4.2
Delivery planning
Decisions regarding ECMO initiation and the timing of delivery must be carefully weighed. There must be well-defined contingency plans should the need arise for urgent or emergent delivery, with appropriate staffing coverage and equipment to expeditiously perform cesarean delivery at the bedside if needed.
When evaluating a pregnant patient for ECMO support, initial discussion might default to a plan for urgent cesarean delivery immediately preceding ECMO initiation. However, the Society for Maternal-Fetal Medicine guidelines suggest that ECMO support itself is not a specific indication for delivery – rather, standard maternal and fetal indications should primarily determine whether delivery should precede ECMO initiation [ ]. In general, if there are no pressing indications for immediate delivery, then it is not necessary to delay starting ECMO support.
Once ECMO support is started and maternal cardiopulmonary derangements are initially stabilized, it may be desirable to further maintain the pregnancy in order to reduce morbidity related to neonatal prematurity. A recent systematic review attests to the feasibility of prolonging pregnancy for days to weeks after initiation of ECMO [ ]. There are scant data to suggest an optimal gestational age to target before planned elective delivery, though 28–32 weeks’ gestation have been previously suggested as a reasonable timeframe in this context [ ]. Should premature delivery be deemed a potential scenario, the administration of corticosteroids to stimulate fetal lung maturation warrants consideration. The mode of delivery must also be considered carefully. In the majority of cases cesarean delivery has been preferred [ ], perhaps due to greater control over delivery timing. However, it is a major surgery imparting maternal inflammatory burden and possible complications such as bleeding or infection. There are reports of vaginal or instrumented delivery during ECMO support, though this presents unique challenges [ , ]. For instance, the required positioning for this means of delivery may cause ECMO cannula displacement or kinking. ECMO flows could be also reduced during periods of Valsalva. In the absence of definitive outcome data by mode of delivery, case-by-case decision-making by the multidisciplinary team will need to include consideration of prior obstetric history, absolute contraindications to vaginal delivery, patient tolerance of interrupted ECMO flow, risk factors for obstetric bleeding or thrombophilia, among other concerns.
Regardless of the timing or method of delivery, there may be significant maternal bleeding owing to the degree of anticoagulation commonly employed during ECMO support and vigilance for uterine atony remains essential when evaluating postpartum hemorrhage in this setting. Mitigating the risk of uterine atony can be achieved by avoidance of volatile anesthetics and the administration of appropriate uterotonics, although the latter may carry adverse cardiovascular effects (e.g., increased afterload with ergot alkaloids) [ ]. Following cesarean delivery, meticulous closure of the uterine incision – and occasionally abdominal packing – are imperative. Throughout their postpartum course, regular assessment of uterine hemostasis is warranted. In instances of hemodynamic instability, postpartum uterine bleeding should be incorporated into the differential diagnosis for prompt intervention.
4.3
Fetal monitoring
Fetal monitoring may provide valuable warning about reversible causes of fetal distress. Additionally, fetal heart rate may represent a “fifth vital sign” for maternal well-being, as an early marker of significant maternal physiologic deterioration [ ]. Fetal monitoring can be performed intermittently by an obstetrician using Doppler ultrasonography of umbilical arteries and the fetal heart. Alternatively, continuous cardiotocography can be employed, but this approach necessitates the continuous presence of personnel (i.e., midwifes, obstetric nurses, obstetricians) proficient in the interpretation of this technique; moreover, adequate resources for emergency cesarean delivery at the bedside would be required, should persistent fetal heart rate abnormalities be noted. Some causes of fetal heart rate abnormalities during ECMO support may include uterine contractions, obstruction of uterine venous drainage secondary to inferior vena cava cannulation, hypothermia, administration of anesthetics and/or opioids, hemodilution, compromised uteroplacental blood flow, and diminished systemic vascular resistance or arterial hypotension.
