The epidemiological triangle. This type of diagram is widely used to represent the interconnectedness of the three major components involved in the emergence of infectious diseases. The “Host” is the organism that is affected by the pathogen or toxin and can develop disease. The “Agent” is the infectious microorganism (pathogen) or toxin. The “Environment” refers to the circumstances that influence the interaction between the Host and the Agent. Examples of influencing factors are given for each component.
Infectious Diseases Biology Terminology
Many pathogenic microbes have been associated with human disease for hundreds or thousands of years. Examples of infectious diseases with long human histories include smallpox, plague, cholera, malaria, tuberculosis, and syphilis. These diseases, and others, resulted in millions of deaths over the centuries and were the focus of targeted efforts of varying degrees around the world to reduce the burden of infectious diseases on human populations. Improvements to public health systems, such as sanitation, drinking water treatment, and education, reduced human contact with pathogens. Scientific advances, such as antibiotics and vaccines to treat and prevent infectious diseases, revolutionized the medical arsenal against microbes. As a result, by the middle of the twentieth century, the incidence of many infectious diseases plummeted, particularly in the developed world. It was widely thought that science had conquered the threat that infectious diseases posed to human health.
What is appreciated now is that microbes are constantly interacting with their environment and evolving. As they do, circumstances may allow for the emergence of new infectious agents/diseases, or the reemergence of previously controlled contagions. These emergences fall into many categories:4
Microorganisms that have not been known previously and that cause new diseases (e.g., severe acute respiratory syndrome coronavirus [SARS-CoV] and Middle East respiratory syndrome coronavirus [MERS-CoV]; human immunodeficiency virus [HIV] that causes acquired immunodeficiency syndrome [AIDS]);
Agents that have been known previously and that cause new diseases (hantavirus in the United States in 1993 that caused respiratory distress instead of kidney disease);
Microbes that have been known previously to cause disease, but the incidence of disease is noticeably increasing in a region (e.g., whooping cough caused by Bordetella pertussis in the United States; diphtheria caused by Corynebacterium diphtheriae in Russia);
New, and often more virulent, strains of a known pathogen that cause disease (e.g., Vibrio cholerae O139 and epidemic diarrheal disease; highly virulent Clostridium difficile NAP1/027 and increased incidence of C. difficile-associated disease in North America and Europe). Increased virulence often occurs when a pathogen acquires a genetic element that allows for the production of a new virulence factor such as a toxin (e.g., Staphylococcus aureus that produces TSST-1 and causes toxic shock syndrome);
Microbial pathogens that cause disease in a new geographical location (e.g., West Nile virus encephalitis in North America; reintroduction of epidemic cholera in Haiti; Chikungunya virus in the Caribbean; Ebola virus in West Africa);
Microbes of animal origin that infect humans (zoonoses). This includes animal-associated microorganisms to which humans are newly exposed (e.g., hantavirus pulmonary syndrome due to Sin Nombre virus from the rodent population in the United States), or animal-associated microbes that are newly able to infect humans (e.g., influenza virus from birds or swine);
Microbial pathogens that have acquired the ability to resist the effects of antimicrobial agents (e.g., multidrug-resistant tuberculosis [MDR-TB]; methicillin-resistant and vancomycin-resistant S. aureus; amantadine-resistant influenza A virus; carbapenem-resistant Enterobacteriaceae [CREs]).
The occurrence of emerging infectious diseases (EIDs) or reemerging infectious diseases in human history is not new. The great plague and influenza pandemics are well-known historical examples. The last few decades have witnessed a recrudescence of EIDs. Furthermore, as global surveillance of diseases has developed, the awareness that new EIDs are occurring has increased. Although exact numbers of EIDs are debatable due to differences in criteria used, Taylor and colleagues suggest that 175 of the 1,400-plus known human pathogens are EIDs (approximately 12%).3
Factors that Drive the Emergence or Reemergence of Infectious Diseases
Adapted from: IOM. Microbial Threats to Health: Emergence, Detection and Response. Washington, DC, National Academies Press, 2003.
Figure 8.2 shows recent EIDs and reemerging infectious diseases in both the developed and developing world.

Emerging and reemerging infectious diseases/agents, 1990–2013. The diseases/agents in the black boxes with the white dots represent select emergences that have occurred since the first edition of this textbook was published in 2010. E. coli, Escherichia coli; vCJD, variant Creutzfeldt–Jakob disease; HIV, human immunodeficiency virus; SARS, severe acute respiratory syndrome; MERS, Middle East respiratory syndrome; NDM-1, New Delhi metallo-beta-lactamase-1.
The question then is: Why are EIDs occurring so frequently despite the optimism of past generations? In 2003, the Institute of Medicine (IOM) published Microbial Threats to Health: Emergence, Detection and Response,5 which outlined thirteen factors that contribute to the emergence or reemergence of new pathogens.
Although now more than a decade old, the IOM report remains a seminal work in framing the understanding of why infectious diseases emerge. The factors reflect a very different world from previous decades. “Globalization,” often characterized by changes in global movement, economic development, and environmental and agricultural practices, has unwittingly exposed the world’s populations to microbial threats. Not all of the categories are necessary for every emerging pathogen; however, neither are they mutually exclusive. The emergence or reemergence of a pathogen is usually a function of many factors. An understanding of all the factors is necessary to prevent or quickly detect future EIDs and to determine how to effectively mitigate an EID disaster.
