Oxytocin
ACTH
FSH-RH
LH-RH
ACTH-RH
TRH
PTH
Calcitonin
Insulin
Glucagon
Somatostatin
LH
TSH
Corticosteroids
Testosterone
Oestrogen
Progesterone
Adrenaline
Noradrenaline
Thyroxine
Tri-iodothyronine
Vitamin D
Note that ACTH (adrenocorticotropic hormone) is sometimes still referred to as corticotropin, and ACTH-RH (adrenocorticotropic hormone releasing hormone) is also frequently called CRH (corticotropin releasing hormone) or CRF (corticotropin releasing factor). We have chosen to use the terms ACTH and ACTH-RH in this text.
Polypeptides
Examples – vasopressin, oxytocin, prolactin, insulin, glucagon. These are usually produced as a prohormone which undergoes conversion to its active form. These hormones are stored in granules and secreted by exocytosis into the bloodstream.
Glycoproteins
Examples – TSH, FSH, LH. These are polypeptide hormones linked to carbohydrate residues. Polypeptides and glycoproteins are hydrophilic and unbound in the bloodstream.
Steroids
Examples – corticosteroids, aldosterone, sex hormones. Steroids are synthesised in the cell mitochondria, from cholesterol. These hormones are not stored in secretory granules, but are produced by the cell as and when required. Steroid hormones are lipophilic and highly bound to proteins in the bloodstream en route to the target organ.
Amines
Examples – thyroxine, adrenaline. All the amine hormones are synthesised from the amino acid tyrosine. They are stored in follicles (thyroxine) or granules (catecholamines). Some (e.g. thyroid hormones) may be converted to a more active form nearer to the site of action.
Cellular action of hormones
Hormones exert their cellular effects by initially binding to receptors, which then produce secondary effects to change cellular function.
Hormone receptor-mediated secondary effects include:
Changes in membrane permeability
Release of second messengers
Changes in intracellular protein synthesis
Hormone receptors
Hormone receptors may be on the cell membrane (or transmembranous) or inside the cell. They increase and decrease in number depending on external stimuli. When there is an excess of hormone the number of receptors decreases (down-regulation); conversely, when there is a deficit of hormone the number of receptors increases (up-regulation). Sometimes down-regulation occurs when the receptors change their chemical structure and become less responsive. The hydrophilic hormones have receptors in the cell membrane, and the lipophilic hormones (e.g. thyroid and steroid hormones) have receptors within the cell, either cytoplasmic or nuclear.
Some hormones require the presence of small amounts of other hormones in order for their receptors to be fully active. This is known as permissiveness. Small amounts of glucocorticoids, for example, are necessary for catecholamines to produce their lipolytic effects.
Mechanisms of hormonal action
Direct effects on cell membranes
The hormone alters the permeability of the membrane to certain ions, e.g. K+ and Ca2+, via G proteins. This effect is very rapid.
Effects via second messengers
Hormones bind with receptors in the cell membrane and cause intracellular effects via second messengers. The second messenger cyclic adenosine monophosphate (cAMP) phosphorylates proteins within the cell. Hormones may increase or decrease levels of cAMP. When a hormone reacts with the receptor within the cell, guanylyl nucleotide regulatory proteins are activated within the cell membrane. These may be stimulatory (Gs) or inhibitory (Gi) to the production of cAMP.
Other hormones activate different second messengers, such as inositol triphosphate (IP3 ) and diacylglycerol (DAG). The hormone receptor complex activates an enzyme which breaks down a membrane phospholipid, phosphatidylinositol diphosphate (PIP2), to IP3 and DAG. IP3 releases Ca2+ from intracellullar stores, such as the endoplasmic reticulum, while DAG activates protein kinase C, which increases cell division and multiplication. This latter reaction is enhanced by the released Ca2+.
Effects on protein synthesis
Thyroid and steroid hormones, being highly lipophilic, cross the cell membrane rapidly to bind with intracellular receptors. Once bound with their receptor they cross to the nucleus, where they bind with DNA. This increases mRNA synthesis and via ribosomes leads to an increase in protein production. This process is relatively slow.
Figure 22.2 shows the mechanism of action of common hormones.