4.4
Aortocaval compression
Beyond 20 weeks’ gestation, supine positioning may result in aortocaval compression by the gravid uterus and compromise maternal venous return as well as uteroplacental blood flow [ ]. A cushion placed under the right hip may provide appropriate left uterine displacement to prevent this.
4.5
Uteroplacental function
Uterine blood flow is poorly autoregulated and depends on maintaining a uterine perfusion pressure greater than uterine vascular resistance [ ]. Consequently, reductions in maternal blood pressure can precipitate fetal bradycardia and hypoxia. Other factors may threaten uteroplacental blood flow and fetal oxygenation during ECMO therapy, including uterine contractions, acidosis, arterial hypotension, systemic inflammation, embolism, and obstruction of uterine venous drainage following inferior vena cava cannulation [ ]. Recommended strategies to uphold adequate uteroplacental blood flow include maintaining a high pump flow rate (>2.5 L/min/m 2 ) and elevated perfusion pressures (>70 mmHg). Sympathomimetic agents such as phenylephrine and norepinephrine are deemed safe during pregnancy to sustain perfusion pressure [ , ]. While vasopressors may induce uterine vascular resistance via α-adrenergic receptor stimulation, this effect is counteracted by concurrent enhancements in maternal cardiac output and systemic blood pressure.
It is also important to ensure maternal hematocrit levels remain above 25%–28% to optimize oxygen-carrying capacity and fetal oxygenation. Hemodilution may risk fetal well-being by exacerbating physiological anemia and reducing placental blood oxygen content. Recommended strategies for managing ECMO in pregnancy encompass optimizing maternal oxygen-carrying capacity, mitigating hypothermia, favouring pulsatile flow, and tolerating slight hypercapnia while maintaining a normal pH through alpha-stat management [ , ]. Non-pulsatile flow patterns may lead to heightened placental vascular resistance and diminished placental perfusion. Additionally, hypothermic perfusion has been associated with uterine contractions, underscoring the importance of meticulous temperature management during ECMO therapy in pregnant patients [ ].
Progesterone plays a pivotal role in uterine relaxation [ ]. Dilution of maternal blood volume with the priming volume of the ECMO circuit could potentially diminish circulating progesterone levels and lead to increased uterine contractions. In the event of undesirable contractility, tocolytic therapy should be promptly initiated with obstetric guidance to mitigate uterine activity and prevent adverse obstetric sequelae. A comprehensive exploration of available tocolytic agents is outside of the scope of this review [ ]. Current evidence remains limited regarding the superiority of one tocolytic agent over another. Therefore adherence to local and institutional protocols for choice of tocolysis in this context is reasonable For hemodynamically unstable patients, the cardiovascular side effects of certain tocolytics such as nifedipine and beta-2-sympathomimetic agents must be recognized [ ]. Additionally, it is imperative to ensure elevated perfusion pressure during uterine contractions to maintain adequate placental perfusion.
4.6
Mechanical ventilation
Lung protective ventilation strategies as outlined by the ARDS network are commonly used for patients with ARDS [ ]. Some hallmark characteristics of lung protective ventilation are reduced tidal volumes (4–8 mL/kg) and maximum plateau pressures (less than 30 cmH2O), and the resultant hypercarbia is typically considered permissible. These ventilation strategies are also employed during pregnancy despite a lack of validation in this population [ ]. In pregnancy, increased intrabdominal pressure from the gravid uterus makes it difficult to avoid higher airway pressures during mechanical ventilation, and prolonged hypercarbia is controversial due to concerns about the effects of sustained fetal acidosis.