Table 8.3 uses pandemic influenza, dengue hemorrhagic fever, MDR-TB, HIV/AIDS, and cholera to demonstrate how these factors interplay in the emergence or reemergence of diseases.
Examples of How Multiple Factors Influence the Emergence or Reemergence of Infectious Diseases
Infectious Disease (Agent) | Pandemic Influenza* (highly pathogenic avian influenza [HPAI] virus) | Dengue Hemorrhagic Fever (dengue virus; transmitted to humans by mosquito vector) | Multidrug-resistant Tuberculosis (Mycobacterium tuberculosis) | AIDS (HIV) | Epidemic cholera in Haiti (Vibrio cholerae) |
---|---|---|---|---|---|
Emergence Factor | |||||
Microbial Adaptation | Reassortment of, or mutations in, influenza virus genes that allow for human–human transmission of HPAI virus | Adaptation of viral strains to urban mosquitoes facilitated emergence | Improper use of antibiotics allowed M. tuberculosis to develop resistances | Mutation of simian immunodeficiency virus to infect humans; emergence of drug-resistant HIV; high mutation rate complicates vaccine development | Mutations in toxin genes have resulted in an altered El Tor strain of V. cholerae with increased virulence |
Human Susceptibility | Extensive viral adaptations means no inherent immunity in humans; no vaccine-enhanced immunity in the initial months of the pandemic | No cross-immunity to the four different viral strains; heterologous infection increases chance of severe disease | Increased tuberculosis in HIV-endemic areas | Lack of host immunity when virus emerged; no vaccine-enhanced immunity | Immunologically naive population on Hispaniola (Haiti and Dominican Republic) where cholera had not been seen for a century |
Climate and Weather | Cold weather in some countries during flu season encourages social clustering and, consequently, viral transmission | Rainy seasons increase mosquito population | |||
Changing Ecosystems | Changing marshland habitats and waterfowl distribution | Repopulation of New World by mosquito species after mid-twentieth century mosquito eradication programs ended | |||
Human Demographics and Behavior | Increased worldwide poultry production to feed increased human population; cohabitation with potential zoonotic sources | Disease centers in overpopulated urban areas with poor housing and utility management that promote mosquito breeding grounds | Failure to adhere to medication regimens; people in remote areas hard to treat consistently; immigration of infected persons | Unprotected sexual activity; illicit intravenous drug use; prostitution | Disaster relief being provided in response to the 2010 earthquake |
Economic Development and Land Use | Live markets put humans and infected birds in close contact | Dam building promotes mosquito breeding grounds | |||
Technology and Industry | Crowded poultry feedlots favor viral transmission between birds | Possible disease transmission through blood products | Disease transmission through blood products | ||
International Travel and Commerce | Global travel can rapidly spread disease; illegal exotic bird trade can transfer infectious birds | Travelers can spread strains between endemic areas; outbreaks in nonendemic areas with appropriate mosquito species (e.g., southern United States) | Dissemination of M. tuberculosis on airplanes via recirculation of air | Global travel spreads disease | Travel of international relief workers to Haiti to aid in the earthquake disaster response; relief workers from Asia, where cholera is endemic/epidemic, may have been incubating cholera at the time of deployment |
Breakdown of Public Health Infrastructure | Prolonged nature of the pandemic strains resources | Lack of effective mosquito control; poor water and sewage systems in developing areas | Inability to monitor tuberculosis population; high treatment interruption rates in developing countries; HIV epidemic areas overwhelmed | Lack of education and intervention programs, overwhelmed workforce in developing countries | Disruption of already-poor sanitation, water treatment, and healthcare infrastructures by the earthquake disaster |
Poverty and Social Inequality | Rapid spread of the virus in the developing world | Developing countries that lack vector control programs risk high incidence | Expense of directly observed therapy inhibits consistent use in poorer nations | Expense of antiretroviral therapy; stigmatization of men who have sex with men, especially in early days of the emergence; marginalized women’s rights in some societies | Occurrence in a developing nation with few resources for public health infrastructure and response; waning aid and funding |
War and Famine | Increased global travel during World War I facilitated propagation of the 1918 influenza pandemic | Tuberculosis spreads quickly through refugee camps (e.g., in Somalia) | Treatment programs are difficult to administer in areas of conflict | Malnutrition in the population resulting in increased disease severity and poor outcomes | |
Lack of Will | Pharmaceutical industry and vaccine/therapeutic development | Poor surveillance in endemic countries | Inadequate infection control policies or practices | Initial low research and intervention priorities for an agent primarily spreading in men who have sex with men; refusal of officials in some developing countries to acknowledge HIV in the population | Low priority for vaccine development; initial reluctance of international relief force to acknowledge that response efforts may have contributed to the reemergence, leading to mistrust of the nation |
Intent to Harm | Theoretical potential of genetically reconstructed 1918 pandemic influenza virus to be used in a terrorist attack |
* At the time of this writing, pandemic HPAI has not reemerged. Based on knowledge from prior influenza pandemics and extensive studies on influenza virus epidemiology and genetics, experts have uncharacteristically broad insight into factors that affect how these zoonotic pathogens emerge.
