Extracorporeal Life Support
Graeme Maclaren
Steven A. Conrad
Heidi J. Dalton
KEY POINTS









Extracorporeal life support (ECLS) is the use of a modified cardiopulmonary bypass circuit to support children with refractory respiratory or circulatory failure. This form of life support technology, also known as extracorporeal membrane oxygenation (ECMO), has been used successfully in children since the 1970s and is now regarded as standard therapy in tertiary neonatal and PICUs. Venous blood is removed from the patient, pumped through an oxygenator where carbon dioxide removal and oxygenation occur, and then returned back into the venous (venovenous [VV] ECLS) or arterial circulation (venoarterial [VA] ECLS). Venovenous ECLS is used almost exclusively to provide respiratory support whereas VA ECLS is used for circulatory support or in combined cardiopulmonary failure.
In addition to providing oxygenation, carbon dioxide clearance, and potential circulatory support, another advantage to ECLS is facilitating a reduction in other forms of life support that can perpetuate organ dysfunction. For example, ECLS allows substantial reduction in (or even cessation of) mechanical ventilation and inotropic therapy, thus reducing the risks of ventilator-induced lung injury, myocardial dysfunction, and end-organ damage.
CIRCUIT COMPONENTS
The components of an ECLS circuit, although based on the traditional cardiopulmonary bypass circuit, have been developed or adapted for long-term support (Fig. 40.1). Vascular cannulas are placed for blood drainage and reinfusion. A small drainage reservoir (or bladder) helps to ensure continuous availability of blood for the pump. This reservoir is essential in roller-pump circuits and optional in centrifugal pump circuits. A roller or centrifugal pump provides blood flow through the circuit. An artificial lung (oxygenator) provides gas exchange and a heater-cooler provides precise temperature control. Other circuit components allow for infusion of medications, incorporation of a hemofilter or other adjunctive techniques such as plasmapheresis, and monitoring systems for blood gas, flow, and pressure.
Oxygenator
The oxygenator, also referred to as a membrane lung, provides gas exchange between the blood and the atmosphere. Although called an oxygenator, it is more appropriately called an artificial lung, as it transports CO2 as well as O2. The need to provide support for days to weeks imposes challenging requirements on oxygenator design.
The traditional oxygenator that has been used since the beginning of ECLS until recently is the spiral-wound solid silicone sheet membrane lung (Fig. 40.2). It consists of two long sheets of silicone sealed at the edges and wound on a polycarbonate support. Gas manifolds are attached at the ends and other manifolds provide blood distribution between the rolls. Sizes range from 0.6 to 4.5 m2. The larger devices have integrated heat exchangers. Although they served well in the past,
silicone membrane oxygenators had a number of problems compared with the latest generation of oxygenators, including higher resistance to flow, uneven distribution of flow, larger priming volumes, and a bulkier design that compounded the difficulties of ECLS patient transport.
silicone membrane oxygenators had a number of problems compared with the latest generation of oxygenators, including higher resistance to flow, uneven distribution of flow, larger priming volumes, and a bulkier design that compounded the difficulties of ECLS patient transport.
![]() FIGURE 40.1. Components of a typical extracorporeal circuit. The circuit consists of a drainage reservoir (bladder), blood pump, membrane lung, heater-cooler, and connecting tubing. In many modern centrifugal pump circuits, the heater-cooler is integrated into the oxygenator and no reservoir is used (see Fig. 40.9). |
The latest generation of artificial lungs is hollow-fiber oxygenators, constructed with polymethylpentene, which allows gas but not liquid transfer. Smaller than the old silicone membrane lungs, they may cause less platelet consumption and provide more effective gas exchange (1,2). Many of these devices are also coated with heparin to decrease the risk of clotting. Blood flows over the hollow fibers while fresh gas (100% oxygen or an air/oxygen mixture) runs through them, facilitating gas exchange (Fig. 40.3). The rate of fresh gas flow (“sweep”) is the principal determinant of carbon dioxide clearance. Recent evaluation of data on oxygenator use from the Extracorporeal Life Support Organization (ELSO) shows that over 95% centers now use hollow-fiber oxygenators instead of solid membrane lungs.
Blood Pump
Two types of blood pumps are currently used for ECMO. The traditional pump, still widely used throughout North America, is the roller pump, a positive-displacement pump in which a rotating roller head squeezes a length of blood-filled tubing against a backing plate as the roller head rotates (Fig. 40.4). This pump is used with gravity drainage, and thus it is necessary to use an assist reservoir (bladder) at the pump inlet to maintain a continuous supply of blood to the pump, as inlet occlusion can result in large negative pressures (-500 mm Hg or more). In the event of outlet tubing obstruction, the pump can generate pressures high enough to cause tubing rupture, requiring continuous monitoring of circuit pressures. When properly used and monitored, this type of pump has a low incidence of complications.
