Chapter 55 Envenoming and Injuries by Venomous and Nonvenomous Reptiles Worldwide
For online-only figures, please go to www.expertconsult.com
Dangerous Reptiles
Within the class Reptilia, some alligators and crocodiles (order Crocodylia), notably Crocodilus niloticus and C. porosus, are able to kill and eat humans (see Chapter 58); even large sea turtles (order Testudines) can be dangerous. The leatherback turtle (Dermochelys coriacea), which can reach 3 m (9.8 feet) in length and 900 kg (1984 lb) in weight, is reported to have capsized small boats. Giant pythons and Komodo dragons occasionally prey on humans (see later text). Many species of the clade Toxicofera, constituting 60% of the Squamata (snakes and lizards),66 including snakes of Canada, the United States, and Mexico, and helodermatid lizards of the southern United States and Mexico are capable of venomous bites (see Chapter 54). This chapter covers dangerous snakes and lizards, venomous and nonvenomous, found in the rest of the world and therefore considered “exotic” in North America.
Venomous Snakes
Taxonomic Revolution
Snake taxonomy is based on morphologic features, such as numbers and arrangement of scales (lepidosis), dentition, osteology, myology, sensory organs, form of the hemipenes (paired male genital organs), and, increasingly, sequence analysis of DNA encoding of important mitochondrial and other enzymes.167,237,238 The accelerating pace of taxonomic revision of medically important taxa has left clinicians and nonherpetologists confused and frustrated. To change familiar names and split genera and species of medically important taxa can be misleading, even though it may be well justified on evolutionary and taxonomic grounds. Correct identification and designation of species responsible for bites is important for optimal clinical management and choice of appropriate antivenom. Therefore the latest taxonomic designations are employed in this chapter, with obsolete but familiar names in parentheses. Use of correct taxonomy is essential when designing regional antivenoms and selecting appropriate venoms for their manufacture. It is also crucial for laboratory toxinologists who work on venoms that are sometimes of uncertain provenance. Several toxins have been misnamed because of ignorance of the correct nomenclature for the source species. For instance, “ceruleotoxin” was named after Bungarus caeruleus, although its true origin was Bungarus fasciatus. “Grambin” and “graminelysin” were named after Trimeresurus gramineus, but were obtained from Viridovipera (Trimeresurus) stejnegeri. In an attempt to improve the flow of information between taxonomists, toxinologists, and clinicians, an important series of reviews was published in Toxicon,295,296 succeeded by an invaluable website (http://www.bangor.ac.uk/~bss166/update.htm). Some published work on toxins can never be reproduced because the venoms used, their origins shrouded in incorrect snake nomenclature, can never be identified.297
Fortunately, nomenclature and identification have been clarified for several groups of medically important snakes: African spitting cobras,294,299 Afro-Asian saw-scaled vipers,161 Asian cobras,293,294,298,301 Russell’s vipers,301 arboreal pit vipers,132,178 and Bothrops atrox.300 Many of the most important causes of snakebite in each country have been identified and their geographic distributions mapped (http://www.who.int/bloodproducts/snake_antivenoms/en/). New species of both clinical and toxinologic interest continue to be discovered, for example New Guinea small-eyed snake (Micropechis ikaheka),270 Indo-Chinese spitting cobra (Naja siamensis),301 hump-nosed pit viper (Hypnale hypnale) in India,101,129 the greater black krait (Bungarus niger) in Bangladesh,57 Wall’s kraits and the Sind krait in Nepal and Bangladesh,113 and the southern African swamp adder (Proatheris superciliaris).259
Based on their nuclear and mitochondrial genes, advanced snakes (Caenophidia), including all venomous snakes, are now classified into three superfamilies:237,238
Identification of Venomous Snakes
Distinguishing Snakes from Legless Lizards, Amphibians, and Snake-Like Fish
Most lizards have eyelids, external ear orifices (Figure 55-1, Pogona barbata), thick and fleshy tongues that are forked and snake-like in Varanidae, Teiidae, Lacertidae, and Anguidae, and very long friable tails; they lack the enlarged ventral scales of snakes. Legless lizards include slow worms and glass lizards (family Anguidae), worm-like geckos (family Pygopodidae) (Figure 55-2, Lialis jicari), and skinks (family Scincidae), some of which have vestigial limbs (Figure 55-3, Chalcides armitagei) and may be confused with snakes unless these features are detected. The fossorial amphisbaenid lizards have worm-like annular grooves along the length of their bodies (Figure 55-4, Amphisbaena fuliginosa; Figure 55-5, A. mertensii). Caecilians (legless amphibians) (Figure 55-6, Dermophis mexicanus) have no obvious eyes or scales. Eels and pipe-shaped fish are easily distinguished by their gills and fins from snakes.

FIGURE 55-3 Armitage’s cylindrical skink (Chalcides armitagei: family Scincidae) (The Gambia) showing vestigial limbs.
(Copyright D.A. Warrell.)

FIGURE 55-5 Mertens’ worm lizard (Amphisbaena mertensii: family Amphisbaenidae) (Brazil).
(Copyright D.A. Warrell.)