Another example of the convergence of factors resulting in disease emergence and transmission is the Ebola virus disease (EVD) outbreak in West Africa,6 the first such outbreak in that region and a situation still evolving at the time of this writing in August 2014. With 3,069 cases and 1,552 deaths reported as of August 26, 2014 in Guinea, Liberia, Sierra Leone, and Nigeria, it is the largest EVD outbreak to date. The outbreak started in Guinea; phylogenetic analysis suggests evolution of the virus in the area as opposed to importation of the outbreak strain from other countries.7 The initial EVD cases and transmission were unrecognized, likely a result of local clinicians’ unfamiliarity with the disease and its symptoms, and also due to inadequate healthcare availability and resources. Human behaviors in the area may have also played a role in the emergence and persistence of the disease, including: funeral practices that involve touching the deceased; food habits such as consumption of bats, a likely vector; mistrust of government officials, aid organizations, and healthcare workers resulting in delayed or avoided care; and fear, resulting in actions such as airplane flight limitations that affect resources and response capabilities. Indication that residents attacked and looted an EVD clinic in a poor neighborhood of Monrovia, Liberia suggests instability in the area is resulting in unconventional risks for further transmission of this disease. Likely the most critical factors related to the scope of this EVD outbreak compared to previous outbreaks are modernization and urbanization. Cases have occurred in larger cities with more mobile populations, and travel by land and air has transmitted disease between countries, hindering the comprehensive contact tracing necessary to prevent continued transmission.
Many of the thirteen factors outlined by IOM drive disease emergence by influencing the interaction of humans with animal reservoirs of potential pathogens. In fact, approximately 75% of recently emerged pathogens are zoonotic. The abundance, location, and behaviors of putative animal reservoirs, and human influences on them, are important factors in disease emergence. Microbes often live in harmony with animal hosts and the pathogenic infection of humans is inadvertent.
Infectious Diseases and Disaster Medicine
History has shown that infectious disease outbreaks, epidemics, and pandemics have the potential to afflict large numbers of people. Estimates for the next severe influenza pandemic suggest millions of cases in the United States alone with hundreds of thousands of flu-related fatalities. The 2001 deliberate release of anthrax spores in the United States through the postal system and the 2003 SARS pandemic are reminders that the scope of the disaster is not just a function of actual case numbers, but of the ability to manage the outbreak and to the public reaction during the event. Both situations taxed the available resources of some of the most sophisticated public health systems in the world despite relatively low numbers of cases.6,7
Disasters are commonly considered to be acute, often regional, events. Even in the realm of infectious diseases, the anthrax letters incident in the United States is often cited as an example of the type of response required for an infectious disease disaster. More likely, however, biological situations (of either intentional or unintentional origin) that strain response efforts will unfold in a more gradual manner. Furthermore, if disasters are defined as situations that require external resource assistance, then the global AIDS pandemic (now decades long) can be considered a disaster. Disasters due to EIDs are of particular concern given the paucity of information on the biology of the agent, the course of disease, and mechanisms of treatment. Even a local outbreak of a known infectious agent can strain a response effort.
Management of infectious disease disasters shares many general aspects of the management of other disasters. The basic principles of leadership and collaboration, resource management, surge capacity, triage, and public relations are all important; however, the specifics of response activities can have special considerations when an infectious agent is the cause of the disaster.
Table 8.4 provides a description of unique features of infectious disease disasters that are not usually encountered in many other disaster response efforts.
Challenges of Infectious Disease Disasters that May Differentiate Them from Other Types of Disasters
Category* | Challenge |
---|---|
Infectious Agent | Novel agent or one not previously associated with disease |
No known treatment or cure | |
Unknown reservoir | |
May not initially be recognized as the causative agent of the disaster | |
Disease | Not characterized previously |
Medical community lacks experience identifying and treating | |
Symptoms are similar to other infectious diseases | |
People who are concerned about exposure but not truly exposed | |
Transmission | Contagious agent – large numbers infected over time |
Global response may be necessary to contain agent | |
Multiple cities affected | |
Disaster could last weeks, months, years or decades | |
How to decide when the disaster is over | |
Personnel | Exposure of response personnel to agent |
Healthcare workers’ absenteeism due to concern of contracting agent | |
Resources | Isolation of cases in the healthcare facility |
Decontamination of hospital equipment | |
Capacity of laboratory to process samples | |
Distribution of limited supplies (drugs, equipment) | |
May be other infectious disease outbreaks concurrently | |
The Public | Quarantine and Isolation |
Screening for symptoms (at hospitals, airports) | |
Controlling movement (closed borders) | |
Closing services (schools, churches, public transportation) | |
Psychological fears | |
Media relations | |
Ethics and Law | Mass vaccinations |
Quarantine/restriction of movement | |
Allocation of resources | |
Demands on healthcare workers, first responders | |
Terrorism | Balancing epidemiological and criminal investigations |
* The first three categories (agent, disease, transmission) are unique to infectious disease disasters. The remaining categories (personnel, resources, the public, ethics and law, and terrorism) may apply to other types of disasters, but the challenges listed are unique or particularly applicable to infectious disease disasters.
The Infectious Agent
Infectious disease disasters, unlike physical and chemical incidents, are caused by biological entities that are diverse and under constant selective pressures to change. It may be clear that an outbreak has occurred due to the contagiousness and nature of the illness that characterize cases presenting to healthcare facilities; however, the identity of the agent that is sickening patients may be elusive, and any effort to mitigate the disease and spread of the agent will be compromised. Cases of severe atypical pneumonia perplexed physicians in Guangdong province, China in 2002. Chinese officials maintained the causative agent to be a bacterium called Chlamydia.8 It was not until months later, after global spread occurred requiring a then-unprecedented international response effort, that a new coronavirus was publicly identified as the cause of a heretofore-uncharacterized disease, SARS.
