• Marina Allen, MD
• Oscar de Leon, MD
I. | INTRODUCTION Brief History |
II. | INDICATIONS, APPLICATIONS, CONTRAINDICATIONS Indications Contraindications |
III. | ANATOMY Vertebral Column Joints of the Vertebral Column Ligaments Spinal Cord/Spinal Canal Surface Anatomy, Structures Superficial to the Epidural Space |
IV. | PHYSIOLOGIC EFFECTS OF EPIDURAL BLOCKADE Cardiovascular Respiratory Gastrointestinal Renal/Genitourinary Neuroendocrine |
V. | PHARMACOLOGY OF EPIDURAL BLOCKADE Nerve Impulse Physiology Action of Local Anesthetics Mechanism of Action of Local Anesthetics for Neuraxial Blockade Choice of Local Anesthetics Onset/Duration of Local Anesthetics Novel Additives to Local Anesthetics in the Epidural Space Other Factors Affecting Epidural Blockade Dose, Volume, and Concentration |
VI. | CLINICAL APPLICATION Patient Evaluation Patient Preparation Communication with Surgical Staff Equipment Patient Positioning Technique Problem Solving |
VII. | COMPLICATIONS OF EPIDURAL BLOCKADE Drug-Related Complications Procedure-Related Complications |
VIII. | SUMMARY |
INTRODUCTION
Epidural blockade is one of the most useful and versatile procedures in modern anesthesiology. It is unique in that it can be placed at virtually any level of the spinal spine, allowing more flexibility in its application to clinical practice. It is more versatile than spinal anesthesia, giving the clinician the opportunity to provide anesthesia and analgesia, as well as enabling diagnosis and treatment of chronic disease syndromes. It can be used to supplement general anesthesia, decreasing the need for deep levels of general anesthesia, therefore providing a more hemodynamically stable operative course and faster emergence from general anesthesia. It provides better postoperative pain control and more rapid recovery from surgery. When combined with spinal anesthesia in a technique called a CSE (combined spinal-epidural), benefits of both techniques can be combined and shortcomings of each avoided.
Numerous studies have demonstrated the benefits of epidural blockade. Epidural anesthesia or analgesia can reduce the adverse physiologic responses to surgery such as autonomic hyperactivity, cardiovascular stress, tissue breakdown, increased metabolic rate, pulmonary dysfunction, and immune system dysfunction. Thoracic epidural analgesia has been shown to decrease the incidence of myocardial infarction1 postoperative pulmonary complications2,3 and to promote the return of gastrointestinal motility without compromising fresh suture lines in the GI tract.4–6 Epidural anesthesia and analgesia reduces the incidence of hypercoagulability. 7,8 Well-conducted randomized trials have demonstrated the perioperative use of epidural anesthesia and analgesia may reduce overall mortality and morbidity by approximately 30% compared with general anesthesia using systemic opiods.9
This chapter aims to provide the information necessary to provide safe and effective epidural blockade. The reader is encouraged to review specific chapters in this text for a more detailed discussion on specialized topics such as local anesthetics, combined spinal-epidural anesthesia, obstetric anesthesia, and serious complications such as epidural hematomas.
Brief History
Two French physicians, Jean-Anthanase Sicard, a radiologist, and Ferdinand Cathelin have been credited with the intentional administration of caudal epidural anesthesia over a century ago in 1901. They found that injecting a dilute solution of cocaine through the sacral hiatus can provide an effective treatment for severe sciatic pain and suggested the technique for surgical procedures.10 Nineteen years later, a Spanish military surgeon by the name of Fidel Pages Mirave, is credited with describing the lumbar approach to “peridural” anesthesia. Unfortunately he was killed in an accident at the early age of 37, and his work lay dormant for several years.11 Then in 1931, an Italian surgeon, Archile Dogliotti, performed abdominal surgery using single-shot lumbar epidural anesthesia, popularizing the method for producing “segmental peridural anesthesia.” He noted that a sufficient length of spinal nerves needed to be blocked with an anesthetic solution of sufficient quantity to provide adequate anesthesia. He correctly identified the epidural space by describing the sudden loss of resistance noted after the needle had crossed the ligamentum flavum.12
Aburel, Hingson, and Edwards all devised methods for continuous but cumbersome epidural blockade; however, Cuban anesthesiologist, Manual Martinez Curbelo, is credited with making the technique more practical. On a visit to the Mayo Clinic in 1947, he watched Edward Tuohy perform continuous spinal blocks. Tuohy had replaced sharp spinal needles with a curved tip design developed by Ralph Huber. Tuohy modified the needle by adding a stylet to decrease the risk of skinplugging during insertion. Curbelo then used the Tuohy needle with a silk ureteral catheter to provide continuous segmental lumbar “peridural” anesthesia.13 Several modifications of the Tuohy-Huber epidural needle have been developed in the more recent past and are being utilized in modern anesthesia practice. The epidural catheters have undergone more major changes since the original 3.5 F silk catheter used by Curbelo. The silk catheters were difficult to sterilize and prone to causing serious infections. Polymers of nylon, Teflon, polyurethrane, and silicone are currently used by manufacturers to produce thin, kink-resistant catheters of appropriate tensile strength and stiffness.10,14
Combining spinal with epidural anesthesia (CSE) began shortly after epidural anesthesia was reintroduced by Dogliotti. In 1939, Dr. A. L. Soresi presented a paper in which he and his colleagues provided a combination of spinal and epidural anesthesia safely to over 200 patients. He used one needle to first enter the epidural space, injected local anesthesia, then used the same needle to enter the subarachnoid space, dosing the patient with a smaller amount of local anesthetic. He demonstrated that he was able to provide anesthesia to his patients for 24 to 48 h.15 In 1979, a Swedish physician named. Curelaru was the first to describe a combined technique using separate intervertebral injections. Then in 1982, Coates from England and Mumtaz from Sweden published reports of the popular needle-through-needle approach16,17 for combined spinal-epidural anesthesia. Since that time, modifications of the CSE needle delivery systems as well as the creation of special trays for the procedure have been developed and are being utilized in the practice of modern anesthesiology.
INDICATIONS, APPLICATIONS, CONTRAINDICATIONS
Indications
Epidural anesthesia has been typically limited to procedures involving the lower limbs, pelvis, perineum, and lower abdomen. As clinicians have become more experienced with its application, epidural anesthesia with or without sedation has been used as the sole anesthetic or in combination with general anesthesia for a larger variety of cases. Michalek reported the use of cervical epidural anesthesia at the C6-7 level for a total parathyroidectomy with parathyroid gland implantation into the forearm. He concluded that combined procedures involving the neck and upper limbs could be safely conducted under cervical epidural blockade.18 Several studies have described the use of high thoracic epidural anesthesia for off-pump coronary revascularization19,20 and even for minimally invasive aortic valve replacement,21 although this has not become common practice in the United States. In patients in whom general anesthesia could lead to prolonged ventilatory care, such as those with diffuse interstitial lung disease, thoracic epidural anesthesia as the sole anesthetic has been described as a successful alternative.