Distinguishing Venomous from Nonvenomous Snakes
There is no quick, simple, and absolutely reliable method for distinguishing venomous from nonvenomous snakes. The fangs may be very small in elapids, or they may be folded back inside a sheath in vipers (Figure 55-7, Bothrops atrox). In a dead snake, a needle may be swept along the upper jaw from the angle of the jaw to the snout to engage upon and reveal the fangs. Some key morphologic features are subtle. Most elapid snakes lack the loreal scale (between the preocular and nasal scales) possessed by most colubroid snakes (Figure 55-8, Dendroaspis angusticeps and Philodryas viridissimus). Pit vipers (Crotalinae) possess a heat (infrared)-sensitive pit organ, situated between the eyes and nostrils, whose functions include heat regulation and detection of warm-blooded prey and predators (Figure 55-9, A, Popeia popeiorum). Pythons and boas have labial heat-sensitive organs (Figure 55-9, B, Morelia viridis). Snakes of the subfamily Viperinae, the Old World vipers and adders, lack this pit organ.
Fortunately, the most dangerous species tend to be well known in the areas where they are medically important. The characteristic hood of cobras and some other elapids is visible only when the snake is rearing up in a defensive attitude (Figure 55-10, Naja mossambica). Vipers and pit vipers are often identifiable by their striking and sometimes colorful dorsal pattern (Figure 55-11, Bitis arietans; Figure 55-12, Deinagkistrodon acutus). Russell’s vipers (Daboia russelii) (Figure 55-13, A), Daboia siamensis (Figure 55-13, B), and puff adders (B. arietans) (see Figure 55-11) hiss loudly by expelling air through their large nostrils. The saw-scaled or carpet vipers (genus Echis) and some other desert vipers produce a characteristic rasping sound by rubbing their coils together (Figure 55-14, A, Echis pyramidum, and Figure 55-14, B, Cerastes cerastes), and king cobras (Figure 55-15, Ophiophagus hannah) are said to “growl.” The tail tip series of interlocking and resonating modified scales of rattlesnakes produce their familiar castanet-like warning sound (Figure 55-16, Crotalus durissus cumanensis). However, in a world increasingly densely inhabited by humans, this sound may no longer be protective to these snakes but rather attract lethal attention. There is some evidence that natural selection now favors rattlesnakes that are more reticent about sounding their rattles. Less spectacularly, several genera of South American pit vipers (Agkistrodon, Bothrops, Lachesis), especially their juveniles, vibrate the tips of their tails when aroused, producing a whirring sound in the undergrowth.

FIGURE 55-11 Puff adder (Bitis arietans) (South Africa) showing the distinctive repeated U (chevron) dorsal pattern.
(Copyright D.A. Warrell.)

FIGURE 55-12 Hundred-pacer (Deinagkistrodon acutus) (China) showing the striking dorsal pattern.
(Copyright D.A. Warrell.)

FIGURE 55-16 South American rattlesnake (Crotalus durissus cumanensis) showing rattle (Colombia).
(Copyright D.A. Warrell.)
Mimics, Misidentification, and Mismanagement
Some nonvenomous or less-venomous colubroid snakes closely resemble highly venomous species in their appearance and behavior. This is probably an example of Batesian mimicry. There are examples from all continents: in Europe, Hemorrhois nummifer (coin-marked snake) mimics Macrovipera lebetina (Levantine viper) (Figure 55-17); in Africa, Telescopus variegatus (tiger snake) (Figure 55-18, A) and Dasypeltis scabra (egg-eating snake) (Figure 55-18, B) mimic Echis spp. (saw-scaled viper) (Figure 55-18, C); in Southeast Asia, Boiga multomaculata (Figure 55-19, A) mimics D. siamensis (Eastern Russell’s viper) (Figure 55-19, B), various species of Dryocalamus (e.g. D. davisonii [Figure 55-20, A]) and Lycodon (e.g., L. aulicus Figure 55-21, A) mimic kraits (Bungarus candidus [Figure 55-20, B] and B. caeruleus [Figure 55-21, B]); and in the Americas, Dipsas (Figure 55-22, A, D. indica), Xenodon (Figure 55-22, B, Xenodon neuwiedii; Figure 55-22, C, X. severus), Waglerophis merremi (Figure 55-22, D), and many others mimic Bothrops species (Figure 55-22, E, B. brazili). The best known examples are mimics of coral snakes (Micrurus) (Figure 55-23, A to E: M. corallinus, M. decoratus, M. frontalis, M. mipartitus, M. spixii obscurus), which include Oxyrhopus, Rhinobothryum, and Simophis spp. (Figure 55-24). The familiar jingle “red on yellow kills a fellow, red on black venom lack (or, “OK Jack”)” safely distinguishes North American coral snakes (Micruroides euryxanthus, Micrurus fulvius, M. tener [Figure 55-25]) from harmless scarlet snakes (Cemophora coccinea), king snakes, and milk snakes (Lampropeltis spp.), but in Central and South America this does not apply, because many species of Micrurus have “red on black” (see Figure 55-23).