For a number of infectious agents, previously known or unknown, there is no specific treatment or cure. Medical management is limited to supportive care, which may require long hospital stays. Depending on the number of afflicted persons, this could affect resource availability (discussed later). The unknown nature of some pathogens also limits detection and diagnostic capabilities.
Infectious agents are often zoonoses. Human infection from the animal reservoir occurs when environmental and behavioral factors coincide to allow for transmission of the agent. In the case of EIDs, the identity of the animal reservoir may be unknown. Successful mitigation of disease spread is contingent on discovering the reservoir. The 1993 emergence of hantavirus pulmonary disease in different locations in the United States occurred due to increased contact between rodent and human populations; disease eradication followed reduction of human contact with rodent excreta.
The Disease
In some situations, the medical literature may not have previously described the disease (e.g., the various viral hemorrhagic fevers that have emerged over the years), or a particular disease was not previously associated with a type of infectious agent (e.g., acute respiratory disease and hantaviruses). In either case, understanding the mechanism of disease is important to provide effective care and prevent future cases. Incomplete or incorrect disease classification hampers an effective response effort. Alternatively, a disease may be classically associated with an infectious agent; however, outbreaks are rare (e.g., SARS) or historical (e.g., smallpox) and the medical community lacks experience in identifying and treating the disease. This scenario can also affect the timeliness with which a disaster is controlled.
In many instances, an EID has similar symptoms to other diseases that are endemic to a region. SARS patients had general symptoms of fever, headache, and malaise that typically progressed to pneumonia. Healthcare workers had the daunting task of differentiating patients with respiratory ailments to properly isolate and treat the SARS cases.9 Likewise, a 1995 Neisseria meningitidis outbreak in Minnesota occurred during flu season, overwhelming a hospital emergency department and complicating triage.10 Additionally, some cases of EVD in West Africa in 2013 and 2014 may initially have been diagnosed as malaria6 – a disease that requires different clinical and infection control practices to prevent illness and transmission.
Particularly during epidemics with common symptoms such as headache and fever, healthcare facilities may be inundated with the so-called worried well. Although psychology experts have advocated for abandoning this phrase and replacing it with more appropriate terminology such as “medically unexplained symptoms,” it is still often used to refer to persons who think they may have symptoms although they do not actually have the disease, or to well persons who present to healthcare facilities in the hopes of receiving prophylaxis “just in case.” These situations are understandable given the fear of contracting the infectious disease and the desire to protect oneself and one’s family. Communication with the public is an important component of the response. It provides information on the disease and actions to take if people think they have been exposed. Crowd control, screening, and triage may be necessary actions to separate infected and uninfected persons.
Transmission of the Infectious Agent
An infectious disease may be contagious. This occurs when the reproductive rate (R0) – the average number of secondary cases to which an infected person spreads the disease when no control measures are used – is greater than 1. Some agents, such as Bacillus anthracis (the causative agent of anthrax) are not contagious (R0 < 1) and containment of the disaster is dependent on prevention of human contact with B. anthracis spores in the environment. Many other infectious agents are contagious (R0 > 1). Pandemic influenza R0 estimations vary, but most are approximately 2–3.11 This means that one person with influenza will likely infect two other people. Interestingly, in the case of SARS-CoV, the R0 was usually approximately 2–4, yet some people appeared to be super spreaders, passing the virus to at least ten people.12 This variance in R0 among different hosts complicates predictions of the magnitude of the epidemic.
There are many implications of a communicable disease agent for disaster relief. Large numbers of afflicted persons could result from a single “emergence” of an agent or from one bioterrorist attack because more and more people are exposed to the agent. Due to travel of infected persons (e.g., SARS in 2003, MERS in 2013), or environmental factors that influence animal ecology (e.g., hantavirus pulmonary syndrome in 1995), the infectious disease may affect many cities, straining the ability of national and regional agencies to assist in local response efforts. Furthermore, as multiple neighboring public health jurisdictions are affected, communication and collaboration becomes important. If the infectious agent crosses international borders, a global effort may be required to end the spread of disease. This could include travel restrictions, surveillance, and the sharing of resources (e.g., vaccines and antibiotics) and technology (e.g., diagnostics).
The communicability of an infectious agent can also affect the duration of the disaster. Rather than resulting in an acute incident, an infectious disease disaster could last weeks, months, or even years as waves of people are affected in a region or across the globe. Pandemic influenza is predicted to last 18–24 months. The AIDS disaster has lasted for decades. Sustaining disaster relief for years will be challenging – resource utilization, a fatigued healthcare workforce, even changing political administrations, can all affect response and recovery efforts. As mentioned previously, other infectious disease outbreaks will surely occur, requiring an even greater effort from an already overwhelmed system.