Affecting permeability | Via G proteins increasing cAMP | Via G proteins decreasing cAMP | Via PIP2, IP3 and DAG | Via mRNA |
---|---|---|---|---|
GH Prolactin Insulin | Oxytocin Vasopressin LH FSH TSH ACTH Adrenaline (β-receptors) PTH Glucagon | Somatostatin Adrenaline (α2) | Adrenaline (α1) Vasopressin | Thyroxine Tri-iodothyronine Steroid hormones |
Control of hormone production
Various mechanisms control hormone secretion. These include levels of inorganic ions (e.g. sodium-dependent release of vasopressin), organic molecules (e.g. glucose stimulating insulin release) and direct physical and chemical stimulation (e.g. as in the case of gut hormones).
The most important regulator of hormone production is the negative feedback loop.
In the negative feedback system high levels of a substance produced by the hormone suppress the secretion of that hormone, ensuring that the level of the substance itself remains relatively constant.
Negative feedback can be direct or indirect. An illustration of direct negative feedback is the effect of circulating glucose on insulin production. An example of indirect negative feedback is the effect of circulating glucocorticoids on ACTH-RH, which in turn affects levels of ACTH.
Hypertrophy and atrophy
If the level of a specific hormone in the blood remains very low despite maximal production and release, there will be a large increase in the level of the relevant tropic hormone, and the other cells in the producing gland will enlarge and multiply in order to compensate. This is seen as a thyroid goitre in iodine deficiency. Conversely, if there are very high circulating levels of hormone produced by medication (e.g. steroids), the tropic hormones will fall to very low levels and the gland will atrophy. Tropic hormones, therefore, influence both hormone production from the cell and the size of the gland producing the hormone.
Pituitary gland
Anatomy
The pituitary gland is made up of the anterior lobe (adenohypophysis) and posterior lobe (neurohypophysis), and is connected to the hypothalamus by the pituitary stalk. It weighs less than 1 g, and is located at the base of the brain in the sella turcica.
Embryological development of the pituitary
The anterior and posterior lobes develop quite separately from each other. The anterior lobe develops from Rathke’s pouch, an outgrowth from the roof of the mouth. The posterior lobe develops as an extension from the hypothalamus, an evagination of the floor of the third ventricle. The two join together. The part of the anterior lobe which fuses with the posterior lobe is known as the intermediate lobe, and it is rudimentary in humans. The remains of Rathke’s pouch form the residual cleft (Figure 22.3).
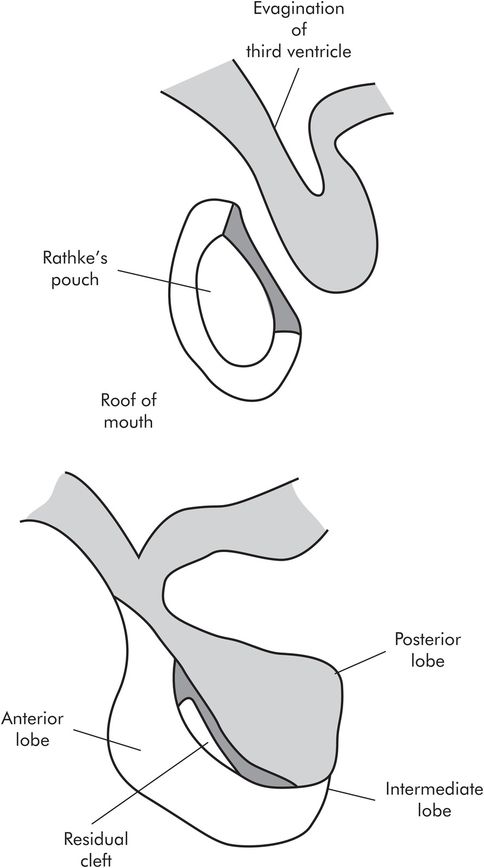
Development of the pituitary gland
Anterior lobe of pituitary
The anterior and posterior lobes have independent functions, but both are connected to the hypothalamus by the pituitary stalk. The anterior lobe is connected to the hypothalamus by the portal circulation, which transports releasing hormones into the lobe, stimulating the production of tropic hormones into the bloodstream.