It has traditionally been acceptable to accept hypercarbia up to 50 mmHg for the mechanically ventilated parturient [ , ]; such a threshold was extrapolated from a classic study by Ivankovic et al., observing the impact of hypercarbia among healthy patients undergoing vaginal assisted delivery with methoxyflurane anesthesia [ ]. Some patients were assigned to have iatrogenically induced transient (<10 min) hypercarbia up to 57 mmHg and their neonates had higher Apgar scores than those born to the control group – this effect was attributed to hypercarbia causing higher cardiac output, increased uteroplacental blood flow, uterine relaxation, and a leftward shift of the oxyhemoglobin dissociation curve. However, this seminal study’s conclusions may not apply to critically ill parturients with infection, prolonged hypercarbia, and concomitantly administered drugs (e.g., fentanyl, midazolam, and propofol) which often facilitate mechanical ventilation but impact neonatal outcomes [ ].
A more recent study of delivery during maternal mechanical ventilation or ECMO support has reported that maternal hypercarbia >45 mmHg at delivery is indeed associated with lower 1- and 5-min Apgar scores (<3 at 1 min and <5 at 5 min). It is difficult to separate the effects on the neonatal outcome due to elevated maternal and fetal PCO 2 compared to the impact of concomitant factors in critical illness . While the precise relationship between lower Apgar scores and higher maternal PaCO 2 ranges may be subject to debate, neonatal depression must be anticipated when maternal PaCO 2 is elevated. Accordingly, when delivery is imminent in a mechanically ventilated critically ill parturient with or without ECMO, the presence of maternal hypercarbia represents a need to prepare for neonatal resuscitation.
Prone positioning may improve oxygenation and survival for patients with ARDS and refractory hypoxemia [ ]. Although logistically challenging, prone positioning may be safely performed during ECMO in pregnancy. Previous reports have described the feasibility of an 8-h supine and 16-h prone protocol in parturients while maintaining continuous fetal heart monitoring [ , , ].
4.7
Cannulation
In theory, aortocaval compression may impede femoral guidewire or cannula advancement, but in practice compression of the inferior vena cava does not appear to significantly impede femoral cannulation [ , ]. Along with aortocaval compression, femoral vein cannulation may increase venous back pressure within the uterine vasculature and impede utero placental perfusion. As such, continuous fetal heart rate monitoring is informative during cannulation. It is possible that persistent fetal bradycardia during cannulation may require emergent cesarean delivery in the intensive care unit [ ]. Sometimes, it may be necessary to use smaller gauge ECMO cannulas. Alternatively, a dual-lumen bicaval cannula placed via the right internal jugular vein and circumvents the above complications associated with femoral cannulation [ ]. Placement of an additional venous drainage cannula may also be considered.
4.8
ECMO flow rates
During VV-ECMO, ECMO flows must be adjusted to maintain adequate oxygenation, usually considered an arterial saturation greater than 80% in non-pregnant adult patients [ ]. However, this level of relative hypoxemia is unacceptable during pregnancy. A maternal PaO 2 of 60 mmHg (i.e., oxygen saturation greater than 90%) must be maintained for adequate oxygenation to the fetus [ ]. Recurrent fetal decelerations or other abnormalities on the fetal heart rate tracing may indicate the need to augment maternal oxygen saturation via increased VV-ECMO flow [ ].
Hypercapnia may result in fetal acidosis. Because a maternal PaCO 2 of 30–32 mmHg during pregnancy represents normocarbia during pregnancy, the sweep flow is increased to target this ideal PaCO 2 during ECMO. However, rapid correction of PaCO 2 exceeding 50% within the first 24 h of ECMO initiation may confer a risk of maternal neurologic complications so slow titration is warranted [ ].
4.9
Fetal acid-base status
Intensivists must take into consideration the acid base status of the fetus while managing critically ill parturients. Under normal circumstances, fetal blood gas measurements closely parallel maternal blood gases, so fetal acid-base status may be indirectly estimated from maternal blood sampling. In healthy parturients, the fetal–maternal PCO 2 gradient during the first stage of labor is 8.8 mmHg, increasing to 15.3 mmHg during the second stage [ ]; furthermore, this difference is accentuated with hyperventilation and decreased during hypercarbia. While the relationship between maternal and fetal blood gas measurements in healthy parturients undergoing vaginal and cesarean delivery was already elucidated in the 1960s, this relationship was not established in critically ill pregnant patients until very recently [ ]. During severe ARDS, the fetal-maternal PCO 2 difference is exaggerated to 17.5 mmHg, due to prolonged hypercarbia and other compounding factors such as infection and concomitant use of sedation medications. Regardless, the fetal blood gas PCO 2 remains correlated with maternal PCO 2 values during critical illness.