Although it is intriguing to realize that epidural blockade can be performed for procedures that in the past were limited to general anesthesia, the decision about whether to use this form of neuraxial blockade should be determined by the needs of the patient. Physiologically, blocks above T5 have a far greater effect on patient hemodynamics than blocks at T10 or lower. However, if the benefits of epidural blockade outweigh the risks to the patient, and the sensory blockade needed for the particular procedure can be obtained, then it is indicated. This distinction is sometimes blurred in teaching institutions when the desire to “practice” overshadows the needs of the patient, or when impatient surgeons do not want to allow novice epiduralists the time to safely perform the procedure. By keeping the needs of the patient in the forefront, this should never be a problem in modern day clinical practice. Common applications for epidural blockade are listed in Table 14-1.
Contraindications
Contraindications to epidural blockade have been historically divided into absolute, relative, and controversial (Table 14-2).
Absolute
Absolute contraindications include patient refusal, severe, uncorrected hypovolemia (sympathectomy with hypovolemia may cause profound circulatory collapse), increased intracranial pressure (may predispose patient to brainstem herniation if accidental dural puncture occurs or if a large volume of anesthetic is rapidly injected into the epidural space), and infection at the site of injection.22 True allergy to local anesthetics of both classes, although exceedingly rare, is also an absolute contraindication.
Relative
Coagulopathy, whether iatrogenic or idiopathic, previously an absolute contraindication, is now considered a relative contraindication.23 Anticoagulants, however, should be withheld based on the mechanism of action and duration of the drug. The greatest concern with anticoagulant therapy and anticoagulation is the risk of an epidural hematoma (refer to epidural hematoma chapter). According to the consensus statements by the American Society of Regional Anesthesia and Pain Medicine,23 epidural block can be placed 4 h after the last dose of subcutaneous heparin, and 12 h after the last dose of low-molecular-weight heparin. NSAIDs, including aspirin, are not contraindications to epidural placement provided that catheter placement is uncomplicated (one or two attempts). Other relative contraindications include uncooperative patient (therefore exposing neural structures to an unacceptable risk of injury), fixed cardiac output states (inability to increase cardiac output in response to sympathectomy), anatomic abnormalities of the vertebral column (making placement technically impossible), and unstable neurologic disease (may mask exacerbation signs/symptoms).24,25 Case reports have been published on patients undergoing epidural anesthesia safely with serious neurologic conditions such as neural tube defects, Guillain-Barré syndrome, and quadriplegia with autonomic hyperreflexia.26–28 Careful evaluation and documentation of the patient’s baseline neurologic status, a thorough discussion of risks as well as the benefits of epidural anesthesia and a multidisciplinary approach is required for these patients. The same approach should be taken with any patient with serious medical diseases prior to instituting epidural blockade.
Common Applications for Epidural Blockade
Orthopedic surgery | Major hip/knee surgery, pelvic fractures |
| |
Obstetrics | Cesarean section, labor analgesia |
| |
Gynecologic surgery | Procedures involving female pelvic organs |
| |
Urologie surgery | Prostate, bladder procedures |
| |
General surgery | Upper and lower abdominal proceduresa |
| |
Pediatric procedures | Penile procedures, inguinal hernia repair, anal surgery, orthopedic procedures on the feet; supplement to GA, postoperative pain relief, orthopedic procedures on feetb |
| |
Vascular surgery | Vascular reconstruction of the lower limbs, amputations involving the lower extremities |
| |
Thoracic surgery | Postoperative analgesia, combination with GA to reduce GA requirements |
| |
Medical conditions | Known/suspected malignant hyperthermia |
| |
Diagnosis and management of chronic pain | Chronic pain |
a Height of block with side effects required for upper abdominal procedures, may make it difficult to avoid patient discomfort and increased risk.
b Usually through a caudal epidural approach.
Clinical Pearls
Epidural blocks can be placed 4 h after the last dose of subcutaneous heparin, 12 h after the last dose of LMWH.
Contraindications: Epidural Blockade
Absolute | Relative | Controversial |
| ||
Patient refusal | Coagulopathy | Inadequate training/experience |
| ||
Platelet count < 100,000 | ||
| ||
Uncorrected hypovolemia | Uncooperative patient | Elaborate tattoos at the needle insertion site |
| ||
Increased ICP | Severe anatomic abnormalities of spine | Positioning that compromises respiratory status |
| ||
Infection at site | Sepsis | Anesthetized patient (cervical/thoracic) |
| ||
Allergic to amide/ester LA | Hypertension | Previous back surgery |
ICP = intracranial pressure; LA = local anesthetic
The more controversial contraindications to epidural anesthesia include inability to communicate with the patient (placing an epidural in an anesthetized patient),29 tattoos (potential risk of pigment-containing tissue coring into the epidural space),30 complicated surgeries with major blood loss,25 and in surgical maneuvers in which respiration maybe compromised or the airway may be difficult to manage.
Clinical Pearls
NSAIDs (including ASA) are not contraindications to epidural placement.
LMWH should be held at least 12 h before placement of catheter and 2 h after removal.
Epidural placement is relatively safe with internationalized ratio (INR) <1.5.
If an epidural vein is punctured, subcutaneous heparin administration should be held at least 2 h, and LMWH held at least 24 h.
Glla/IIIb inhibitors should be withheld for at least 4 weeks after epidural placement.
Epidural placement is best avoided for 7 days after clopidogrel and 14 days after ticlopidine.
ANATOMY
The key to safe and effective administration of an epidural blockade begins with a thorough understanding of the anatomy of the vertebral column, ligaments, and blood supply, the epidural space, spinal canal, and associated structures. A three-dimensional mental picture of surface structures as they relate to internal structures helps the clinician troubleshoot difficult epidural placement.
Vertebral Column
General Appearance
The vertebral column consists of 7 cervical, 12 thoracic, and 5 lumbar vertebrae. At the caudal end, the 5 sacral vertebrae are fused to form the sacrum, and the 4 coccygeal vertebrae are fused to form the coccyx (Figure 14-1). The primary functions of the vertebral column are to maintain erect posture, to encase and protect the spinal cord, and to provide attachment sites for the muscles responsible for movements of the head and trunk.31 The normal spinal column is straight when viewed dorsally or ventrally. When viewed from the side, there are two ventrally convex curvatures in the cervical and lumbar regions, giving the spinal column the appearance of a double “C”.