Transmissibility of infectious agents in the context of disaster medicine and infectious disease emergence is poignantly exemplified by the cholera epidemic in Haiti that followed the devastating January 2010 earthquake. The country, already burdened with suboptimal public health, medical, and sanitation infrastructures, was critically in need of assistance to respond to victims of the earthquake. The world reacted, and thousands of aid workers descended on Haiti in the days, weeks, and months after. In October 2010, a new issue began to emerge – increasing cases of severe, acute gastrointestinal illness in areas along the Artibonite River that spread to all parts of the country. Surprisingly, the disease was cholera, which had not been seen in Haiti in over 100 years. It was possible that a local strain of the bacteria had reemerged after the earthquake. However, extensive analysis revealed an unexpected source – molecular characterization of the strain and epidemiological evidence strongly indicate that the epidemic strain of Vibrio cholerae was introduced to the region by a vessel of United Nations relief workers from Asia. It appears that sewage disposal practices on the ship introduced a particularly virulent South Asian strain of V. cholerae to the Artibonite River and eventually exposed millions of immunologically naive people to the agent, largely due to insufficient sanitation and healthcare systems. By the fall of 2013, hundreds of thousands of people had become ill and over 8,000 people had died in Haiti,13 and the bacteria had spread to neighboring Dominican Republic and Cuba. This is the first widely documented case of the international disaster response resulting in a second large-scale disaster of an infectious nature. This occurrence has not only led to mass casualties, but also to the reemergence of an infectious disease in a region.14
Implementation of the incident command system for disaster relief of an acute event such as a fire is relatively straightforward, with a clear start and end point. The beginning and end of an infectious disease disaster can be much less clear. Often, a period of days occurs when no new cases are diagnosed, the outbreak is determined to be over, and public health response activities return to normal; then, the community experiences a second wave of cases and public health and healthcare entities must work quickly to reinstate outbreak procedures. This concept is illustrated by the 2003 SARS epidemics in Ontario, Canada and in Taiwan, with a focus on infectious disease transmission in the healthcare setting. The 2003 SARS epidemic curve for Ontario, Canada demonstrates two phases of increased disease incidence (Figure 8.3).

Reported SARS cases in Ontario, Canada in 2003 demonstrating the two phases of the epidemic. A) Number of reported cases of SARS by classification and date of illness onset – Ontario, Canada, February 23–June 7, 2003. B) Number of reported cases of SARS in the second phase of the epidemic by source of infection and date of illness onset – Toronto, Canada, April 15–June 9, 2003.
Provincial public health officials had assumed that the outbreak in Ontario was contained at the end of April 2003 because no new cases of SARS were diagnosed after April 20. World Health Organization (WHO) officials concurred; the travel advisory to Toronto was lifted on April 30 and Toronto was removed from the WHO list of locations with disseminated SARS on May 14, 2003. Ontario health officials relaxed the strict hospital infection control directives for SARS. Days later, the second phase of the epidemic in Ontario began. Apparently, patient-to-patient and patient-to-visitor spread of the virus was still occurring unnoticed at one hospital. When SARS control measures were lifted, viral exposure of hospital workers led to a resurgence of cases.
Once again, infection control directives were issued, the hospital ceased admitting new patients, and hospital workers faced restrictions and quarantine.15 Taiwan also had transmission of SARS among healthcare workers.16 In contrast to the Toronto experience, some patients and staff were quarantined in the affected healthcare facility, infection control practices were enhanced at all facilities, and extensive community screening, outreach, and infection control practices were instituted. Although the total case count was higher in Taiwan, the outbreak curve was not bimodal. The experiences of these two cities stress a number of points: 1) Surveillance is critical to limiting the spread of an infectious agent in the healthcare setting. All patients and healthcare workers should be monitored for development of symptoms. 2) Decision-makers must be wary of relaxing strict infection control measures too soon. Although officials in Ontario and at WHO waited at least 20 days (two incubation periods) before lifting the SARS directives, this action was complicated by the difficulty in differentiating SARS patients from patients with other respiratory ailments. 3) The psychological toll on affected citizens, and especially healthcare workers who may have witnessed their colleagues become sick and die, was immense in both cities and must be factored into the situational awareness for the event for determination of response actions. The very nature of infectious agents is often unpredictable, especially when the agent is newly emerging. This reality needs to be balanced with the desire to return an overwhelmed staff and system to normal operations.
Food-borne transmission of infectious agent adds other facets to the epidemiological investigation. Identification of the contaminated product(s) can involve: obtaining food histories from cases and controls, sometimes weeks after the initial cases surface; extensive laboratory analysis of food and environmental samples; consideration of food distribution networks and trace-backs to food sources; implications on the food industry and consumer perceptions; differences in local, regional, and national food outbreak surveillance protocols; and ramifications of/to the economy and international trade. The 2008 Salmonella serotype Saint Paul outbreak, associated with over 1,400 cases in the United States and Canada – initially attributed to tomatoes and then to Mexican hot peppers – has been the subject of numerous hearings and analyses to elucidate shortcomings in food safety and outbreak response in North America.17 Likewise, the 2011 outbreak of E. coli O104:H4 in Germany and other parts of Europe, which resulted in about 4,000 illnesses and 53 deaths, was misattributed to Spanish cucumbers before Egyptian sprouts were identified as the likely transmission vehicle. International response included the banning of Spanish and/or European Union produce by some countries (e.g., Russia), a UN epidemiological investigation of Egyptian fenugreek seed suppliers, and the Egyptian government refuting claims that seeds from their growers were the source of the outbreak.18
Response Personnel
The communicability of infectious diseases poses a unique threat to first responders, hospital emergency departments, and primary care providers. Although a radiological attack can result in exposure of healthcare workers, the mechanism and nature of the injuries is well-defined and the threat, once identified, can be relatively easily contained and avoided. In contrast, containing an infectious agent in the healthcare setting can be far more insidious – some people may be asymptomatic carriers of the agent, surfaces may be contaminated, and appropriate personal protective equipment (PPE) may not be in use. The infectious nature itself of a newly emerging pathogen, including whether it is contagious prior to symptom onset, may not even be recognized. All of these factors can result in exposure of healthcare workers to the agent. In the 1957 influenza pandemic, healthcare workers constituted a large proportion of the infected. The emergence of Ebola-Zaire virus in 1976 devastated the region, including the clinic run by Belgian missionary Sisters. Almost 20 years later, 30% of physicians and 10% of nurses were infected with Ebola-Zaire during an outbreak in the Democratic Republic of the Congo (formerly known as Zaire).19 SARS in Toronto primarily spread in the healthcare setting (72% of cases were healthcare related), and 44% of cases were healthcare workers.20 MERS transmission in 2013 was also documented in the healthcare setting.21,22
It may be necessary to restrict the movement of individuals in a community to prevent spread of the infectious agent. This is particularly true in the healthcare setting where infectious people congregate and where immunocompromised patients can be exposed. During the SARS pandemic, many healthcare workers were directed to function under work quarantine. These workers were instructed to go to work or stay home, with minimal contact outside these areas. Many healthcare workers are stationed at different facilities or have more than one healthcare-related job. The movement of workers between facilities could expose many more patients to the infectious agent, yet prohibiting this movement would leave facilities understaffed.