4.10
Anticoagulation
Unfractionated heparin (UFH) is the most used systemic anticoagulant during ECMO [ , , ].UFH is widely available, inexpensive, readily reversible, and familiar to acute care clinicians. Additionally, UFH does not traverse the placenta and poses no teratogenic risks to the developing fetus at standard doses used in clinical practice. Typically, UFH is administered as a bolus (50–100 units per kilogram) at the time of cannulation, followed by a continuous infusion titrated according to laboratory testing and clinical evaluation. Pregnancy is a prothrombotic state; hence, vigilance is needed to detect clot formation within the ECMO circuit.
When a cesarean delivery is performed either immediately preceding ECMO initiation or during ECMO support, there is an escalated risk of obstetric hemorrhagic complications. It is vital to recognize that while heparin administration heightens the risk of bleeding, obstetric complications themselves pose a more substantial threat of extensive hemorrhage than heparin therapy which can be antagonized if necessary. Prophylactic administration of antifibrinolytic agents such as tranexamic acid for cesarean delivery is controversial [ , ], with potential concerns about thrombosis given the hypercoagulability associated with pregnancy [ ]. In general, the administration of tranexamic acid does aid in hemostatic control for patients receiving ECMO support [ ]. Overall, there is a lack of consensus regarding the optimal anticoagulation strategy for ECMO patients during pregnancy, wherein bleeding remains the predominant complication. Monitoring of anticoagulation is preferably achieved using anti-Xa levels ( Table 2 ).
5
Complications
Maternal survival after ECMO is very favorable, with 75% of patients surviving at 30 days and 74% at one year [ ].This is particularly striking compared to the general population, where registry data have demonstrated survival rates of 44% and 57% for respiratory and cardiac indications, respectively [ ]. Moreover, pregnant patients receiving ECMO post-cardiac arrest (also known as extracorporeal cardiopulmonary resuscitation; ECPR) have a survival rate of 88% compared to a 59% population-based cardiac arrest survival rate, suggesting that ECMO may be particularly advantageous in this setting [ ].
Fetal survival after maternal ECMO support is 65%–84%, a remarkable figure given the significant physiologic toll that maternal critical illness can exert on a fetus [ , ]. It is worth noting that neonatal complications associated with ECMO support include preterm birth, neonatal intensive care unit admission, and neurologic injury [ ]. There remains a paucity of longer-term outcome data for neonates delivered during or after maternal ECMO support.
In general, ECMO-related complication rates for parturients tend to be similar to the general population. Common complications include hemorrhage, vascular injury, neurologic complications, and thromboembolism [ , ]. Given the common use of anticoagulation during ECMO support, bleeding is a common complication (37%), and may arise from numerous potential sources [ , ]. Localized external bleeding may benefit from topical hemostatic agents such as oxidized cellulose or kaolin-impregnated gauze [ ]. Additionally, mechanical hemostasis must be attempted by any means possible. For uncontrolled hemorrhage, temporary suspension of systemic anticoagulation or even full reversal of anticoagulation may be considered, though this must be balanced against the possibility of ECMO circuit thrombosis. In such a scenario, it is essential to monitor for visible clot formation within the circuit and be prepared to more frequently change the oxygenator and other components. When there is unacceptably high risk of major bleeding, ongoing maintenance of ECMO support with minimal or no anticoagulation may be justifiable, particularly when employing heparin-coated systems [ ].