Structure of Vertebrae
Each vertebra is composed of a vertebral body and a bony arch. The arch consists of two anterior pedicles and two posterior laminae. The transverse processes are located at the junction of the pedicles and lamina, and the spinous process is located at the junction of the laminae. The spinous processes vary in their angulation in the cervical, thoracic, and lumbar regions. The spinous processes are almost horizontal in the cervical, lower thoracic, and lumbar regions, but become significantly more sharply angled in the midthoracic region (Figure 14-2). The greatest degree of angulation is found between the T3 and T7 vertebrae, making insertion of an epidural needle in the midline more difficult.
The shape and size of the vertebrae differ from the cervical to the lumbar region secondary to function. The vertebral bodies are smaller in the cervical region an become progressively larger in the lumbar area where they support the greatest amount of weight (Figure 14-2).
Figure 14-1. Anatomy of the vertebral column.
Joints of the Vertebral Column
The vertebrae articulate at the intervertebral and facet joints. The intervertebral joints are located between adjacent vertebral bodies. They maintain the strength of attachment between vertebrae. The facet joints form between articular processes. The facet joints are heavily innervated by the medial branch of the dorsal ramus of the spinal nerves. This innervation serves to direct contraction of muscle that moves the vertebral column.
Ligaments
The vertebrae are joined together by a series of ligaments and disks. Anteriorly, the vertebral bodies are separated by the intervertebral disks. The ligament connecting them runs from the base of the skull to the sacrum and is called the anterior longitudinal ligament. The posterior surface of the vertebral bodies is connected by the posterior longitudinal ligament, which also forms the anterior wall of the vertebral canal. The other ligaments of importance (Figure 14-3):
• Intertransverse ligament: connects transverse processes
• Supraspinous ligament: attaches to the apices of the spinous processes, extends from sacrum to skull where it becomes the ligamentum nuchae
• Interspinous ligament: connects spinous processes
• Ligamentum flavum: thick, elastic ligament, connects the laminae, composed of a right and left ligament that joins in the middle forming an acute angle; narrows toward the articular processes
Figure 14-2. Comparison of cervical, thoracic, and lumbar vertebrae.
Spinal Cord/Spinal Canal
The spinal canal is formed by adjacent vertebral foramina. The canal provides support and protection to the spinal cord and its nerve roots. The spinal cord extends from the foramen magnum to the L1-2 vertebral level in adults, and L3 vertebral level in children before becoming the conus medullaris.
Figure 14-3. When performing an epidural, the needle passes through the following:
– skin
– subcutaneous fat
– supraspinous ligament
– interspinous ligament
– ligamentum flavum
From the spinal cord extends a series of dorsal and ventral roots that converge to form mixed spinal nerves. The mixed nerves contain motor, sensory, and in many cases, autonomic fibers. There are eight cervical, 12 thoracic, 5 lumbar, 5 sacral, and 1 coccygeal pairs of spinal nerves. The roots inferior to the conus medullaris become the cauda equina before exiting through the lumbar and sacral foramina. After the spinal nerves leave the spinal canal through the intervertebral foramina, they divide into the anterior and posterior primary rami. The posterior primary rami innervate the skin and muscles of the back. The anterior rami supply the rest of the trunk and the limbs. Each spinal nerve supplies a specific region of skin referred to as a dermatome (Figure 14-4). There is overlap between adjacent segmental nerves. Loss of a single spinal nerve will produce an area of altered sensation, but won’t result in total sensory loss. For instance, destruction of at least three consecutive spinal nerves is required to produce a total sensory loss of the dermatome supplied by the middle nerve of the three.
Preganglionic fibers of the sympathetic nervous system originate from the spinal cord from T1 to L2. They travel with spinal nerves to form the sympathetic chain. This chain extends the entire length of the spinal column on the anterolateral aspects of the vertebral bodies. The chain gives rise to the stellate ganglion, splanchnic nerves, and the celiac plexus (Figure 14-5).
Simple surface landmarks can be used to indicate the level of dermatomal blockade, which correlates with the segmental spinal nerve (Figure 14-6). The degree and effect of sympathetic block depends on the height of the block (Table 14-3)
Meninges/Meningeal Spaces
Surrounding the spinal cord and its roots are three layers of membranes. The innermost layer is called the pia mater, which attaches intimately to the surface of the spinal cord and roots of the spinal nerves. As the roots of the spinal nerves extend distally, the pia mater transforms into the second layer called the arachnoid. The aranchoid detaches from the roots and reflects back across the pia, enclosing the spinal cord within a cavity called the subarachnoid space. The space is filled with cerebrospinal fluid and transmits blood vessels to and from the spinal cord. Superficial to the arachnoid is the thick dura mater. The space between the arachnoid and dura is called the subdural space. Because the arachnoid is pushed against the dura mater by the pressure of the CSF, the subdural space is negligible. It contains a small amount of serous fluid which allows the dura and arachnoid to move over each other.32 Because of its exceedingly small volume, it is referred to as a potential space.
Clinical Pearls
Unintentional injection into the subdural space during epidural anesthesia explains the occasional patchy epidural, high epidural/spinal level or total spinal after epidural placement.
Key features of a subdural injection: diffuse, spotty anesthesia with a delayed onset for 15 to 30 min, or clinical presentation of a high or total spinal.
The subdural space extends into the skull, so LA injected into this space can affect higher levels in the brain than epidural medications—watch for neurologic changes.
Epidural Space
The epidural space is smaller than the subarachnoid space, extends from the base of the skull to the sacral hiatus, and surrounds the dura mater anteriorly, laterally, and posteriorly. The epidural space is bound posteriorly by the ligamentum flavum and laterally by the pedicles and the intervertebral foramina. It is filled with the fat, areolar tissue, lymphatics, veins, and nerve roots that traverse it, but there is no free fluid (Figure 14-7). The volume of fat is greater in obese individuals and less in the elderly. It is postulated that the decrease in epidural fat explains the age-related changes in epidural dose requirements.32,33
Figure 14-4. Body dermatomes.
The epidural space is rich in blood vessels, including Batson’s venous plexus. Batson’s plexus is continuous with the iliac vessels in the pelvis and the azygos system in the abdominal and thoracic body walls (Figure 14-8). Because this plexus has no valves, blood from any of the connected systems can flow into the epidural vessels. This is especially important in obstetrics when compressed caval vessels can lead to engorgement of the epidural veins, increasing the risk of catheter entry into a vein. The engorgement is even greater at the intervertebral foramina where the vessels may bulge from the vertebral canal. Therefore, the incidence of penetrating a blood vessel with an “off-midline” needle insertion may be more likely.
Figure 14-5. Preganglionic fibers of the sympathetic nervous system.