The centrifugal pump is a nonocclusive pump that generates flow via a rotating impeller (Fig. 40.5) and has been used for long-term support in many countries outside North America for over a decade. The impeller generates a constrained vortex, creating active suction at the pump inlet which siphons venous blood into the pump, and positive pressure at the pump outlet which propels blood through the oxygenator and back into the patient. These pumps have extremely low incidence of mechanical failure. The main risk with their use is hemolysis. When there is sudden inadequate inflow of blood into the pump (e.g., from hypovolemia or kinking of the venous cannula), the suction generated by the pump is strong enough to cause cavitation and red blood cell lysis (3). A second source of hemolysis in the absence of cavitation is the use of high pump speed in the face of reduced flow from the pump, continuously applying high shear rates with resultant shear injury, and hemolysis. These risks can be lowered by monitoring pump inlet pressure and plasma-free hemoglobin.
The choice of pump and oxygenator depends on product availability and institutional experience and resources. There is some evidence that the latest generation of ECLS equipment is associated with fewer mechanical complications and better patient outcomes than traditional circuitry (4).
Vascular Cannulas
Extracorporeal life support requires high blood flows, often equal to cardiac output and occasionally higher. Cannulas of sufficient sizes to support this level of flow are necessary. The single-lumen cannulas used for vascular access are not unique to ECLS, having been developed for cardiovascular surgery. Most cannulas are constructed of polyurethane and may include wire reinforcement to prevent kinking. Cannulas that are placed percutaneously require appropriately sized tissue dilators.
Traditional cannulation employs two single-lumen cannulas, one for venous drainage and a second for return to the venous or arterial circulation. Single-lumen cannulas are used in all modes of support. The cannula size chosen is dictated by the size and flow requirements of the patient and the size of the vessel(s) available for cannulation. Neonates have vessels that range from ˜6-10 French (Fr) (carotid artery) to 12-15 Fr (internal jugular vein), whereas adolescents can accommodate up to 20 Fr arterial and 24 Fr venous cannula (or larger).
Double-lumen cannulas have been developed specifically for VV ECLS (Fig. 40.6). Placed through the internal jugular vein, these cannulas have two drainage ports located near both atriocaval junctions and a reinfusion port located between these two, directed toward the tricuspid valve. Unlike single-lumen cannulation approaches, the double-lumen cannula is designed to reduce recirculation by capturing blood that returns via both vena cavas.
![]() FIGURE 40.6. Double-lumen cannula for single-vessel (right internal jugular) access for venovenous extracorporeal life support. |
Blood flow through a vascular cannula is complex and not linearly dependent on pressure. In general, flow is generally not predictable from traditional hydraulic equations. The relationship between the pressure gradient applied to a cannula and the resultant flow is characterized and published as pressure-flow curves (Fig. 40.7). The M-number system was developed to characterize flow through ECLS cannulas and represents a single index of resistance based on a correlation between Reynolds numbers and friction factor. The number is experimentally determined for each catheter and allows comparison of expected flows among different cannulas. A higher M-number represents a higher resistance and thus a lower flow at a given driving pressure. An “updated” M-number system (“UM number”) has recently been developed to simplify the application of these principles (5). Most centers have a cannulation chart for bedside use that lists cannula sizes alongside expected blood flow rates and patient weights.
OTHER COMPONENTS
Roller-pump systems require continuous availability of blood at the inlet to avoid development of large negative pressures and hemolysis. This availability is ensured by placing a small assist reservoir, or bladder, just before the pump inlet. Gravity assist is achieved by placing the reservoir and pump ˜100 cm below the level of the cannula, providing a hydrostatic siphon for drainage and maintaining a positive pressure at the pump inlet. The reservoir also buffers against fluctuations in drainage. If drainage decreases, for example, due to hypovolemia, the reservoir will begin to empty, signaling the need for correction of the cause for poor drainage. Most roller-pump systems have the capability of servoregulation. A switch situated in the reservoir holder opens when the bladder empties, turning off the pump and allowing time for filling of the reservoir. With the pump off, the bladder refills and the pump resumes operation.
Centrifugal systems do not mandate the use of a venous assist reservoir, although some centers incorporate them into their standard circuit configuration in an attempt to reduce the incidence of hemolysis. Without the reservoir, active suction can provide drainage without the need for gravity assist, allowing the equipment to be operated near the level of the patient. A constrained bladder that replaces the standard assist reservoir is available to provide compliance protection and noninvasive pressure monitoring.