Health professionals are a dedicated group of individuals who adhere to a code of ethics to provide care for the ill and injured (often referred to as “duty to care”); however, the management of infectious disease outbreaks is stressful. The long hours often due to understaffing, high volume of patients, duration of the outbreak, and publicity can have adverse psychological impacts on responders and primary care providers. If the infectious agent is emerging and unknown, highly communicable and/or highly lethal, it is possible that healthcare workers will be unwilling or unable to perform their duties. Various studies have been conducted to assess healthcare worker willingness to provide care during infectious disease disasters (notably SARS23 and influenza pandemics24) and the contributing factors for refusing the duty to care. An analysis of published, peer-reviewed articles found that personal obligations and protection of self and loved ones from disease via availability of antiviral medication and/or vaccine were important determinants in willingness to report to work.25
The personnel “on call” during an infectious disease disaster are not just the direct patient care staff. Public health staff (nurses, epidemiologists, sanitarians, and laboratory technologists) will be involved from the beginning to determine the extent of the disaster and how to stop the spread of the infectious agent, and to identify the infectious agent source. These efforts necessitate long work hours for days and often weeks. A second unrelated infectious disease outbreak or disaster could occur during or shortly after the first disaster, requiring the same personnel to act without respite. This protracted demand on the workforce may require recruitment of additional personnel not specifically trained for a particular task to maintain the increased level of service (surge capacity). For example, in the 1995 N. meningitidis outbreak in Minnesota, extra people were needed to dispense antibiotics, a job that legally could only be performed by a registered pharmacist until the licensing board provided emergency authorization for others to do so. Understanding surge capacity needs is critical to timely, consistent, and effective remediation of the event.
Resources
The availability of resources in public health is a concern even in the absence of a disaster situation. The 2004 shortage of seasonal influenza vaccine in the United States resulted in long lines, distribution issues, and public attention – the shortage itself became a disaster of sorts. This scenario of limited vaccine availability was heightened in the 2009 H1N1 influenza pandemic,26 even though disease severity was not high. During an outbreak or epidemic, mobilization of potentially large volumes of preventive and/or prophylactic medicines to the affected area(s) is necessary in a short period of time. Approximately 10,000 courses of ciprofloxacin were required to treat the people possibly exposed to anthrax spores in the United States in October 2001.27 The 1995 N. meningitidis outbreak in Minnesota resulted in the vaccination of 30,000 people, more than half the population of the town. The vaccine stock was not available locally and it took 2 days to deliver the medication to the impacted area. In the 2010 emergence of cholera in Haiti, oral cholera vaccine was not used based on a number of factors including unavailability of enough doses for the population and logistical issues with implementing a vaccination campaign in the aftermath of the earthquake.28
Healthcare workers and other response personnel at risk of exposure must use appropriate PPE to prevent exposure to the infectious agent. U.S. hospitals use national guidelines for the types of PPE required based on the mode of pathogen transmission (e.g., contact, droplet, or airborne). Details on the types of PPE required for the different modes of transmission are available at the U.S. Centers for Disease Control and Prevention (CDC) website.29 The World Health Organization also espouses use of PPE for response to infectious conditions.30 Public health experts may recommend extra precautions when the agent initially emerges and there is incomplete information on the mode(s) of transmission. For example, evidence suggested that SARS-CoV was not spread by airborne transmission (characterized by dissemination through the air on small particles); however, healthcare workers were often directed to wear airborne PPE (N95 respirators). Consideration should be given to ensuring that PPE can be used properly in an emergency situation (e.g., some respirators need to be fit-tested for optimal functioning) and to contingency plans if PPE availability is insufficient.
The healthcare workforce is a resource itself. As workers become ill, stressed, or quarantined, fewer people will be available to care for patients (in fact, the number of patients may increase as workers become patients). Some of the most qualified people to treat disease will be on the front lines at the beginning of the disaster and at increased risk of contracting disease. This may require less experienced individuals from other departments to fill the void. Many healthcare workers died from SARS in 2002 and 2003, including Dr. Carlo Urbani, the WHO infectious diseases specialist in Vietnam who is credited with discovering the outbreak and taking steps to prevent its spread.