Similarities between the superficial appearances of venomous and nonvenomous species, or even between different venomous species, may lead to choice of the wrong antivenom or inappropriate use of antivenom. For example, in a study of 860 snakebites in Sri Lanka,9 snakes were misidentified by the hospital staff on 51 occasions, resulting in unnecessary use of antivenom. Hump-nosed pit vipers (Hypnale hypnale, Figure 55-26) were misidentified as Russell’s vipers (Daboia russelii, see Figure 55-13, A) on 36 occasions; cat snakes (Boiga ceylonensis [Figure 55-27], and B. trigonata trigonata) were misidentified as hump-nosed pit vipers twice, as Russell’s vipers three times, and as a saw-scaled viper (Echis carinatus, Figure 55-28) once; pythons were misidentified as Russell’s vipers twice; wolf snakes banded phase (L. aulicus [see Figure 55-21, A]), and L. striatus sinhalayus) were misidentified as common kraits (B. caeruleus) five times and rat snakes (Ptyas mucosus) as cobras twice. Antivenom was given inappropriately on 13 occasions because a nonvenomous species or a hump-nosed viper had been mistaken for one of the four species covered by Indian polyspecific antivenom. In a study of 1631 snakebites in Thailand,239 Oligodon dorsolateralis was mistaken for Calloselasma rhosostoma twice and for D. siamensis once, common kukri snakes (O. cyclurus) for C. rhodostoma on six occasions, B. multomaculata (see Figure 55-19, A) for D. siamensis on seven occasions, Lycodon laoensis for B. candidus once, Rhabdophis subminiatus for C. rhodostoma once, and Dryocalamus davisonii (see Figure 55-20, A) for B. candidus (see Figure 55-20, B) or B. fasciatus (see Figure 55-20, C) on four occasions. Bungarus candidus was confused with B. fasciatus on five occasions with the result that inappropriate monospecific B. fasciatus antivenom was given.

FIGURE 55-25 Texas coral snake (Micrurus tener) (Kingsville, Texas) showing “red on yellow” pattern.
(Copyright D.A. Warrell.)

FIGURE 55-26 Sri Lankan hump-nosed pit viper (Hypnale hypnale: family Viperidae; subfamily Crotalinae; scale in cm).
(Copyright D.A. Warrell.)
Fangs and Venom Apparatus*
Colubroidea: Natricidae, Colubridae, Dipsadidae
Venom from supralabial (Duvernoy’s) glands is inoculated by enlarged posteriorly situated (opisthoglyphous) maxillary teeth that can be formidably long (hence the term “rear-fanged snake”) (Figure 55-29, Tomodon dorsatus). These teeth may have anterior grooves or be solid. To envenom a human, the snake must seize, hold onto, and chew the finger of its victim, who is often a herpetologist. Duvernoy’s glands are surrounded by muscles (adductores superficialis, medialis and profundus and retractor quadrati that may help to express the secretions).
Elapoidea: Lamprophiidae, Subfamily Atractaspidinae
The genus Atractaspis (African and Middle Eastern burrowing asps, stiletto snakes, burrowing or mole vipers or adders, false vipers, or side-stabbing snakes) have long front fangs upon which they impale their victims by a side-swiping motion of the fang protruding from the corner of the partially closed mouth (Figure 55-30, Atractaspis aterrima). In some species, the venom glands are very long—perhaps one-sixth the snake’s total length. The Natal black snake (Macrelaps microlepidotus) possesses two very large grooved opisthoglyphous fangs at the posterior ends of its maxillae.
Elapoidea: Elapidae
Cobras—Naja; kraits—Bungarus; mambas—Dendroaspis; shield-nosed and African coral snakes—Aspidelaps; Asian coral snakes—Calliophis; African garter snakes—Elapsoidea; terrestrial venomous Australasian snakes—for example, Acanthophis, Pseudechis, Pseudonaja, Oxyuranus, Notechis; and sea snakes—for example, Laticauda, Enhydrina, Hydrophis, and Lapemis, have relatively short, proteroglyphous (fixed) front fangs mounted on a relatively fixed maxilla so that they cannot be folded flat against the jaw (Figure 55-31, Oxyuranus scutellatus canni). However, mambas can rotate their fangs in the sagittal plane because the maxilla has a posterior articulation with the prefrontal bone (Figure 55-32, Dendroaspis angusticeps). This allows the snake to close its mouth despite having unusually long elapid type of fangs and to increase its gape when striking at large prey. Venom glands are situated behind the eyes and are compressed by adductor externus superficialis (equivalent to levator anguli oris) muscles. Some coral snakes possess inferolabial glands in the lower jaw that also secrete venom conducted by shallow grooves in the mandibular teeth.

FIGURE 55-31 Short front fangs of a Papuan taipan (Oxyuranus scutellatus: family Elapidae) (Port Moresby, Papua New Guinea).
(Copyright D.A. Warrell.)
Spitting Elapids.41
The fangs of African and Asian spitting cobras and the South African ringhals or rinkhals (Hemachatus haemachatus) have venom channels that turn forward at their point of exit near the tips of the fangs. Compressor muscles produce a stream of venom under high pressure so that a spray of venom can be ejected forward for a meter or more into the eyes of an aggressor (Figure 55-33, Naja nigricollis).23,41,298,299 This is a defensive strategy that temporarily blinds the aggressor, usually a large, threatening mammal, thus allowing the snake to escape. To eject venom 2 m, pressures of 1.5 kg/cm2 (21.3 psi)would be required.