The portal circulation is a network of capillaries which arises from the superior hypophyseal artery. The primary capillary plexus, located on the floor of the hypothalamus (known as the median eminence), absorbs releasing factors; the blood then passes via the portal veins in the pituitary stalk to the secondary capillary plexus, where they are released into the anterior lobe. The tropic hormones are then secreted into the plexus and into the bloodstream (Figure 22.4). The anterior lobe hormones are found in five types of secretory cell:
Somatotropes (50%) produce growth hormone (GH).
Lactotropes (10–30%) produce prolactin.
Corticotropes produce adrenocorticotropic hormone (ACTH).
Thyrotropes produce thyroid-stimulating hormone (TSH).
Gonadotropes produce follicle-stimulating hormone (FSH) and luteinising hormone (LH).
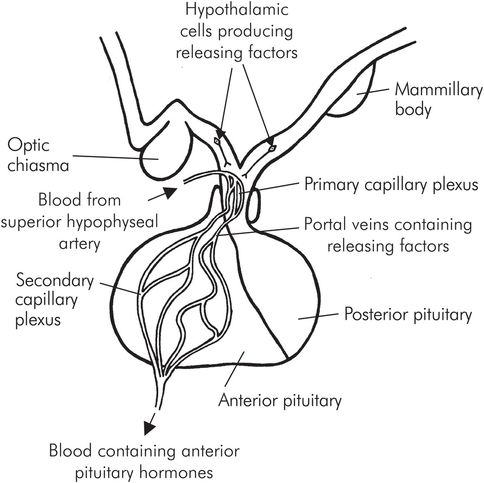
Portal system of the anterior pituitary gland
Posterior lobe of pituitary
Posterior lobe hormones are produced in neurosecretory cells in the hypothalamus, in the so-called median eminence. These form granules which pass down the axons through the pituitary stalk and are stored in the posterior lobe, later to be released into the bloodstream when stimulation occurs.
Vasopressin is produced in the supraoptic nucleus of the hypothalamus, and oxytocin from the paraventricular nucleus, within the median eminence (Figure 22.5).
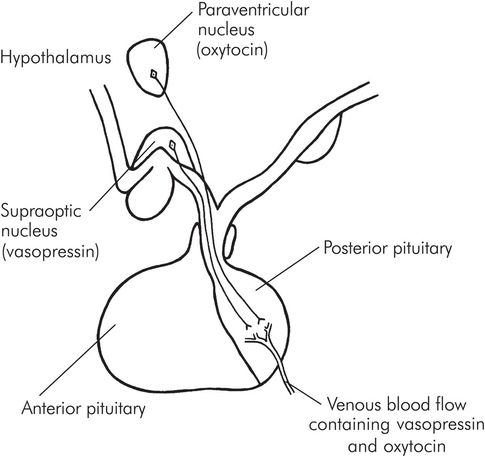
Secretion of vasopressin and oxytocin by the posterior pituitary
Pituitary hormones
Anterior pituitary hormones
Thyroid-stimulating hormone (TSH, thyrotropin)
Adrenocorticotropic hormone (ACTH, corticotropin)
Growth hormone (GH, somatotropin, STH)
Prolactin (PRL)
Follicle-stimulating hormone (FSH)
Luteinising hormone (LH)
Posterior pituitary hormones
Vasopressin (antidiuretic hormone, ADH)
Oxytocin
Functions of individual hormones
Thyroid-stimulating hormone (TSH)
Stimulates the production of thyroid hormones from the thyroid gland, and growth of the gland itself.
Adrenocorticotropic hormone (ACTH)
Stimulates the adrenal cortex to produce corticosteroid hormones (mainly cortisol) and also binds to melanotropin receptors in the melanocytes in the skin, which produce melanin and pigmentation. There is significant diurnal variation in its secretion, which is higher in the morning and lower in the evening.
Growth hormone (GH)
Stimulates the growth of tissues in the body by promoting protein synthesis, lipolysis and a rise in blood glucose. It is especially active in childhood, and causes an increase in length of the long bones until the epiphyses fuse. Not all of its effects are direct. Some of the effects on target tissues are mediated through polypeptide substances produced by the liver and other tissues, called somatomedins. These are closely related to insulin. Insulin-like growth factor 1 (IGF-1) is involved with skeletal and cartilage growth. IGF-2 also exists and is thought to be involved in fetal growth.