Even with the proper use of PPE, a contagious microbe can spread in the healthcare setting. Examples include patient-to-patient or patient-to-visitor transmission. Therefore, the isolation of infectious patients to one area of the facility is recommended. This may necessitate extra equipment and supplies dedicated for use in the isolation area. Patients infectious with pathogens spread by airborne transmission (or with emerging pathogens for which airborne transmission is suspected) should be sequestered in negative pressure rooms from which air is released directly outside or filtered before recirculation throughout the facility. There are, however, limited numbers of these units and a large infectious disease disaster may require cohorting multiple patients in the same room or even the establishment of facilities committed to treating only infectious patients. During other types of large disasters, patients are often transferred to various hospitals in the region. Although this has been successfully accomplished in some infectious disease disasters (e.g., in Singapore during the SARS epidemic), any patient transfer risks further spreading of the disease and should be undertaken within the context of overall containment strategies. Furthermore, in systems that allow it, neighboring hospitals may be unwilling to accept patients from hospitals with confirmed cases due to concern of the disease spreading to their own patients and staff. If the original hospital is designated as an infectious disease facility, these other hospitals may be willing to accept nonexposed patients in transfer, thereby increasing capacity for contagious patients within the original facility.
Equipment that is used to treat multiple patients, ranging from stethoscopes to ventilators, must be properly managed between patients using disposal or decontamination processes as appropriate. This may be particularly difficult for new infectious agents for which effective decontamination protocols are not known. Furthermore, taking equipment out of circulation, even temporarily, may delay treatment of patients.
There are usually two general aspects to mitigating an infectious disease outbreak: the care of individual patients (to alleviate disease and suffering) and the population epidemiological investigation and response (to prevent further transmission). In both cases, laboratory testing of human and/or environmental samples for evidence of the pathogen is important to ensure the correct intervention strategies are directed to the right people and areas. Although an increase in the number of patient samples during an outbreak is often expected, the number of environmental samples can be quite large. At times, the magnitude of testing required is overwhelming to even the larger regional, national, and international laboratories, whose services are required for sizeable incidents and/or for the testing of certain pathogens. For example, thousands of analytical assays were performed on environmental samples in the 1993 U.S. hantavirus pulmonary disease epidemic, in the 1999 West Nile virus emergence in the United States, in the 2001 U.S. anthrax attacks, and in the 2012 E. coli O104:H4 outbreak in Germany. The response to an EID outbreak may be largely dependent on the local public health workforce, but this response may be directly reliant on the capacity of other health departments and agencies.
The Public
The 2003 SARS epidemic in Toronto provides numerous examples of unique considerations for interacting with the public during an infectious disease disaster. The etiology of SARS was initially unknown, but it was apparent that person-to-person transmission was occurring. Therefore, voluntary quarantine measures were implemented, representing the first time in 50 years that such measures were used in North America to control disease transmission in a community. Approximately 23,000 people were asked to adhere to home quarantine (remain at home, wear a mask, have limited contact with family members, and measure their temperatures twice a day) and/or work quarantine. Studies after the epidemic ended suggest that complete compliance to home quarantine requirements was low.31 Respondents to a web-based survey indicated confusion over the quarantine instructions and inability to contact public health officials for clarification. Furthermore, the quarantine period was necessarily 10 days, a relatively long time for most people to be away from work and community activities.
For this pandemic, it was not necessary to close borders (within and/or between nations) to general travel. Diseases with higher transmission rates, such as smallpox from a bioterrorist attack (estimated R0 = 10),32 may require such stringent measures. Issues to consider are enforcement, the effect on businesses, and the effect on the supply chain for disaster management. Institutions within a community where people congregate may require closure, including schools and places of worship.
Whether or not movement or quarantine measures are implemented, public concern and psychological trauma will likely be high for both contagious and noncontagious diseases. This concern will be a function of exposure risk to the agent and subsequent infection, the severity of illness, and the availability of treatment for oneself and one’s dependents. Media coverage during the disaster influences community resilience and either exacerbates or alleviates fears, depending on perceptions of the mitigation effort and truthfulness and accuracy of the messages.
Ethics and Law
There are many ethical and legal considerations in the management of an infectious disease disaster. The following issues are illustrative:33
The process of making population-based decisions for infection control during a disaster (e.g., mass vaccinations, quarantine, and movement restrictions) will raise concerns about the legality and necessity of infringements of individuals’ rights.
A scarcity of resources such as vaccines, therapeutics, or hospital equipment will require difficult decisions about who receives the resources and who does not.
In the event of a disaster caused by a highly contagious, highly virulent, uncharacterized, and/or genetically engineered agent, to what extent should first responders and other healthcare workers be expected to comply with “duty to care” orders for the public good?
Terrorism
This chapter will not elaborate on preparedness and response for infectious disease events caused by bioterrorism because their presentation and management is similar to that for other microbial threats. Criteria include most of those already described, albeit some may be particularly relevant (e.g., public fear, the number of areas affected, and laboratory capacity). The U.S. Department of Health and Human Services (HHS), specifically CDC, and the U.S. Department of Agriculture, Animal and Plant Health Inspection Service jointly administer the Federal Select Agent Program, which maintains and oversees use of a list of select agents and toxins that “have the potential to pose a severe threat to public, animal or plant health or to animal or plant products.”34 Diseases caused by many of the agents on this list, including anthrax, smallpox, and the viral hemorrhagic fevers, are not commonly encountered by the medical community in the Western hemisphere. A bioterrorist may use an agent that has been genetically engineered to be highly virulent, resistant to therapeutics, and/or to cause a novel disease. In these cases, health professionals will be at a further disadvantage to prevent disease and death.