Figure 14-6. Surface landmarks for various dermatomal levels of epidural blockade.
Clinical Pearls
Blood vessel puncture during epidural placement is more common in the pregnant patient due to engorgement of the epidural veins from caval compression.
Off-midline needle insertion may more commonly result in blood vessel puncture due to engorged epidural veins converging into the intervertebral foramina.
Controversies Regarding the Anatomy of the Epidural Space
Through endoscopic examination, computed tomography (CT), magnetic resonance imaging (MRI), and cryomicrotome sectioning of cadavers, the epidural space has been found to be more segmented and less uniform34 than traditionally taught (Figure 14-9). In a cadaver study, Blomberg35 identified a midline band that he attributed to septations in the epidural space. This was later suggested by Hogan36 to actually be an artifact of the midline posterior epidural fat pad. Between the epidural segments, the dura is adjacent but not adherent to the periosteum of the vertebra, making this space a potential space that can be dilated by the injection of fluid. It has been postulated that these anatomic variations in the epidural space account for the unilateral block or unpredictable epidural drug spread that is occasionally seen.32,36
Surface Anatomy, Structures Superficial to the Epidural Space
Surface structures assist the clinician in determining the level of entry into the epidural space. The spinous processes help define the location of the midline as they are usually midline structures. The cervical and lumbar spinous processes are horizontally directed whereas the T4 through T9 thoracic spinous processes have a sharp caudal angulation (Figure 14-10).
Correlating Dermatomal Level to Surface Landmarks
Dermatomal Level | Surface Landmark | Comments |
| ||
C8 | Little finger | Cardioaccelerator fibers blocked (T1 to T4) |
| ||
T1,T2 | Inner aspect of the arm | Above fibers blocked but to lesser degree |
| ||
T4 | Nipple line, root of scapula | Cesarean section, Appendectomy, upper abdominal surgery |
| ||
T7 | Inferior border of scapula Tip of xiphoid | Splanchnic (T5 to LI) blockage; lower abdominal surgery; T5 to T7 for thoracotomy or fractured ribs (at relevant interspace) |
| ||
T10 | Umbilicus | Usual level for LE procedures, hip surgery, TURP, vaginal delivery |
| ||
L2 to L3 | Anterior thigh | Appropriate for knee, foot surgery |
| ||
SI | Heel of foot | Part of sacral plexus, difficult to block |
LE —lower extremity; TURP= transurethral prostatectomy.
Clinical Pearls
Needle entry into the cervical and lumbar regions can be directed medially with a slight upward angulation, whereas in the upper thoracic region, a midline approach to the epidural space is more difficult because of the angulation of the spinous processes. A paramedian approach is usually more successful.
The safest point of entry into the epidural space is below the level of the spinal cord. In adults, this corresponds to the lower border of the LI vertebrae, and in children, at the lower border of the L3 vertebrae. Epidural insertion in adults is commonly introduced at either the L3-4 interspinous space or one higher, L2-3. A line drawn between the superior aspect of the iliac crests crosses either the spinous process of L4 or the L4-5 interspace. The interspinous space above this point (L3-4 interspinous space) or one higher (L2-3) can safely be chosen for needle entry into the epidural space of adults (Figure 14-6). Some research has challenged the accuracy of the iliac crest in assessing the level, but this is still a generally accepted surface landmark.3738–39 By approximately the age of 8 years, the same interspaces can be chosen for children; however, under the age of 7, to avoid accidental cord injury the caudal approach to the epidural space is safer.
Anatomic landmarks used to identify vertebral levels prior to insertion of an epidural needle are summarized in Table 14-4.
Distance from Skin to Epidural Space
The last anatomic feature of clinical importance pertaining to the epidural space is the distance from the skin to the space. The depth varies depending on the body habitus. The distance is 4 cm in 50% of the population, and 4-6 cm in 80% of the population.24,40,41 It is noteworthy that studies demonstrating skin-to-epidural space in extremes of weight have shown that in the thin patient, the distance can be less than 4 cm, and in the obese, greater than 8 cm.24,42
Clinical Pearls
The distance from skin to epidural space in the midline is 4-6 cm in 80% of the population.
This distance can be shorter in thin patients and longer in obese patients.
PHYSIOLOGIC EFFECTS OF EPIDURAL BLOCKADE
The primary site of action of local anesthetic solutions injected into the epidural space is the spinal nerve roots. The segmental nerve roots in the thoracic and lumbar regions are mixed nerves, containing somatic sensory, motor, and autonomic nerve fibers. Sensory blockade interrupts the transmission of both somatic and visceral painful stimuli, whereas motor blockade provides muscle relaxation with a varying degree of sympathetic blockade.25 The injection site for epidural anesthesia should be close to the nerve roots of interest in order to obtain the best results with minimal amount of local anesthetic and decreased risk of side effects from systemic absorption of the local anesthetic (catheter/incision- congruent).25,43
Figure 14-7. Epidural space: 1. Anterior epidural space, 2. Posterior epidural space, 3. Ligamentum flavum, 4. Blood vessels in the epidural space, 5. Pedicles, 6. Nerve roots, 7. Transverse process, 8. Vertebral body, 9. Spinal cord.
Differential nerve block, an important concept for epidural anesthesia, refers to the phenomenon in which nerve fibers with different functions demonstrate a varying sensitivity to the effects of local anesthetics. Sympathetic fibers are usually blocked first followed by pain/temperature, then proprioception, followed by motor blockade. After an epidural block, sympathetic blockade (temperature) may vary from zero to four segments higher than the sensory block level (pain/light touch), which is two segments higher than motor blockade. Regression of the block occurs in reverse order.
The physiologic effects of epidural blockade on organ systems depends on the spinal level and the number of spinal segments blocked. In general, high thoracic epidural blocks and extensive epidural blocks are associated with more profound sympathetic block, resulting in a more profound physiologic effect in the cardiovascular system.
Cardiovascular
Block Below T4
The effect of epidural anesthesia on the cardiovascular system depends on the level and the degree of sympathetic blockade. Vasomotor tone is maintained by sympathetic fibers from T5 to L1 that innervate vascular smooth muscle. Blockade of these fibers cause venodilation with venous pooling as well as arterial vasodilation with decreased systemic vascular resistance. The venous pooling leads to a decrease in venous return, right atrial pressure, and subsequently, decrease in cardiac output. The decrease in venous return can also lead to an increase in cardiac vagal tone,44 especially for blocks near the T5 level.
Figure 14-8. Blood vessels in the epidural space and their communication with systemic vessels.
Figure 14-9. Segmentation of the epidural space. The yellow areas indicate various segments that may be inconsistently connected.