Lack of GH in childhood leads to dwarfism, and excess to gigantism. The patients in these cases are respectively either very small or very tall, but are in proportion. Excess GH in the adult leads to acromegaly. As this occurs after the epiphyses have fused there is little increase in height, but there is overgrowth of the skull, facial bones and hands and feet. Reduced glucose tolerance in such cases is common.
Prolactin
Prolactin is structurally similar to GH. This hormone stimulates the development of milk-producing breast tissue and milk production post partum. It also suppresses ovulation. Excess production of prolactin can cause lactation and infertility.
Luteinising hormone (LH)
Stimulates ovulation and luteinisation of ovarian follicles in females. It also stimulates testosterone secretion in males. FSH and LH together are involved in the regulation of the menstrual cycle.
Vasopressin (antidiuretic hormone, ADH)
Vasopressin causes water retention by increasing water absorption from the distal tubules and collecting ducts of the kidney.
The secretion of vasopressin is stimulated by the following changes:
A rise in osmotic pressure in the plasma (via osmoreceptors in the anterior hypothalamus)
A decrease in extracellular volume
A rise in angiotensin II levels
Pain, stress and exercise
Nausea and vomiting
Smoking
Its secretion is reduced by:
A decrease in plasma osmotic pressure
An increase in extracellular volume
Alcohol
There are several types of vasopressin receptors, V1a, V1b and V2, which are all G-protein coupled. The antidiuretic effects are mediated through V2 receptors, which open aquaporin 2 (AQP2) water channels in the collecting ducts of the kidneys. Vasopressin also has a powerful vasoconstrictor effect in vitro on vascular smooth muscle, although this is small in vivo. This is mediated through receptors found in the area postrema in the brain, and the mechanism is very sensitive to haemorrhage. Vasopressin receptors are also found in the liver, where they stimulate glycogenolysis, and in the brain. Figure 22.6 shows how these are arranged. V3 is an alternative classification for V1b.
Deficiency of vasopressin leads to diabetes insipidus, in which there is polyuria and polydypsia due to water loss. It can be primary, due to disease of the gland, or secondary, where the kidneys are unable to respond to vasopressin.
Excess of vasopressin (inappropriate secretion) leads to fluid retention with low plasma osmolarity and hyponatraemia. Vasopressin-secreting malignant lung tumours are one cause.
Type | Location | Function |
---|---|---|
V1a | Smooth muscle and heart | Vasoconstrictor effect |
V1b/V3 | CNS | Mediate release of ACTH-RH |
V2 | Collecting ducts | Permit water reabsorption |
Deficiency and excess of pituitary hormones
Pituitary deficiency can be caused by tumours and cysts which press on the pituitary gland. Acute failure may also occur in an enlarged pituitary gland following severe hypovolaemia. This may occur in women who have experienced severe bleeding and hypovolaemia during childbirth leading to pituitary necrosis (Sheehan’s syndrome). The changes which develop depend on the location of damage in the gland.
Control of pituitary hormones
The hypothalamus is intimately connected to the pituitary gland, and is responsible for a number of so-called releasing hormones. These polypeptides are produced in the median eminence of the hypothalamus, and pass into the portal system to the anterior lobe, where they stimulate or inhibit production of the tropic hormones.
Hypothalamic releasing factors include:
Thyrotropin releasing hormone (TRH)
Adrenocorticotropic hormone releasing hormone (ACTH-RH)
Growth hormone releasing and inhibiting hormones (GH-RH and GH-IH) (GH-IH is somatostatin, also produced in pancreatic islet cells)
Prolactin releasing and inhibiting hormones (PRH and PIH – dopamine)
Gonadotropin releasing hormone (Gn-RH) – stimulates production of FSH and LH
Some releasing factors have an effect on other hormones. TRH stimulates prolactin production, and GRH inhibits TSH, for example.
The hypothalamus has many inputs which are concerned with vegetative regulation, and it is also connected to other parts of the central nervous system, and its output is affected by stress, diurnal rhythm and emotional factors.
The function of the anterior lobe is under negative feedback control. When the levels of hormones which are produced in the target organs increase, the production of both the tropic hormones and the releasing factors is reduced. Conversely, when the target-organ hormone levels decrease there is increased production of both the tropic hormones and the releasing factors. This keeps the hormone levels relatively constant (Figure 22.7).
