FIGURE 1 General methodology for acquisition and recording of NWR and RRF. (A) NWR responses are evoked by electrical stimulation in different locations on the extremities (in the figure, only electrical stimulation at the foot sole is shown). The reflex responses are recorded by surface EMG at any muscle biomechanically involved in the NWR response. (B) Specifically for RRF recording, stimuli are delivered at several sites in randomised sequence, and the EMG signals are averaged for every stimulation site. The reflex size is typically quantified in the 60–180 ms time interval (indicated by the vertical lines). (C) The NWR size detected at each electrodes is interpolated and extrapolated. (D) The two-dimensional interpolation map is then superimposed onto a map of the foot for depicting the NWR sensitivity in a particular muscle (Modified from Neziri AY, Curatolo M, Bergadano A, Petersen-Felix S, Dickenson A, rendt-Nielsen L, Andersen OK. New method for quantification and statistical analysis of nociceptive reflex receptive fields in humans. J Neurosci Methods 2009;178:24–30).
Reliability of the NWR and the RRF
Reliability can be defined as the amount of measurement error that is acceptable for the effective practical use of a measurement tool. Establishing the reliability of a biomarker related to pain is essential if it is to be used for detecting differences within and between healthy individual and patients, to follow-up the progression of a given disease, and to investigate the effect of pharmacological interventions, among others. As such, reliability has to be analysed prior to any other experimental hypothesis, since their validity could be questioned if such a test is not adequately consistent in whatever value it indicates from repeated (i.e. test-retest) measurements [8].
Previous studies addressed the test-retest reliability of the NWR threshold in populations of healthy volunteers [48]. The absolute reliability (expressed as coefficient of variation) for the NWR threshold was 5% within the same day, 17% after 4 days and 34% after 16 weeks, whereas the relative reliability (expressed as intraclass correlation coefficient) was 0.98 within the same day and 0.82 after 4 days (no data on relative reliability was reported for the 16 week interval) [26,48]. The test-retest reliability of the NWR threshold was also tested in a population of chronic pain patients, resulting in absolute reliability values of 17% after 7 days and 22% after 14 days, whereas relative reliability values were 0.81 and 0.71 after 7 and 14 days, respectively [13]. In general, the reliability of the NWR in healthy volunteers is comparable to that found in patients with chronic pain, and it is acceptable for experimental and clinical assessment of nociception. However, special caution is necessary when follow-up studies are planned involving long periods of time between sessions (since the reliability decreases with increasing intervals between test and retest).
THE NWR IN THE ASSESSMENT OF SPINAL NOCICEPTION
Relationship to Other Measures of Spinal Nociception
The stimulus-response function to electrical stimulations reveals a linear relationship to stimulus intensity at supra-threshold intensities and a strong correlation to the pain intensity under controlled experimental conditions [17,27,80]. Neurons located in the deep dorsal horn receiving input from both tactile and nociceptive afferents are intercalated in the reflex pathway and the excitability of these neurons is strongly modulated by robust nociceptive activity [69]. Continued nociceptive input leads to gradual depolarisation of spinal neurons and subsequently lower thresholds, leading to stronger neuronal firing for the same afferent input [72]. This kind of temporal summation at spinal level can also be assessed non-invasively using the NWR [5,55]. Stimulation frequencies ranging from 0.5 Hz to 20 Hz and trains lasting up to five seconds have been tested [7,9]. A gradual increase in reflex size is observed during the initial phase of repetitive stimulation which is then followed by a reduction in reflex size [9] most likely reflecting the balance between spinal integration and (delayed) inhibitory mechanisms triggered by the robust input. The reflex build up most likely resembles the initial phase of the wind-up phenomenon which is most pronounced in spinalised animals [30]. Temporal summation has also been tested in relation to reflex receptive fields. Summation sensitivity is most pronounced in the center of the RRF whereas the amount for temporal summation is less in the fringes of the RRF [3].
Modulation of Spinal Nociception in Humans
Under physiological conditions, descending inhibition and facilitation can adapt the responsiveness of the nociceptive system to protect the body from actual or potential injury. Early research on supraspinal control of reflex excitability mainly focused on descending inhibitory control as a potential target for analgesic mechanisms, although later on it became evident that supraspinal control can be both inhibitory and facilitatory [35,54]. Recent studies involving animals suggest that descending facilitation may have a very rapid onset, whereas descending inhibition shows a late occurrence, probably triggered by sufficient C-fiber afferent activity and temporal summation [86]. The neural basis for bidirectional control from the midline system is two populations of neurons in the rostroventral medulla (ON-cells and OFF-cells) that are differentially recruited by higher structures to enhance or inhibit pain [35]. Thus, the rostroventral medulla has been suggested as the last relay, but more rostral centers may contribute such as the anterior cingulate cortex, the reticular nuclei and the periacqueductal grey [60]. These structures can be modulated by cognitive or physiological factors and there is increasing evidence that they may contribute to central sensitization in the transition from acute to chronic pain [35,78].