Clinical Pearls
Upper body vasoconstriction with baroreceptor activation can lead to increased vagal tone, causing bradycardia despite the decrease in venous return.
The compensatory mechanism for the decrease in mean arterial pressure is a reflex increase in vasoconstriction above the level of the block as well as a release in catecholamines from the adrenal medulla. If normal cardiac output is maintained either by volume loading or by physiologic mechanisms, (ie, physiologic release of catecholamines, vasoconstriction in unblocked area), the total peripheral vascular resistance will only decrease by approximately 15%, a value well tolerated by a healthy patient. In an elderly patient with cardiovascular disease, a more significant decrease in blood pressure and the resultant need for vasopressor support.
Block Above T4
The cardiovascular effects of a block above T4 are the result of a high sympathetic block. The cardiac sympathetic fibers arise from T1 to T4, and when blocked, profound hypotension (the result of a decrease in cardiac contractility) and bradycardia can occur. In addition to the cardiac effects, a high level of sympathetic blockade causes:24·45
• Increased central venous pressure without an increase in stroke volume
• Compensatory vasoconstriction in the head, neck, and upper limbs
• Splanchnic nerve blockade with reduction in medullary secretion of catecholamines
• Blockade of vasoconstrictive effect on the capacitance vessels of the lower limbs
Figure 14-10. Needle angulation required to accomplish thoracic blockade in the high thoracic/low thoracic/lumbar regions. A: High thoracic region. B: Low thoracic region. C: Lumbar region.
Anatomic Landmarks to Identify Vertebral Levels Before Epidural Injection
Anatomic Landmark | Features |
| |
C7 | Vertebral prominence, the most prominent process in the neck |
| |
T3 | Root of the spine of the scapula |
| |
T7 | Inferior angle of the scapula |
| |
L4 | Line connecting iliac crests |
| |
S2 | Line connecting the posterior inferior iliac spines |
| |
Sacral hiatus | Groove or depression just above or between the gluteal clefts above the coccyx |
When a sympathetic block occurs at such a high level, the cardiovascular system may be left without its mechanisms for responding to low cardiac output states. This can be detrimental to a patient with limited cardiac reserve because profound hypotension with bradycardia and decreased contractility can result.46 The anesthesiologist must be prepared to take over the control of the circulatory system until the block subsides and the patient stabilizes.
Respiratory
Epidural blockade to midthoracic levels have minimal effect on patients with adequate lung function. Lung volumes (tidal volume, vital capacity), resting minute ventilation, and dead space are basically unchanged even with a high thoracic epidural anesthesia. Even with abdominal or intercostal muscle paralysis by a high thoracic block, major alteration in pulmonary function is not seen.
There is concern regarding the use of epidural blockade in patients with severe chronic lung disease who may be dependent on accessory muscle function to maintain adequate ventilation. This is because paralysis of respiratory muscles and changes in bronchial tone from epidural analgesia can occur. In a study by Gruber and colleagues, thoracic epidurals were placed in patients with end-stage chronic obstructive pulmonary disease undergoing lung volume reduction surgery. Thoracic epidural analgesia with 0.25% bupivacaine did not adversely affect ventilatory mechanics, breathing pattern, gas exchange, and inspiratory muscle force generation in these patients47 (Tables 14-5 and 14-6 ).
Effects of Thoracic Epidural Analgesia on Breathing and Gas Exchange in Patients with Severe Lung Disease
Table 14-6.
Effects of Thoracic Epidural Analgesia on Breathing and Gas Exchange in Patients with Severe Lung Disease
TEA = thoracic epidural analgesia; PEEP = positive end-expiratory pressure.
Rarely, respiratory arrest during high epidural blockade has been reported. Contrary to what may seem a logical explanation, the arrest is not due to the effects of sensory or motor blockade or any effect of the local anesthetic on the brain. The reported cause of rare instances of respiratory arrest is from the sympathetic block, leading to decreased cardiac output and subsequent reduction in blood flow to the brain and brainstem ischemia.
Gastrointestinal
The gastrointestinal effects of epidural anesthesia are largely the result of blockage of the sympathetic splanchnic fibers from the T5 through LI level. Unopposed vagal dominance leads to an increase in secretions; peristalsis; and a smaller, contracted gut. Postoperatively, gastrointestinal motility returns more quickly when epidural analgesia with a local anesthetic is instituted. Several studies have demonstrated the beneficial effect of thoracic epidural anesthesia on visceral perfusion. Christopherson and colleagues used intramucosal pH measurements (pHi) as an indicator of stable visceral perfusion during abdominal surgery. They suggested that thoracic epidural anesthesia prevented the decrease of intramucosal pH during major abdominal surgery as an effect of stable visceral perfusion.48 When thoracic epidural anesthesia is used as an adjunct to general anesthesia for major thoracic, cardiac, or upper abdominal surgery, a segmental block of T1 through T5 is typically the goal. Segmental sympatholysis creating an increase of sympathetic activity in segments below the block leading to impaired splanchnic blood flow has been a concern. In a study in awake and anesthetized dogs, an upper thoracic epidural block had no compromising effect on gastrointestinal perfusion.49
Nausea is a relatively common problem following neuraxial anesthesia. It has been reported to occur in up to 20% of patients undergoing neuraxial blocks. It is thought to be related to increased gastric peristalsis secondary to unopposed vagal activity. It can be prevented by promptly treating hypotension with a fluid bolus, ephedrine, or phenylephrine. Atropine has been shown to be an effective treatment for nausea associated with a high thoracic block.50,51
Renal/Genitourinary
Since renal blood flow is maintained through autoregulation, epidural anesthesia has very little effect on renal function. Neuraxial blockade at the lumbar level has been postulated to impair control of bladder function secondarily to blockage of the S2 to S4 segments. Urinary retention may occur until the block wears off. The clinician should avoid administering an excessive volume of intravenous fluids if a urinary catheter is not in place. If a continuous epidural anesthesia/analgesia is used, then urinary catheterization may be necessary. However, more recent studies have questioned the validity of this belief.32,52
Neuroendocrine
Surgical stress produces a variety of changes in endocrine and metabolic function. Increased protein catabolism and oxygen consumption are common. Increased plasma concentrations of catecholamines, vasopressin, growth hormone, renin, angiotensin, cortisol, glucose, antidiuretic hormone, and thyroid-stimulating hormone have been documented and referred to as the surgical stress response. Perioperative manifestations of the stress response are demonstrated as hypertension, tachycardia, hyperglycemia, suppressed immune function, and altered renal function. Afferent sensory information from the surgical site is thought to play a pivotal role in the response.53 This response can be abolished or reduced by an appropriate level of sensory blockade produced by epidural anesthesia. The inhibitory effect is greatest with lower abdominal and lower extremity surgery and less effective in upper abdominal and thoracic surgery, probably because the epidural anesthesia cannot completely block all nociceptive afferent pathways for these indications.54
The most critical effect of neuroendocrine activation in the perioperative period is the increase in plasma norepinephrine, which peaks about 18 h after the surgical stimulus is initiated. The increase in plasma norepinephrine is associated with activation of nitric oxide in the endothelium of patients with atherosclerotic disease, producing paradoxic vasospasm.55 Thus, in patients with significant atherosclerotic disease, the combination of paradoxic vasospasm and the hypercoagulable state may be the factors modulated by the cardioprotective effects of thoracic epidural anesthesia and analgesia in patients with atherosclerotic cardiac disease.