Viperoidea
Viperidae (vipers, adders, and pit vipers) have highly evolved, long, curved front solenoglyphous (hinged, venom-conducting) fangs, mounted on a rotatable maxilla so that they can be erected when the snake strikes and folded flat against the jaw in a protective membrane when not in use. They contain a closed venom channel and resemble hypodermic needles (Figure 55-34, A, Bothrops jararacussu, and Figure 55-34, B, Bitis rhinoceros). The venom glands are squeezed by compressor glandulae, adductor externus profundus, and pterygoideus muscles.
Venom Composition*
Snakes have evolved the most complex of all venoms, the venom of each species containing more than 100 different components. The evolutionary origins of these molecules are of great current interest.63,69 More than 90% of the dry weight is protein, comprising a variety of enzymes, nonenzymatic polypeptide toxins, and nontoxic proteins. Nonprotein ingredients include carbohydrates and metals (often part of glycoprotein metalloprotein enzymes), lipids, free amino acids, nucleosides, and biogenic amines such as serotonin and acetylcholine.
Enzymes
Eighty percent to 95% of viperid and 25% to 70% of elapid venoms consist of enzymes (molecular weights 13 to 15 kDa), including digestive hydrolases (proteinases, exopeptidase, endopeptidases, phosphodiesterases, metalloproteinases, and phospholipases), hyaluronidase,106,165,166 and activators or inactivators of physiologic processes. Most venoms contain L-amino acid oxidase (containing riboflavin 5′-phosphate prosthetic group that confers the yellow color of many venoms), which may have a digestive function,208 phosphomonoesterase, diesterase, 5′-nucleotidase, deoxyribonuclease, nicotinamide adenine dinucleotide nucleosidase,49 phospholipase A2 (PLA2), and peptidases. Elapid venoms in addition contain acetylcholine esterase,3 phospholipase B, and glycerophosphatase, whereas viperid venoms have endopeptidase, arginine ester hydrolase, kininogenase (which releases bradykinin from bradykininogen), and procoagulants—thrombin-like serine proteinases126 and factor V, factor X, and prothrombin-activating enzymes, which cause disseminated intravascular coagulation leading to consumption coagulopathy. PLA2s are the most widespread and extensively studied of all venom enzymes.50,128 Under experimental conditions, they damage mitochondria, red blood cells, leukocytes, platelets, peripheral nerve endings, skeletal muscle, vascular endothelium, and other membranes, and produce presynaptic neurotoxic activity, opiate-like sedative effects, and autopharmacologic release of histamine. Hyaluronidase promotes the spread of venom through tissues. Proteolytic enzymes (endopeptidases or hydrolases) are responsible for local changes in vascular permeability, leading to edema, blistering, bruising, and necrosis. Metalloproteinases cause local and systemic hemorrhage and local myonecrosis, blistering, and edema through their actions on vascular endothelium, platelets,102 muscle, and other tissues.59,79
Necrotoxins
Many snake venoms contain myotoxic and cytolytic factors that contribute to tissue destruction around the site of the bite. These include zinc-dependent metalloproteinases and myotoxic PLA2s, other digestive hydrolases, and hyaluronidase. Myotoxins are proteins that can damage the muscle cell plasma membrane directly. Most are PLA2s, either enzymatically active (aspartate-49) or enzymatically inactive (lysine-49).78 Cobra cardiotoxins are low-molecular-weight polypeptides with cytotoxic action.
Neurotoxins
Postsynaptic Three-Finger-Fold Neurotoxins†
These polypeptide curarimimetic or α-neurotoxins are low-molecular-weight, nonenzymatic proteins found in elapid and some colubroid venoms.64,127,156 They are termed “short” (60 to 62 amino acids) or “long” (66 to 74 amino acids, such as α-bungarotoxin and cobrotoxin). They bind to the nicotinic acetylcholine (ACh) binding sites of motor endplates of skeletal muscles, at the interfaces between the α/ε and the α/δ subunits of the ACh receptor, thereby preventing the interaction between ACh and the receptor, to cause a rapidly developing neuromuscular paralysis and eventually death from bulbar and respiratory muscle weakness. They have a distinctive “three-finger-fold” structure, complementary in shape to their receptor, and have also been found in some colubrid venoms.
Mamba Toxins
Mamba (Dendroaspis) venoms contain a number of distinctive neurotoxins.85,141 Dendrotoxins (7 kDa, 57-60 amino-acid proteins), such as α-dendrotoxin from eastern green mamba (Dendroaspis angusticeps) (see Figure 55-8) venom, which binds to some classes of voltage-gated potassium channels at nerve endings to cause polarizing block by ACh release, and calcicludine, which blocks calcium channels, are “pear”-fold structures. Two “three-finger-fold” neurotoxins are unique to mamba venoms. Fasciculins (61-amino-acid residues) inhibit some acetylcholinesterases, causing persistent muscle fasciculations,86 whereas calciseptine binds to calcium channels.