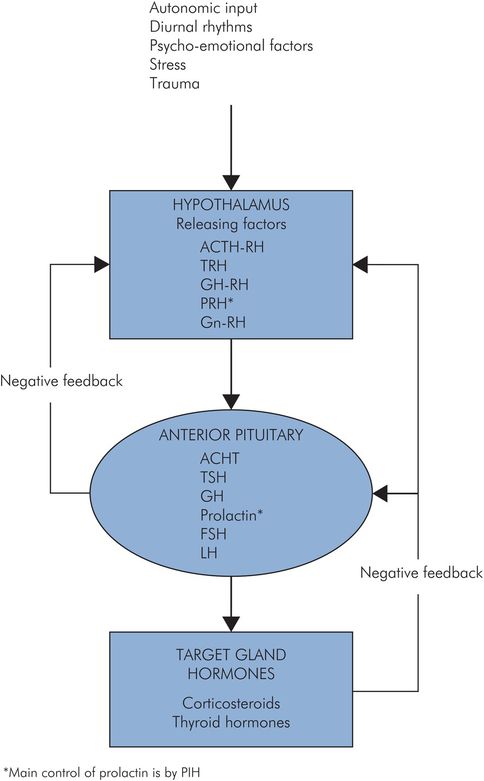
Feedback control of pituitary hormones
When steroids are given as medication over a period of time, the anterior pituitary and the hypothalamus produce minimal stimulation of endogenous cortical adrenal steroids, and the adrenal gland atrophies. This can have serious consequences if the steroids are suddenly stopped, especially if the patient is undergoing a stressful procedure such as surgery.
The pituitary hormones are summarised in Figure 22.8.
Part of pituitary | Hormone | Main stimulus and control | Action |
---|---|---|---|
Anterior lobe | TSH | +ve TRH, –ve thyroid hormones | Thyroid gland to produce thyroid hormones |
ACTH | +ve ACTH-RH, –ve corticosteroid hormones | Adrenal gland to produce corticosteroid hormones | |
GH | +ve GH-RH, –ve GH-IH | Increase growth of body tissue especially bone | |
Prolactin | +ve PRF, –ve PIH | Milk production, suppression of ovulation | |
FSH | +ve Gn-RH | Breast development, milk secretion, spermatogenesis | |
LH | +ve Gn-RH | Stimulation of ovulation, testosterone secretion | |
Posterior lobe | Vasopressin | +ve osmolarity, thirst, pain, haemorrhage | Water reabsorption from distal tubules |
Oxytocin | +ve touch receptors in breast and genitalia | Ejection of milk from breast, uterine contraction |
Thyroid gland
The thyroid gland secretes hormones which control the basal metabolic rate, enabling the body to function optimally.
The thyroid hormones affect carbohydrate, lipid and protein metabolism, also affecting growth and maturation, and body temperature.
Anatomy
The embryonic origin of the thyroid gland is from the floor of the pharynx. The thyroglossal duct marks the path of the gland from the tongue to its final site, and sometimes this persists in adults. The gland is situated in the neck at the level of the second and third tracheal rings. It comprises two lobes on either side of the trachea, joined by the thyroid isthmus. Each lobe has an upper and lower pole. Sometimes there is a third lobe, the pyramidal lobe, which arises anteriorly from the thyroid isthmus. The gland is highly vascular, its arterial supply coming from the superior and inferior thyroid arteries, and its venous drainage via the superior, middle and inferior thyroid veins. The parathyroid glands are located within the thyroid gland.
The gland itself comprises many follicles (acini). The follicles are lined by the thyroid epithelial cells, and within the follicle itself is a variable amount of colloid, which mainly contains thyroglobulin and iodine. There are also parafollicular (C or clear) cells which secrete calcitonin. The thyroid cells rest on a basal membrane, which separates them from the capillaries.
When the gland is inactive the follicles are large and contain substantial amounts of colloid. The cells are small and flattened. When the gland is active, the follicles reduce in size; there is little colloid, and the cells become enlarged and columnar or cuboid. The edge of the colloid develops a scalloped appearance due to reabsorption lacunae at the tips of the cells (Figure 22.9).