PHARMACOLOGY OF EPIDURAL BLOCKADE
To be successful with epidural blockade, the clinician must understand the physiology of nerve conduction and the pharmacology of the local anesthetics. Potency and duration of the drugs, their ability to preferentially block sensory and motor fibers, as well as the anticipated duration of surgery or need for postoperative analgesia are factors that should be considered before instituting epidural blockade.
The principal site of action of local anesthetics after epidural injection is thought to be the spinal nerve roots, the spinal cord, and possibly the brain.56 Nerve fibers with different features and function display varying sensitivity to local anesthetic blockade. For example, sympathetic fibers (thin, myelinated when entering the sympathetic trunk) tend to be blocked with the lowest concentration of drug, followed by pain, touch, and finally motor fibers.
Nerve Impulse Physiology
Nerve conduction involves the propagation of an electrical impulse created by the rapid movement of ions across the nerve cell membrane, creating an action potential. The principal ions involved in generating the action potential are sodium and potassium. The concentration of sodium is high extracellularly and low intracellularly. The opposite is true of potassium (high intracellularly, low extracellularly).
At rest, the cell is more permeable to the positively charged cation potassium. The leakage of a positively charged ion leaves the inside of the cell more negative than the outside of the cell, creating a negative resting membrane potential of -60 to -70 mV. The sodium-potassium pump actively transports sodium ions out of the cell and potassium into the cell to maintain the gradient at the resting level.
Once chemical, mechanical, or electrical excitation occurs, an impulse is conducted along the nerve axon, causing depolarization of the nerve cell membrane. If the depolarization exceeds the threshold level (membrane potential of -60 mV), ion channels in the cell membranes open, allowing a sudden influx of sodium. The rapid influx of positively charged sodium ions causes depolarization of the cell. The influx of positively charged ions alters the membrane potential to become positive (above +30 mV). When the membrane potential exceeds approximately -30 mV, the sodium channels close, abating the influx of sodium into the cell. Depolarization generates a current that causes further depolarization of adjacent segments of the nerve, allowing the action potential to spread along the entire length of the nerve. The cell attempts to return to its resting potential with the efflux of potassium, thereby making the membrane potential less positive (repolarization). Baseline concentration gradients are eventually reestablished by the sodium-potassium-ATP-ase pump.
The rapid influx of sodium that leads to depolarization of the nerve occurs through specific channels in the cell membrane. The sodium channel is a path that changes the nerve from nonconductive to conductive of an action potential (referred to as gated channels). If the change in conductance is created by electrical changes, the channel is called a voltagegated channel. The voltage-gated sodium channel in the nerve is considered to be the site of action for local anesthetics.57 (For more information on mechanisms of neural blockade, please refer to Chapter 20)
Action of Local Anesthetics
Local anesthetic binds to sodium channels, primarily in the inactivated state, preventing further channel activation. Sodium ion movement into the cell is prevented, effectively blocking the development of the action potential. The resulting resting membrane potential is unaffected by further nerve stimulation, referred to as membrane stabilization of local anesthetics.
Mechanism of Action of Local Anesthetics for Neuraxial Blockade
Within the dorsal horn, local anesthetics can block both sodium and potassium ion channels in the dorsal horn neurons, inhibiting the generation and propagation of pain signals (nociceptive electrical activity). Motor blockade occurs from a similar action on the ventral horn neurons. Blockade of calcium ion channels in the spinal cord leads to resistance of electrical stimulation from nociceptive afferent nerves, creating an intense analgesic action seen in centrally administered local anesthetics.58
In addition to ion channel alterations in the central neuraxis, epidurally administered local anesthetics indirectly inhibit the release of substance P and other neurotransmitters involved in pain signal processing. Substance P is involved in pain transmission from the presynaptic terminals of dorsal root ganglionic cells. The putative effects of centrally administered local anesthetics on substance P and these other transmitters are linked to the presynaptic blockade of the voltagegated calcium channel.59,60 When calcium entry is blocked at the presynaptic level, release of these neurotransmitters (glutamate, substance P, calcitonin gene-related peptide [CGRP], neurokini-1 and-2 [NK1,NK2]) at the presynaptic level does not occur. Therefore, epidurally administered local anesthetics can indirectly inhibit pain signal transmission.
Choice of Local Anesthetics
Drugs used for epidural blockade can be categorized into short-, intermediate-, and long-acting local anesthetics. Onset of epidural blockade in the dermatomes immediately surrounding the site of injection can usually be detected within 5 or 10 min of injection of any of the local anesthetics. The time to peak effect varies with the type of local anesthetic chosen and the dose/volume administered. Table 14-7 summarizes the characteristics of the most commonly used local anesthetics for epidural anesthesia.
The shortest acting local anesthetic for neuraxial blockade is chloroprocaine (an ester). In the past, it was associated with adhesive arachnoiditis when large volumes were administered into the subarachnoid space.61 When volumes greater than 25 mL were used in the epidural space, severe back pain most likely secondary to localized hypocalcemia introduced by the preservatives, ethylenediaminetetraacetic acid (EDTA) and bisulfites, in the solution. As of 1996, preservative-free chloroprocaine has been available and has not been associated with either neurotoxic effects or back pain. In ambulatory settings, utilizing the favorable characteristics of chloroprocaine with epidural catheter insertion provides excellent surgical anesthesia without delaying recovery room discharge.
The most commonly used intermediate-acting local anesthetic for surgical anesthesia via the epidural route is 2% lidocaine. When epinephrine is added to the solution (1:200,000), it prolongs the duration of action by 40 to 60%. However, the incidence of hypotension is increased in patients receiving lumbar epidural analgesia due to the beta effect of epinephrine-containing solutions that leads to peripheral vasodilation.