6
Summary
Critical illness during pregnancy presents unique challenges due to the intricate interplay of pregnancy-related physiological changes, pre-existing conditions, and healthcare system disparities. Maternal morbidity and mortality remain significant concerns globally, with disparities in outcomes observed across different regions. In high-income countries rising maternal age and increased comorbidities contribute to the complexity of obstetric care.
Extracorporeal membrane oxygenation (ECMO) has emerged as a crucial intervention for managing critical illness during pregnancy, providing respiratory and/or cardiac support when conventional therapies fall short. The utilization of ECMO in pregnant patients has increased rapidly over the past two decades, with indications varying between the antenatal and postpartum periods.
Managing physiological changes during pregnancy, such as increased cardiac output and metabolic demands, requires close attention and adjustment of ECMO parameters. Maternal and fetal acid-base status, uteroplacental function, and fetal monitoring are critical considerations during ECMO therapy. Furthermore , multidisciplinary planning is mandatory to optimize maternal stability, fetal support, and establish a delivery plan that is timed to minimize the incumbent risks of ECMO initiation, maintenance, and anticoagulation. Despite the inherent challenges, ECMO has shown promising outcomes in pregnant patients, with high maternal survival rates and favorable fetal outcomes reported in various studies. Timely consideration for ECMO initiation is advisable when confronted with severe cardiac or respiratory decompensation, which may involve early referral and transfer of high-risk parturients to a tertiary center providing ECMO support. Multidisciplinary collaboration and specialized care are essential in optimizing ECMO therapy for pregnant patients, highlighting the importance of a coordinated approach in managing critical illness during pregnancy.
7
Practice points
- •
ECMO is an effective rescue therapy for parturients with severe cardiopulmonary dysfunction.
- •
Early transfer to a specialized ECMO center is recommended for critically ill pregnant patients refractory to standard care.
- •
Multidisciplinary team planning is essential to management of ECMO support in this patient population.
- •
Fetal wellbeing during maternal ECMO support depends on maintenance of uteroplacental perfusion.
- •
Maternal adaptations during pregnancy include evolving cardiopulmonary changes throughout gestation, altered acid-base buffering, and hypercoagulability. An understanding of these phenomena entails tailored ECMO support for this population.
8
Research agenda
- •
Optimal anticoagulation strategies for ECMO maintenance continue to evolve. ECMO support during pregnancy represents a challenging balance between maternal hypercoagulability and bleeding risks related to fetal delivery, surgical procedures, and maintenance of the ECMO circuit.
- •
There are limited data to inform ideal delivery planning for pregnant patients receiving ECMO support or being considered for ECMO initiation.
- •
There is a lack of long-term outcome data for neonates delivered during or after maternal ECMO support.
CRediT authorship contribution statement
M.J. Wong: Writing – review & editing, Writing – original draft, Conceptualization. B.S. Kodali: Writing – review & editing, Writing – original draft, Conceptualization. S. Rex: Writing – review & editing, Writing – original draft, Conceptualization.
Sources of funding
None.
Declaration of competing interest
None.
Acknowledgements
None.