The tubing of ECLS circuitry is usually made from polyvinylchloride, although polyurethane and silicone rubber have also been used. Most contemporary ECLS circuits are lined with a surface coating designed to reduce either thrombosis or inflammation, although the effectiveness of these formulations is not well proven. Examples of the former are heparin-bonded coatings and the latter include phosphorylcholine or poly (2-methoxyethylacrylate). Regardless of the coating used, ECLS circuits bind many lipophilic drugs, including sedatives and antibiotics, necessitating increased dosing and careful monitoring (6,7,8).
The heater-cooler unit is essential in children on ECLS, especially infants. It provides extremely effective temperature control such that maintaining normothermia or inducing therapeutic hypothermia is literally done at the touch of a button.
An ECMO circuit is integrated with monitoring systems to ensure safe operation and effective gas transfer. Pressure monitors are used to monitor the development of excessive pressures across the membrane oxygenator, providing an indication of the development of clotting and impairment of blood flow. Inline monitors in the drainage and postoxygenator circuit provide real-time information on venous and arterial saturation, pH, and hemoglobin concentration. Temperature monitors are used to monitor circuit and patient temperatures.
PATIENT SELECTION
Indications
Hypoxemic Respiratory Failure
Children after the first 2 weeks of life have different physiology than that of younger infants and are susceptible to a different spectrum of lung disease. Most features of fetal cardiopulmonary physiology, such as flow through the ductus arteriosus and foramen ovale, have largely disappeared by the end of this period. Respiratory failure in the newborn due to persistent pulmonary hypertension and meconium aspiration is replaced by pulmonary infection, pulmonary aspiration, sepsis, and other conditions. These illnesses often trigger acute respiratory distress syndrome (ARDS) and are frequently accompanied by extrapulmonary organ system involvement. The most common cause of hypoxemic respiratory failure in the pediatric age group that leads to support with ECLS is viral pneumonia (19%), followed by bacterial pneumonia (11%) and ARDS (10%) (9). The remaining fraction comprises respiratory failure due to a number of other etiologies.
The selection of patients for ECLS is founded in a set of criteria that have evolved over time and have historically predicted high mortality (Table 40.1). The essential element of these criteria is to select patients with severe oxygenation impairment, risk of ventilator-induced pulmonary injury, and the capacity for pulmonary recovery. Measures of oxygenation impairment include the oxygenation index (OI: the product of mean airway pressure and fraction of inspired oxygen divided by the arterial partial pressure of oxygen), PaO2/FiO2 ratio (P/F ratio), or intrapulmonary shunt fraction. The OI has been the traditional index used in neonates and younger children, with levels >40 historically considered an indication for initiating ECLS. More recent studies have also identified that serial evaluation of OI may be useful in older patients (including adults), although no strict cut-off values for ECMO initiation or predicting death have been established. The P/F ratio and intrapulmonary shunt are two interrelated measures that do not include an inflation pressure component, but they have been most commonly applied in older children and adults.
The avoidance of ventilator-induced lung injury is a major goal of ECLS. Patients who require excessive inflation
pressures, even in the absence of life-threatening hypoxemia, should be considered for extracorporeal support. This is particularly important if lactic acidosis and shock result from high levels of ventilatory support. The presence of barotrauma (radiographic evidence of pneumomediastinum or pulmonary interstitial air, or persistent air leak) should lead to consideration of ECLS, especially if it is progressive and uncontrollable.
pressures, even in the absence of life-threatening hypoxemia, should be considered for extracorporeal support. This is particularly important if lactic acidosis and shock result from high levels of ventilatory support. The presence of barotrauma (radiographic evidence of pneumomediastinum or pulmonary interstitial air, or persistent air leak) should lead to consideration of ECLS, especially if it is progressive and uncontrollable.
|
Even with these guidelines, the decision of whether to place a patient on extracorporeal support requires consideration of other factors, including comorbid conditions, contraindications to anticoagulation, duration of pre-ECLS cardiac arrest and quality of resuscitation and duration of mechanical ventilation prior to ECLS. The former contraindications to ECLS have given way to individualized decisions of risk assessment and potential benefit. It may be prudent to undertake high-risk or uncertain cases (e.g., uncertain neurologic status following cardiac arrest) with the provision that ECLS will be withdrawn if clinical information is discovered that would suggest futility.