Cardiovascular Toxins
ACE Inhibitors and Bradykinin-Potentiating Peptides
Oligopeptides (molecular weight 600-1,600 Da) from venom of the Brazilian jararaca (Bothrops jararaca) activate bradykinin and, through a bradykinin-potentiating peptide (BPP) activity, prolong bradykinin’s hypotensive effect by inactivating the peptidyl dipeptidase that destroys bradykinin and converts angiotensin I to angiotensin II. This provided the structural model for captopril, first of the synthetic angiotensin-converting enzyme (ACE) inhibitors. BPPs and ACE-inhibiting peptides have been found in several other crotaline and viperine venoms.51
Venom Potency and Lethality
The activities of different venoms can be compared in the laboratory using in vitro and animal in vivo assays. The only practicable index of lethality is the median lethal dose (LD50) measured in mice of specified strain and size (Table 55-1). However, this value cannot be extrapolated easily to give a reliable idea of the lethal dose or even the relative lethality of different venoms in humans. Quite apart from the marked species variations in vulnerability to venoms, the mode of death in different animals may not be the same. For example, in mice, venom of the black-necked spitting cobra (N. nigricollis) causes death with convulsions following paralysis (intravenous LD50 0.40 to 1.37 mg/kg), but humans envenomed by this species do not show neurotoxicity.222,269 A series of standard World Health Organization (WHO) assays for assessing different aspects of venom activity has been developed.212 It is hoped that an assay in insensate chick embryos189 and increasing use of tissue culture assays may reduce the need for studies in living animals.
Variations in the Quantity and Composition of Venom Injected by a Snakebite
In human victims of snakebite, the amount and composition of venom injected by the snake are the most important, but indeterminate, factors influencing evolution of envenoming and prognosis. Apart from inferring the quantity and quality of injected venom from the range and severity of clinical manifestations of envenoming, the only currently available objective method of assessment is to measure venom antigenemia when the patient is admitted to the hospital. This has been found to correlate with clinical severity in the case of several different species of snake, including European vipers,13 Burmese Russell’s viper,233 Martinican lancehead (Bothrops lanceolatus),220 jararaca (B. jararaca),61 and common lancehead (B. atrox).197
Box 55-1 summarizes the factors that determine severity of envenoming. Clinical assessment suggests that the amount of venom injected at a bite is very variable. A proportion of human victims of snakebite, more than one-half in some series, show negligible or no signs of envenoming, even though the snake was identified as being venomous and there were obvious puncture marks indicating that the fangs had penetrated the skin.148,159 These so-called “dry bites” have led to the belief that snakes can somehow control the amount of venom injected, according to the size of their natural prey,88 and that they can inflict an intentionally “defensive bite,” choosing not to expend venom when they strike at an enemy. However, when humans are bitten, the usual circumstances of the bite, inadvertently picking up or stepping on a snake in foliage, may mitigate against mechanical efficiency of the snake’s venom apparatus in injecting venom, because humans are unexpected and abnormal targets. The idea that a snake might exhaust its supply of venom by a recent strike and therefore be less venomous after killing and swallowing its prey or after striking another person has been refuted. Snakes do not exhaust the contents of their venom glands during the first strike. The proportion of unexpended venom after the first strike was found to be 0% to 85% for the Australian death adder (Acanthophis), 5% to 81% for Australian tiger snakes (Notechis), 40% for the Indian cobra (Naja naja), and 30% for the saw-scaled viper (E. carinatus).112 Palestine vipers (Daboia palaestinae) continue to deliver substantial quantities of venom after nine or more consecutive strikes.112 In several reports of consecutive strikes by a single snake on several human victims, the first victim was not always the most severely envenomed. In the case of Russell’s viper bites in Burma (Myanmar), snakes that had recently fed (those in which a rodent was found in their stomachs) inflicted bites that were no less venomous than those with empty stomachs.233 Although the largest snakes do not always inflict the most highly venomous bites, there is a tendency, in most species that have been studied, for the severity of local and systemic envenoming to increase with the size of the individual snake responsible.233 Venom yield has been shown to increase with ambient temperature, but no definite seasonal variation has been documented.
BOX 55-1 Determinants of Snakebite Incidence and Severity of Envenoming
Intraspecies Variation in Venom Composition*
In many snake species, venom composition has been found to change as the snake grows older, presumably to accommodate a changing range of prey species. Most of the species that have been studied are from the Americas (see Chapter 54), but in the rest of the world, Indian cobra (Naja naja) venom has been shown to have decreasing lethality with increasing age, whereas venoms of the saw-scaled viper (E. carinatus) and black-necked spitting cobra (N. nigricollis) show decreasing lethality despite increasing protein concentration.252 Seasonal variation in the venom composition of a particular species has been suspected but not proved. There is now abundant literature describing variations in venom lethality, composition, and activities between individuals of a particular species at the same geographic focus or from different places within the snake’s geographic range.45,162,252 In the case of the Malayan pit viper (Calloselasma rhodostoma),45 Echis spp.,17,33 and Sistrurus spp.,73 available prey species seemed to have had a major evolutionary influence on venom composition in different parts of its geographic range.