As with every terrorist attack, a criminal investigation should ensue after a bioweapon is used. In other types of attacks, this investigation begins immediately after the actual incident has occurred (e.g., an explosion), during the aftermath and rescue efforts. When a bioweapon is used, it may be days or longer before exposed people develop symptoms. Depending on the agent used, it may be an even longer time before a crime is suspected. The site or mechanism of the actual agent release may never be known. If the agent used in the attack occurs naturally in the region, foul play may not even be suspected.
Table 8.5 lists some clues that suggest an outbreak could be due to criminal activity. Although the criminal investigation will focus on finding the perpetrators of the attack, a second investigation – an epidemiological investigation – will be progressing as well to determine the cause and spread of disease. Both public health and law enforcement investigations will require sample analysis and interviews with the public and must progress in a collaborative manner despite each entity having different goals.
Indications that an Infectious Disease Outbreak May Be Due to a Bioterrorist Attack
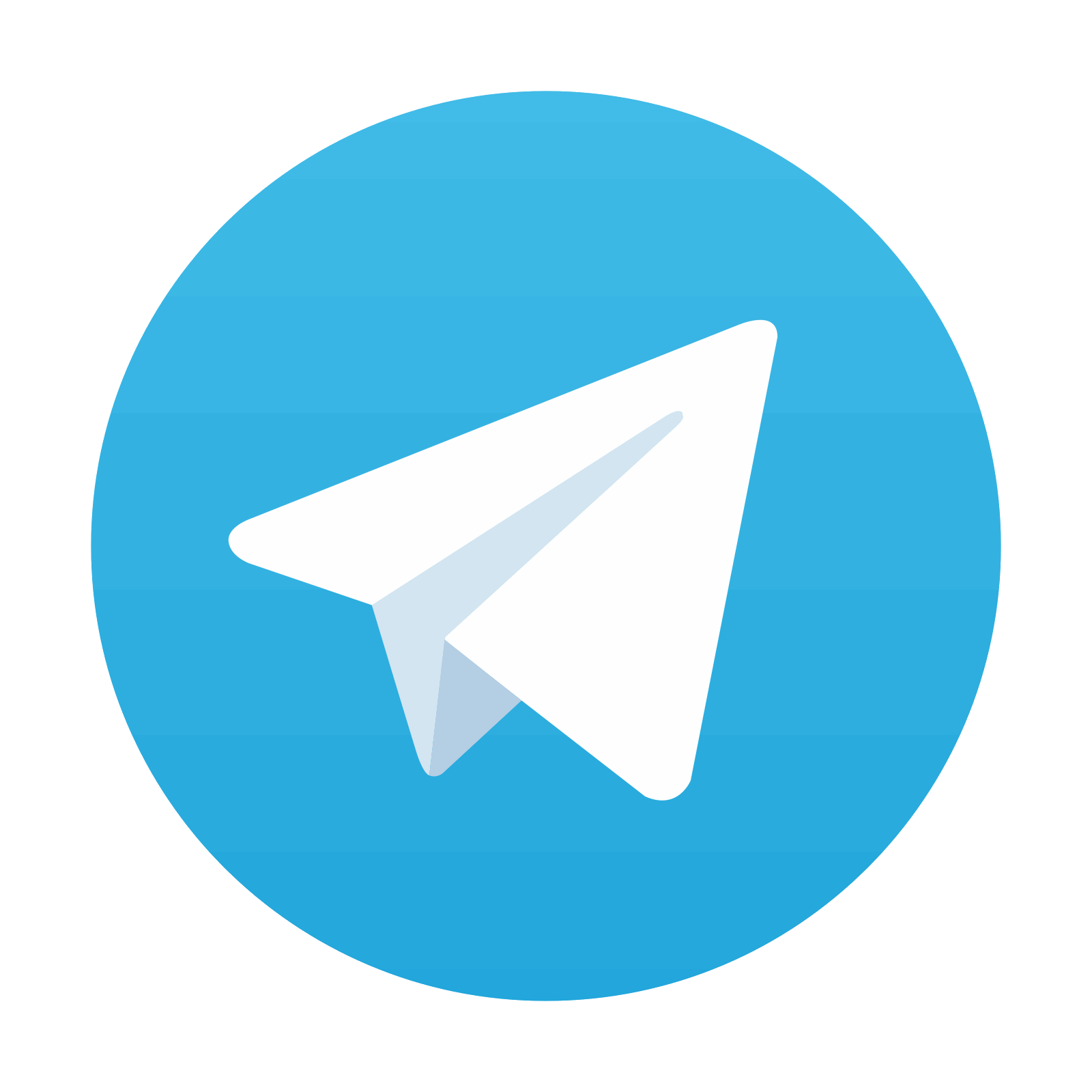
Stay updated, free articles. Join our Telegram channel

Full access? Get Clinical Tree
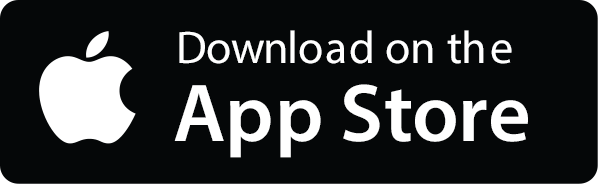
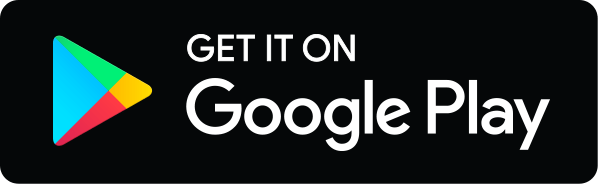