References
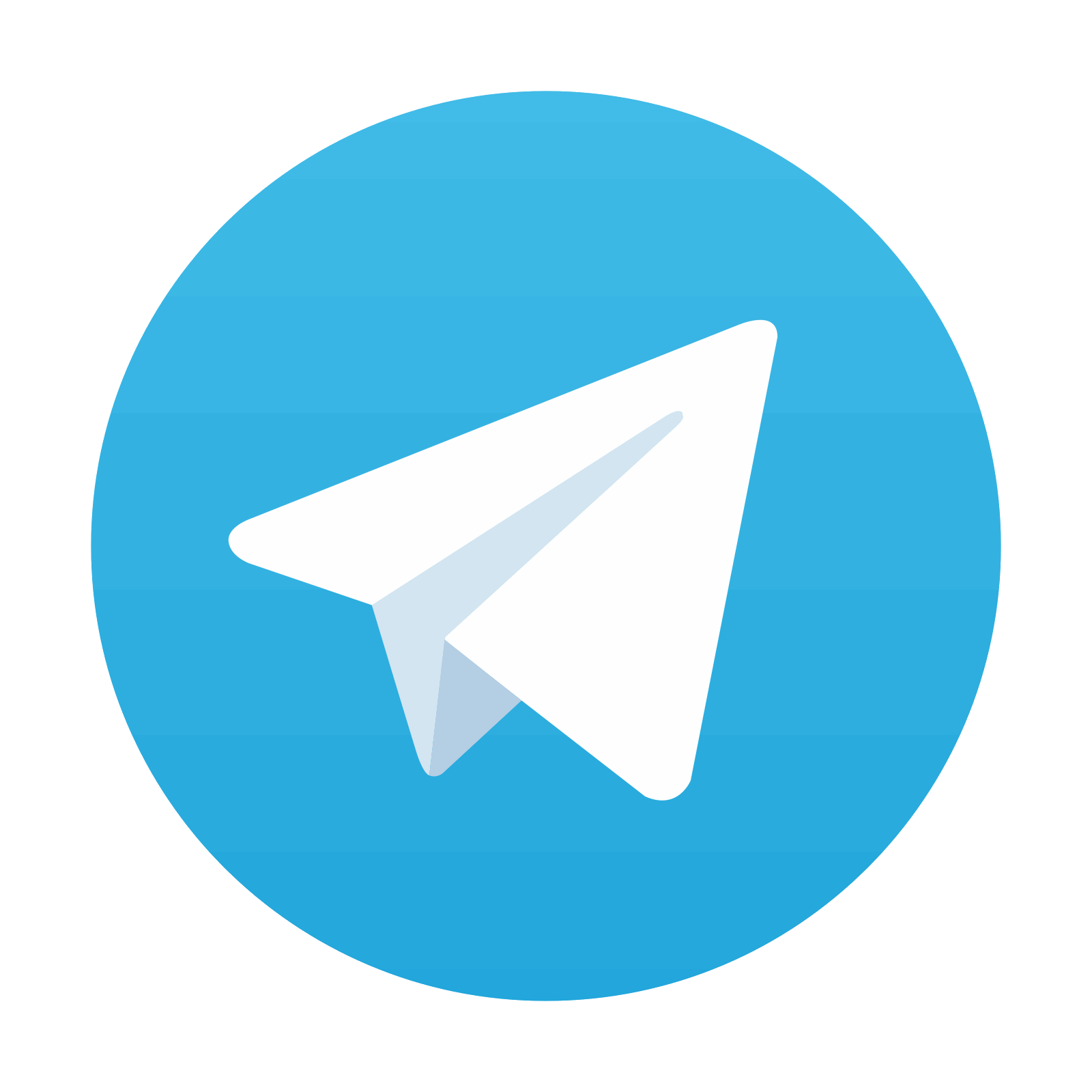
Stay updated, free articles. Join our Telegram channel

Full access? Get Clinical Tree
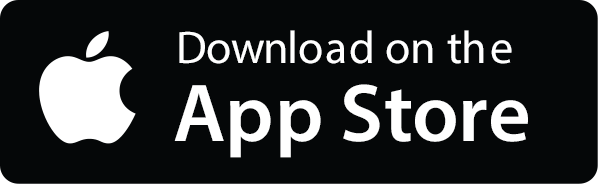
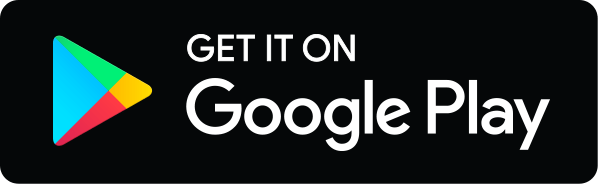