Effect of the Site and Depth of the Bite
In experimental animals, the route of venom administration (topical, intradermal, subcutaneous, intramuscular, intraperitoneal, intravenous, intracerebral) affects the speed, pattern, and lethality of envenoming. This is virtually impossible to study clinically, but in anecdotal cases of human envenoming, in which systemic effects develop rapidly after a bite with minimal local signs, it has been assumed that venom entered the bloodstream directly as a result of a fortuitous intravenous puncture (Figure 55-35, online) (Bothrops atrox bite).
Epidemiology of Snakebite
Snake Species Responsible for Most Bites
Table 55-2 lists the species responsible for most snakebite deaths and severe morbidity outside North America. Fortunately, some species notorious for the potency of their venom (e.g., sea snakes and the reclusive Australian inland taipan, Oxyuranus microlepidotus) or their great size (e.g., the south Asian king cobra, O. hannah [see Figure 55-15], which can grow longer than 5.8 m [19 feet]; Gaboon vipers, Bitis gabonica and B. rhinoceros [see Figure 55-34, B], which may exceed 2 m [6.6 feet] in total length and weigh more than 8 kg (17.6 lb); and bushmasters (genus Lachesis) that grow to more than 4 m [13 feet]) (Figure 55-36, Lachesis stenophrys) rarely bite humans. African night adders and burrowing asps (genera Causus [Figure 55-37] and Atractaspis [Figure 55-38]),276 Asian green pit vipers (e.g., genera Cryptelytrops [Figure 55-39], Trimeresurus, Viridovipera [Figure 55-40])96,239 and Sri Lankan and southwest Indian hump-nosed pit vipers (H. hypnale)12,48,101 are responsible for many bites, but severe envenoming is rare or uncommon.
TABLE 55-2 Species of Snake Probably Responsible for Most Deaths and Morbidity Outside North America and Mexico
Geographic Area | Scientific Name | Common Name |
---|---|---|
Europe | Vipera and Macrovipera spp. | Vipers, adders |
Vipera ammodytes | Long-nosed viper | |
Africa | Naja nigricollis, N. mossambica, etc. | African spitting cobras |
Naja haje, N. annulifera, N. anchietae | Egyptian and snouted cobras | |
Naja senegalensis | ||
Dendroaspis spp. | Mambas | |
Echis ocellatus, E. jogeri, E. leucogaster, E. pyramidum, etc. | Saw-scaled or carpet vipers | |
Bitis arietans | Puff adder | |
Asia, Middle East | Naja oxiana | Oxus cobra |
Echis spp. | Saw-scaled or carpet vipers | |
Macrovipera lebetina | Levantine viper | |
Daboia palaestinae | Palestine viper | |
Asia, Indian Subcontinent, and Southeast Asia | Naja naja, N. kaouthia, N. siamensis | Asian cobras |
Bungarus spp. | Kraits | |
Daboia russelii, D. siamensis | Russell’s vipers | |
Calloselasma rhodostoma | Malayan pit viper | |
Echis carinatus | Saw-scaled or carpet vipers | |
Hypnale hypnale | Hump-nosed pit viper | |
Cryptelytrops, Trimeresurus, and related genera | Arboreal and other pit vipers | |
Asia, Far East | Naja atra | Chinese cobra |
Bungarus multicinctus | Chinese krait | |
Protobothrops flavoviridis | Japanese habu | |
Protobothrops mucrosquamatus | Chinese habu | |
Gloydius blomhoffii and G. brevicaudus | Mamushis | |
Australasia, New Guinea | Acanthophis spp. | Death adders |
Pseudonaja spp. | Brown snakes | |
Pseudechis spp. | Black snakes | |
Notechis spp. | Tiger snakes | |
Oxyuranus scutellatus | Taipan | |
Micropechis ikaheka | New Guinean small-eyed snake | |
Central, South | Micrurus spp. | Coral snakes |
America | Bothrops atrox | Common lancehead |
Bothrops asper | Terciopelo | |
Bothrops jararaca | Jararaca | |
Bothrops bilineatus (Bothriopsis bilineata) | Papagaio | |
Agkistrodon, Bothriechis, Porthidium, and related genera | Cantils and other pit vipers | |
Lachesis spp. | Bushmasters, surucucu | |
Caribbean | Micrurus lemniscatus, M. circinalis | Coral snakes (Trinidad) |
Bothrops asper | Mapepire (Trinidad) | |
Bothrops caribbaeus | Serpent (St Lucia) | |
Bothrops lanceolatus | Fer de lance (Martinique) | |
Crotalus durissus | Colebre (Aruba), Islas Los Testigos | |
Lachesis muta | Mapepire zanana (Trinidad) |

FIGURE 55-37 Velvety-green night adder (Causus resimus: subfamily Viperinae) (Watamu, Kenya).
(Copyright D.A. Warrell.)

FIGURE 55-38 Burrowing asp (Atractaspis fallax: subfamily Atractaspidinae) (Watamu, Kenya).
(Copyright D.A. Warrell.)

FIGURE 55-39 White-lipped green pit viper (Cryptelytrops albolabris: subfamily: Crotalinae) (Thailand).
(Copyright D.A. Warrell.)