Long-acting local anesthetics used for epidural blockade are 0.5% bupivacaine, 0.5% levobupivacaine, and 0.5% ropivacaine. Of these, the most commonly used agent for epidural blockade is bupivacaine. Dilute concentrations can be used for analgesia, with more concentrated solutions employed for surgical anesthesia. Epinephrine added to the solution can prolong its duration of action, but this is less consistent with the shorter acting agents. Accidental intravascular injection of bupivacaine has produced severe cardiotoxic reactions (hypotension, atrioventricular block, ventricular fibrillation) refractory to usual resuscitation methods. The rationale for the resistance to resuscitative measures lies in its high degree of protein binding and more pronounced effect on cardiac sodium channel blockade.62,63 Levobupivacaine, the S-enantiomer of bupivacaine, has nearly an identical profile to bupivacaine but without the systemic cardiac toxic effects of bupivacaine. Ropivacaine, a mepivacaine analog, also has a similar profile of action to bupivacaine. In most studies, ropivacaine has demonstrated a slightly shorter duration of action than bupivacaine, with a less dense motor block when comparing equivalent doses. The only deterrent to a wider use of ropivacaine in clinical practice is its higher cost.64,65
Etidocaine, another long-acting amide local anesthetic, is infrequently used for epidural anesthesia. It produces a profound motor block that unfortunately can outlast the sensory block,66 making it an undesirable choice for epidural anesthesia (see Table 14-7).
Onset/Duration of Local Anesthetics
Several investigators have attempted to find methods to speed up the onset or increase the duration of epidural blockade. Adding epinephrine to the local anesthetic can substantially increase the duration of action of some local anesthetics most likely by decreasing the vascular absorption. The effect is greatest with 2-chloroprocaine, lidocaine, and mepivacaine, and less effective with the longer acting agents. Other vasoconstrictors, such as phenylephrine, have not been as effective in reducing the peak blood levels of local anesthetics as epinephrine.67
Alkalinization of the local anesthetic solution has been used to increase the speed of onset of local anesthetics. By increasing the concentration of the nonionic form of the drug, more drug is available to penetrate the lipid nerve cell membranes to produce more rapid intraneural diffusion. Adding sodium bicarbonate (1 mEq/10 mL of local anesthetic) immediately before injection of lidocaine, mepivacaine, or chloroprocaine produces a clinically significant faster onset of anesthesia and may provide a more complete block.68,69 However, ropivacaine and bupivacaine will precipitate with the addition of bicarbonate unless a low concentration (0.1 mEq/10 mL of local anesthetics) is used. Combining long- and short-acting drugs to obtain a rapid onset as well as prolonged sensory block has not proven to be effective or predictably better. For example, mixing 2-chloroprocaine with bupivacaine for the rapid onset of the former and long duration of the latter resulted in shortening the duration and effectiveness of the bupivacaine.70 Utilizing the additives currently available and using continuous techniques of drug administration rather than single-shot techniques obviates the need for mixing local anesthetics.
Novel Additives to Local Anesthetics in the Epidural Space
A variety of other classes of drugs have been studied more recently to try to improve the quality of neuraxial blockade, both in the epidural space and in the subarachnoid space. In addition to a variety of opioids (eg, fentanyl, sufentanil, and preparations of morphine), α-adrenergic agonists, Cholinesterase inhibitors, semisynthetic opioid agonist-antagonists, ketamine, and midazolam have been studied with varied results.
Clonidine, the prototypical α2-adrenoceptor agonist used in neuraxial blocks, has been studied extensively in combination with local anesthetics, as a primary agent, and in combination with a variety of other drugs. Clonidine is an α-adrenoreceptor agonist with selectivity for α2-adrenoreceptors. The mechanism by which clonidine prolongs neuraxial anesthesia is unclear. Animal studies have shown that clonidine reduces regional spinal cord blood flow, therefore slowing the rate of drug elimination.71 Kroin and colleagues demonstrated that the mechanism by which clonidine prolongs the duration of a block when mixed with local anesthetics is not mediated by an α-adrenergic mechanism but is more likely related to the hyperpolarization-activated cation current (Ih)·72
Some of the benefits of the administration of clonidine in the epidural space include
1. Prolongs and intensifies the effects of epidural local anesthetics without increasing the degree of hypotension for epidural anesthesia and analgesia
2. Reduces the dose requirements of local anesthetics for labor epidural analgesia73,74
3. Produces analgesia without motor impairment and prolongs the duration of the local anesthetic analgesic effect71
4. Has a synergist effect when combined with opioids and opioid agonist-antagonists, allowing reduced doses and therefore side effects of both
5. Modulates the immune stress response to thoracic surgery75
6. Is superior in preserving preoperative lung function for thoracotomy patients76
7. May reduce cytokine response, therefore further reducing pain sensitivity77
8. Has possible antibacterial activity against Staphylococcus aureus/epidermidis
Side effects that are commonly associated with epidural clonidine include a dose-independent hypotension, bradycardia, sedation, and dry mouth. Combination of clonidine with other agents such as opioids, anticholinergics, opioid agonist- antagonists, and ketamine have been studied to enhance the beneficial effects of these drugs while limiting the adverse side effects.78,79
Neostigmine, a cholinesterase inhibitor, is a more recent addition to the list of epidural additives for selective analgesia. The mechanism of action for the analgesic effect of neostigmine appears to be the inhibition of the breakdown of acetylcholine and the indirect stimulation of muscarinic and nicotinic receptors into the spinal cord, inducing analgesia. By administering it via the epidural route, the gastrointestinal side effects (nausea/vomiting) noted with subaranchoid administration are reduced. It has been reported to provide postoperative pain relief without inducing respiratory depression, motor impairment, or hypotension.78 When combined with other opioids, clonidine, and local anesthetics, it may provide benefits similar to clonidine without the side effect profile of any of the drugs given alone.80–82
Other agents such as ketamine, tramadol, droperidol, and midazolam have been studied with various effectiveness in epidural analgesia. Considerable controversy surrounds the use of midazolam intrathecally. Despite multiple publications recommending its use intrathecally,83–85 recent studies have demonstrated that even a single dose of intrathecal midazolam may have neurotoxic effects on the neurons and myelinated axons.86 Until its safety profile can be ensured in human subjects, it is not recommended for use intrathecally or epidurally at this time.87
One agent showing promise is a new, extended release formulation of one of the oldest opioids, morphine. Epidural morphine has proven analgesic efficacy with possibly less bothersome side effects of intravenous dosing. Pain relief with single epidural injection lasts less than 24 h, requiring the institution of alternate methods to provide pain relief. Depodur, the brand name for extended-release epidural morphine, uses a drug-release delivery system called Depofoam. Depofoam is composed of microscopic lipid-based particles with internal vesicles that contain the active drug and slowly release it. Recent studies of Depofoam have demonstrated effective pain relief with relatively minor side effects for up to 48 h when appropriately dosed.88–90
Other Factors Affecting Epidural Blockade
Injection Site
The epidural blockade is most effective when the block or the catheter is inserted in a location that corresponds to the dermatomes covered by the surgical incision. The most rapid onset and the densest block occurs at the site of injection. By inserting the catheter closer to the surgical site, a lower dose of drug can be given, thereby reducing side effects.91,92 This concept is especially important when thoracic epidurals are used for postoperative analgesia.