VV support is the preferred ECLS mode for hypoxemic respiratory failure. The presence of inotropes, once used as an indication to proceed to VA support, is not a contraindication to VV support (10). In most cases, inotrope requirements are reduced as the myocardium recovers from hypoxemia and intrathoracic pressure is reduced by lowering mechanical ventilation. The presence of severe shock despite inotropes, however, is generally best supported with the VA approach. In other words, if the primary indication for ECLS is shock, VA should be regarded as the standard mode. If the primary indication is hypoxia, VV should be used irrespective of the inotrope dose. It is imperative to be able to rapidly change cannulation strategies in complex or uncertain cases and this should be factored into initial planning prior to cannulation.
Hypercapnic Respiratory Failure
Severe hypercapnia with respiratory acidosis, in particular severe asthma, is effectively managed by VV
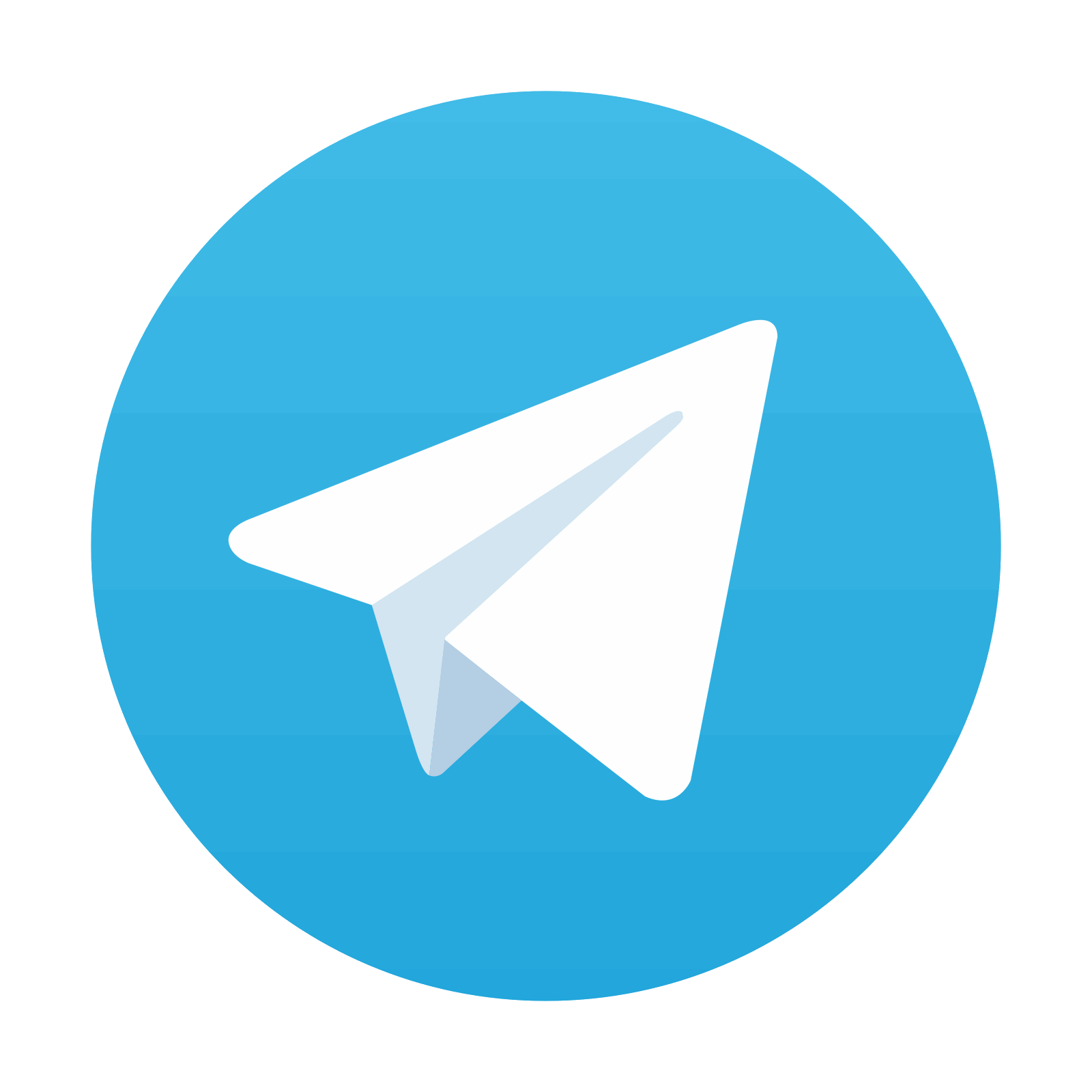
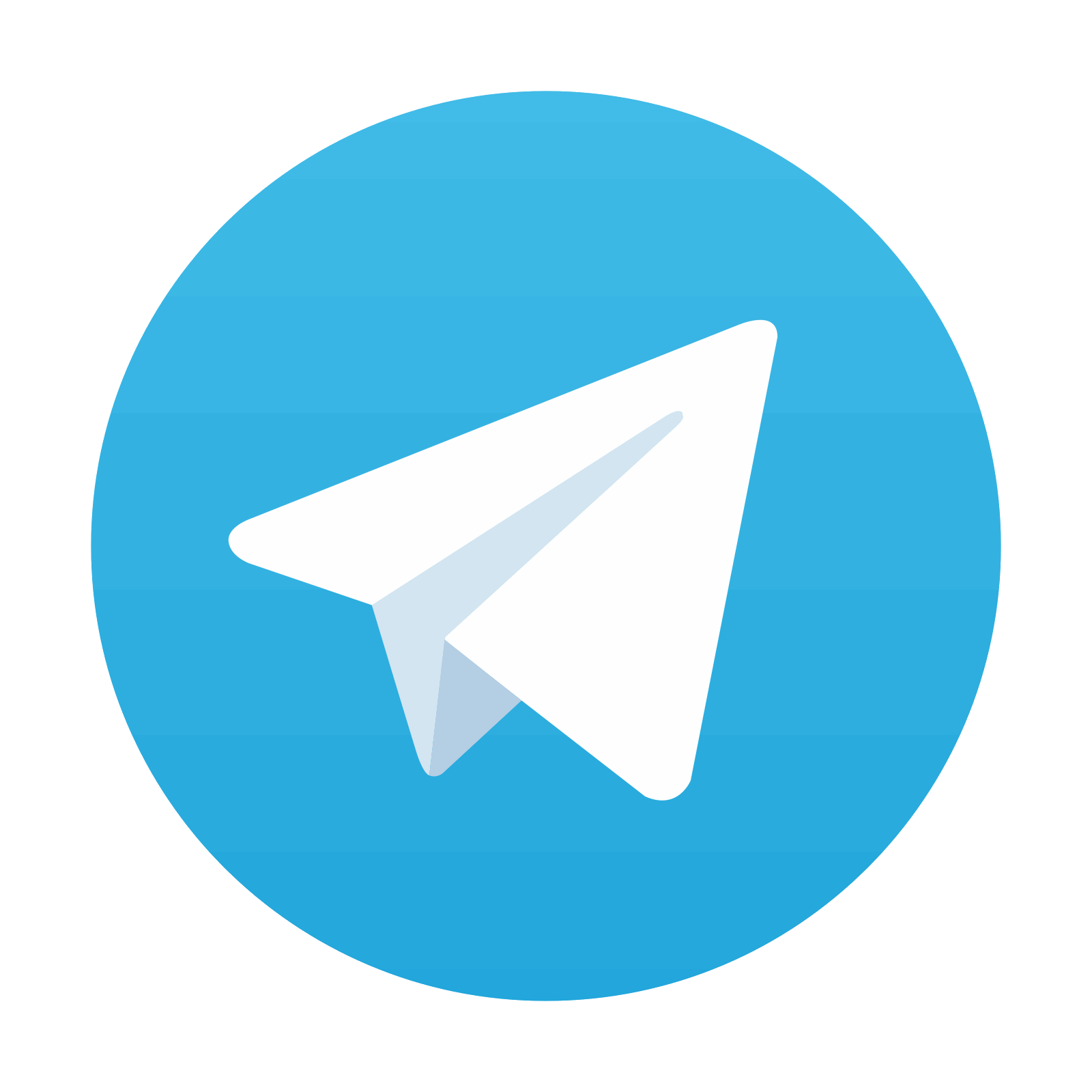
Stay updated, free articles. Join our Telegram channel

Full access? Get Clinical Tree
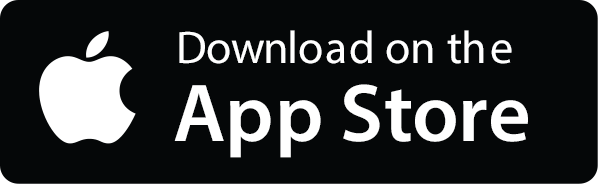
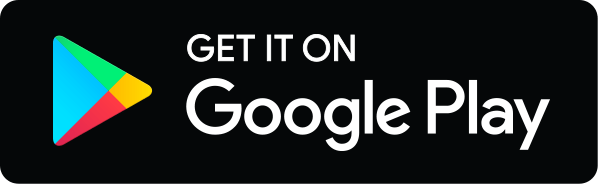