Distribution of Venomous Snakes
Venomous snakes are distributed from sea level up to high altitudes. The Himalayan pit viper, Gloydius himalayanus, normally confined below 1500 m (4921 feet), has been recorded at the base of the Dharamsala glacier in northern India as high as 4877 m (16,000 feet),187 and the rattlesnake (Crotalus triseriatus triseriatus) (Figure 55-41) has been found on Mt Popocatepetl and Ajusco and Volcán Pico de Orizaba in Mexico at altitudes up to 4600 m (15,092 feet).110 The European adder, Vipera berus, is very widely distributed, from England, Scotland, and Wales in the west, across Europe and Asia to North Korea and Sakhalin Island in the east, and north into Scandinavia and the Arctic Circle (Figure 55-42, online). No other venomous species is found in the Arctic, and none occurs in the Antarctic, Crete, Ireland, Iceland, Madagascar, New Caledonia, New Zealand, and Hawaii. Most of the islands of the western Mediterranean Sea and Atlantic and Pacific Oceans are free of venomous snakes (Figure 55-43, online).
Sea snakes occur in the Persian Gulf and Indian and Pacific Oceans between latitudes 30 degrees north and 30 degrees south, as far south as New Zealand and Tasmania, and as far north as Siberia (yellow-bellied or pelagic sea snake Pelamis platurus) (Figure 55-44, online).137,172,248 The beaked sea snake (Enhydrina schistosa) and Hydrophis species frequent estuaries and have been found 130 to 160 km (80 to 100 miles) up rivers. In Papua New Guinea, E. schistosa was taken in the Ramu River, 30 km (18.6 miles) from the sea, where it had caused fatal envenoming (Figure 55-45). In freshwater lakes, Hydrophis semperi occurs in Lake Taal (also called Lake Bombon) (Figure 55-46) in the Philippines and E. schistosa in Tonle Sap in Cambodia. There are no sea snakes in the Atlantic Ocean or the Red Sea.248
Snakebite Mortality and Morbidity
In the tropical countries where snakebite is most common, there are few reliable data. There have been only three serious attempts to assess global snakebite mortality.38,105,207 However, community-based studies have revealed gross underreporting of deaths in these studies. One reason is that records of patients treated by traditional methods are missing from official hospital-based statistics, and deaths reported at the hamlet or district level may not be sent on to ministry headquarters. Population surveys* have given a far more accurate picture of snakebite incidence, morbidity, and mortality. The prevalence of chronic morbidity following snakebite is unknown, but it is possible that, for every person killed by snakebite, one or two may survive with a long-term physical handicap.
Americas (Central, South, and the Caribbean)
Estimates of the total number of deaths caused by snakebites each year in Central and South America (including Mexico) were as follows: in 1954, between 3000 and 4000207; in 1998, 5000 out of 300,000 bites38; and in 2008, 3300 to 4500 deaths out of 81,427 to 137,123 bites.105 The incidence is high among indigenous Amerindian tribes. In Acre, western Brazil, snakebite accounted for 3.3% of all deaths and 24% of deaths in the 15- to 44-year-old age-group among 100 families of Kaxinawa of the Rio Tejo, Upper Juruá rubber extraction area.24 A survey of forest-dwelling Amazonian Indians and rubber tappers of the Juruá Valley found that 30% of the population had been bitten during their lifetime. Mortality was estimated at about 400 deaths per 100,000 population per lifetime.160
Brazil
At the beginning of the 20th century, Vital Brazil estimated that, among 19,200 probable bites in Brazil, there were 4800 deaths. In 1949, 2000 deaths were estimated.207 During the period 1990 to 1993, there was an average of 27,200 cases per year with 120 deaths per year, the overall case fatality being 0.4%. In 2005, 28,711 snakebites were reported (15/100,000 population) with 114 deaths (Secretaria de Vigilância em Saúde).
Asia
Estimates of snakebite deaths in Asia range between 15,000 and 100,000/year,40,105 reflecting the difficulty in assessing an occupational/environmental disease that exacts so much of its mortality outside hospitals. The highest recorded incidence was 162 deaths per 100,000 population per year, determined in the Eastern Terai area of Nepal.193 In this study, only 20% of deaths occurred in hospitals. Increased risk for fatality was associated with being bitten inside the house while resting between midnight and 6:00 AM, which suggests bites by the common krait (B. caeruleus) (see later discussion). Other risk factors were an initial visit to a traditional healer and delayed transport to the hospital. In Bardhaman (Burdwan) District, West Bengal, a field survey in randomly selected villages suggested that among the total population of nearly 5 million people, nearly 8000 were bitten and 800 killed by snakes each year.87
Bangladesh
Nineteen thousand people in nearly 4000 randomly selected households throughout the country provided information about snakebites. National totals of approximately 600,000 bites and approximately 6000 deaths per year were calculated by extrapolating to the total population of rural Bangladesh.168
India
From 1869, when Joseph Fayrer first solicited data from “British India,” around 20,000 human deaths each year were attributed to snakebite. Based on a nationally representative study of 128,000 deaths in 6671 randomly selected areas of India from 2001 to 2003, a total of about 46,000 snakebite deaths was estimated for 2005.145
Japan
In the Amami and Okinawa Islands of Japan, there were 5488 bites by the habu (Protobothrops [Trimeresurus] flavoviridis), resulting in 50 deaths during the 9 years from 1962 to 1970.184 The highest incidence of bites on one of the islands was 4.6 per 1000 population per year.