After lumbar injection, analgesia-anesthesia spreads caudally and, to a greater degree, cranially. There is a delay at the L5 to S1 segments secondarily to the larger size of these nerve roots.93 With thoracic injection, the local anesthetic spreads evenly from the site of injection, but because of the larger nerve roots, there is greater resistance to blockade. By controlling the dose in the thoracic region, a true segmental block can be placed, affecting only the thoracic region. Lumbar and sacral regions will be spared, therefore avoiding more extensive sympathetic blockade and subsequent associated hypotension and bladder dysfunction.
Dose, Volume, and Concentration
The dose of local anesthetics necessary for analgesia or anesthesia is a function of the concentration of the solution and the volume injected. Concentration of the drug affects the density of the block. The higher the concentration, the more profound the motor and sensory block. Lower concentrations can produce a more selective sensory block.94
Volume is the variable that affects the degree of distribution of the block. A larger volume will block a greater number of segments. A generally accepted guideline for dosing epidural anesthesia in adults is 1-2 mL per segment to be blocked. This guideline should be adjusted for shorter patients or for the very tall. For example, to achieve a T10 sensory level from an L3-4 injection, approximately 9-18 mL of local anesthetic should be administered. Below concentrations of the equivalent of 1% lidocaine, motor block is minimal, regardless of the volume of the local anesthetic injected, unless doses are given at repeating intervals.57
Clinical Pearls
Volume is the key factor in determining the height of the block.
The cookbook guideline for dosing an epidural in adults is 1-2 mL per segment to be blocked.
Adjust the guideline for shorter patients (<5 ft 2 in.) or taller patients (>6 ft 2 in.).
Example: T10 block from L3-4 injection: 9-18 mL of local anesthetic.
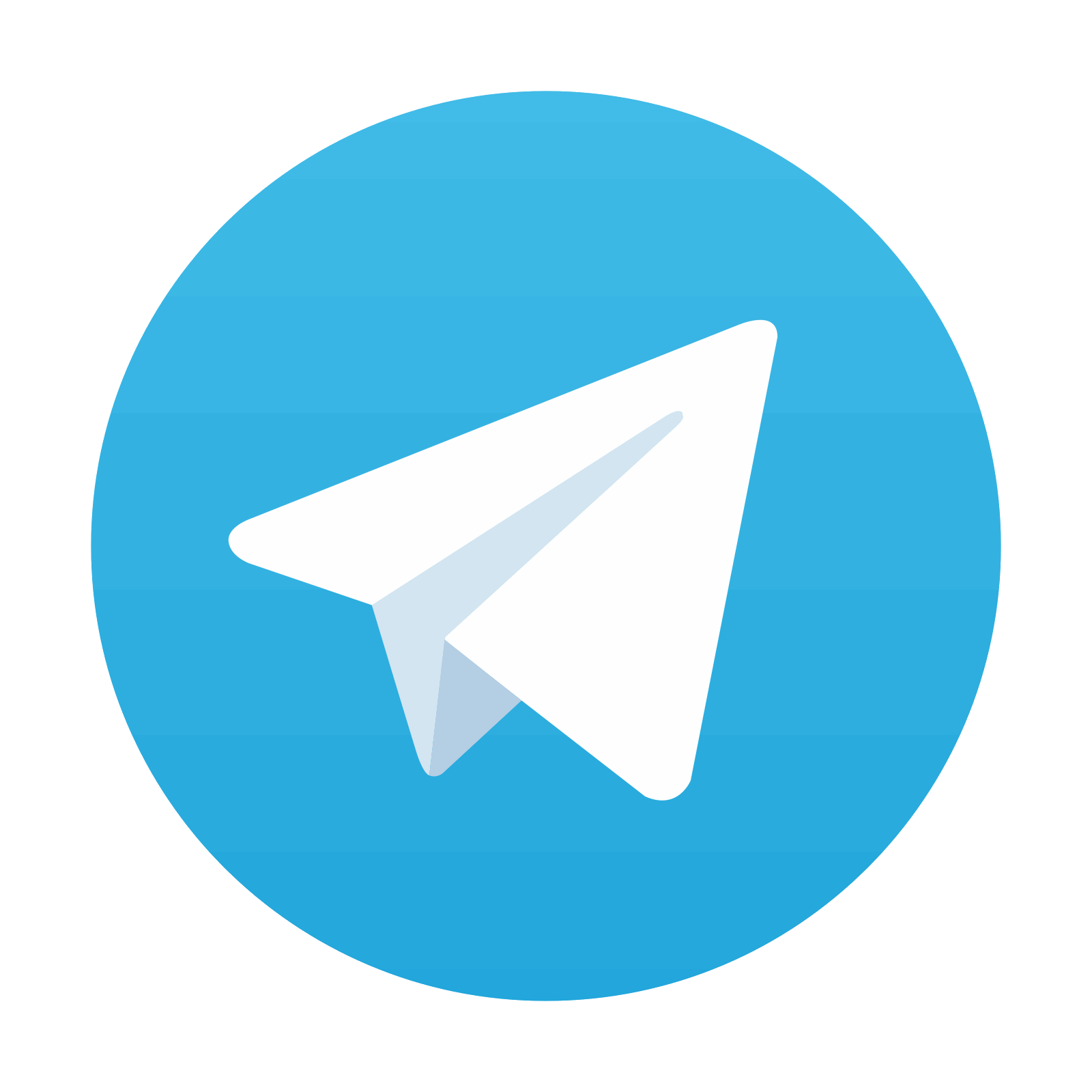
Stay updated, free articles. Join our Telegram channel

Full access? Get Clinical Tree
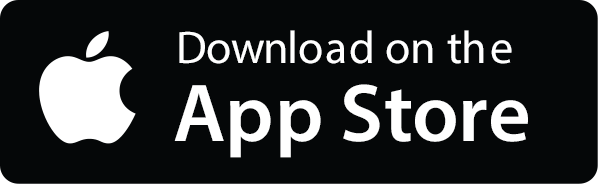
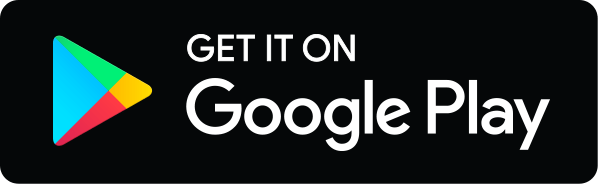