Africa
There are probably more than 100,000 envenomings and 5000 deaths in Africa each year. However, Chippaux38,39 has estimated fivefold higher figures of 1 million bites, 500,000 envenomings, and 20,000 deaths.
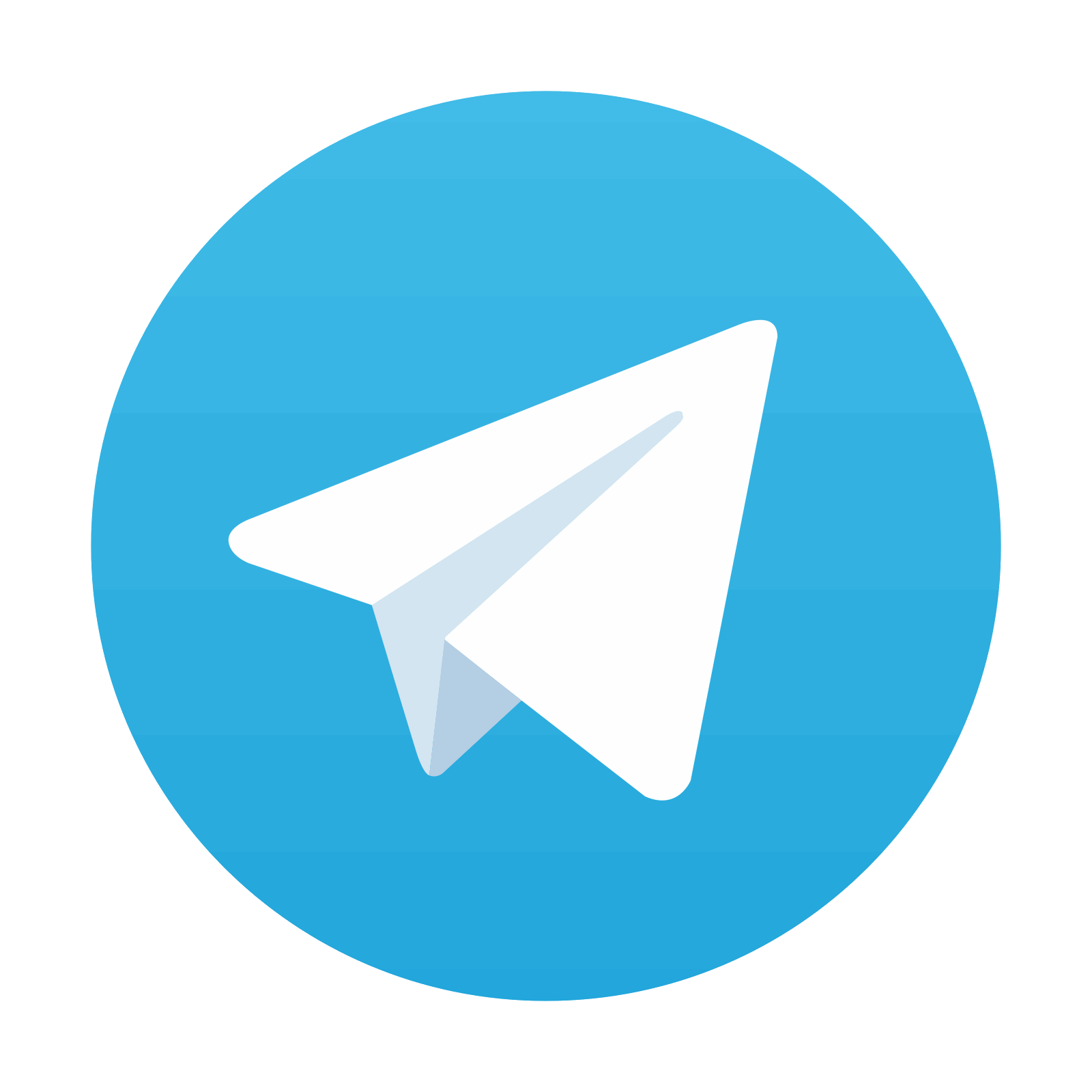
Stay updated, free articles. Join our Telegram channel

Full access? Get Clinical Tree
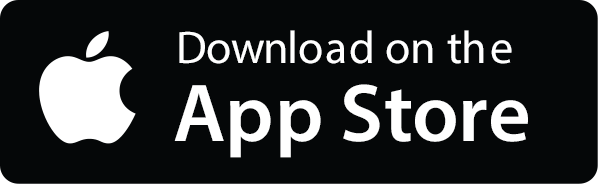
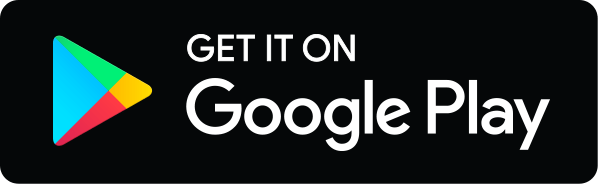