Supraspinal control is strongly modulated via concurrent tonic nociceptive input. Hence, conditioning pain modulation (CPM) has been shown to inhibit the NWR. Immersion of the contralateral hand in ice water results in a clear inhibition of the NWR excitability [76,83] most likely due to activation of descending activity inhibiting dorsal horn neurons [42]. CPM also reduces the reflex temporal summation response [70]. Descending modulation of the NWR due to variation in cognitive activity has also been reported. This includes viewing pictures with strong emotional content [58], anticipatory cues [44], and hypnotic suggestions of analgesia [87]. A recent study has shown enlarged NWR responses following catastrophising self-statements but interestingly without concurrent modulation of the NWR temporal summation response [59]. Cognitive modulation, by changing the level of attention and distraction, has been shown to lead to reduced and enlarged RRF area, respectively [14], suggesting dynamic modulation of the excitability of deep dorsal horn neurons.
NWR excitability is often used as a biomarker in animal studies. Facilitated reflex responses have been observed following robust activation of nociceptive afferents close to the reflex testing site [34,65,84]. Translation of these models of acute reflex facilitation to humans has been less successful. The few examples available include reflex facilitation following topical application of capsaicin for 30–60 minutes [3,33] and a recent study where deep injections of capsaicin resulted in an expansion of RRF area in both spinal intact and complete spinal cord injured subjects [11].
The NWR has been used to investigate differences in spinal nociception related to the influence of supraspinal control by testing in spinal cord injured (SCI) individuals [37]. After spinal cord transection, the NWR becomes larger and changes into a stereotyped flexor pattern with flexion of all joints [25,31]. The NWR thresholds also become higher in in SCI volunteers compared to healthy volunteers [2,36]. In relation to the RRF, it is shaped by excitatory and inhibitory spinal neuronal circuits under supraspinal influence [64]. In volunteers with chronic SCI, the loss of descending control and appropriate peripheral input causes severe changes in the spinal circuitry [23], leading to an abnormal RRF expansion when supraspinal control is impaired and/or when spinal neurons are sensitized [2,24,32,66]. Moreover, a recent study demonstrated that people with complete SCI not only presented larger RRF areas, but also a reversed RRF topography compared to RRF of healthy volunteers, e.g., the most sensitive area for the dorsiflexor tibialis anterior in healthy volunteers is the arch of the foot, whereas the arch of the foot became the less sensitive area in people with complete SCI [11]. In any case, the RRF was still able to expand during experimental induction of central sensitization, possibly reflecting some spinal protective plastic mechanisms may still be partially functional without supraspinal control. A functional model of the NWR has been proposed (Fig. 2), integrating these new findings with existing knowledge on spinal and supraspinal neural networks involved in the control and modulation of the NWR and the RRF [35,60].
Assessment of Spinal Hyperexcitability in Pain Patients
Experimental and clinical studies in diverse cohorts of patients (e.g., whiplash, fibromyalgia, osteoarthritis, musculoskeletal disorders, headache, and neuropathic, visceral and post-surgical pain) have shown common traits (dynamic tactile allodynia, secondary punctate or pressure hyperalgesia, aftersensations, enhanced temporal summation and enlargement of referred pain areas) reflecting alterations in central pain processing [20,85]. These traits can be assessed using different experimental tests: verbal reports, pain questionnaires, light touch, vibration as well as detection, first pain and tolerance thresholds to pressure, electrical, heat and cold stimuli [41,56,71,74,75]. However, these tests are subjective in the sense that they rely on the volunteers’ reports after sensory stimulation, and thus they can be voluntarily and/or involuntarily affected by a number of factors, most notably the psychological distress associated with chronic pain conditions [16]. In this regard, there is increasing evidence that objective methods, such as those based on the NWR and the RRF, can detect hyperexcitability in the nociceptive pathways without the problems usually associated with subjective assessments.
FIGURE 2 Functional organization of the nociceptive withdrawal reflex (NWR) pathways. (A
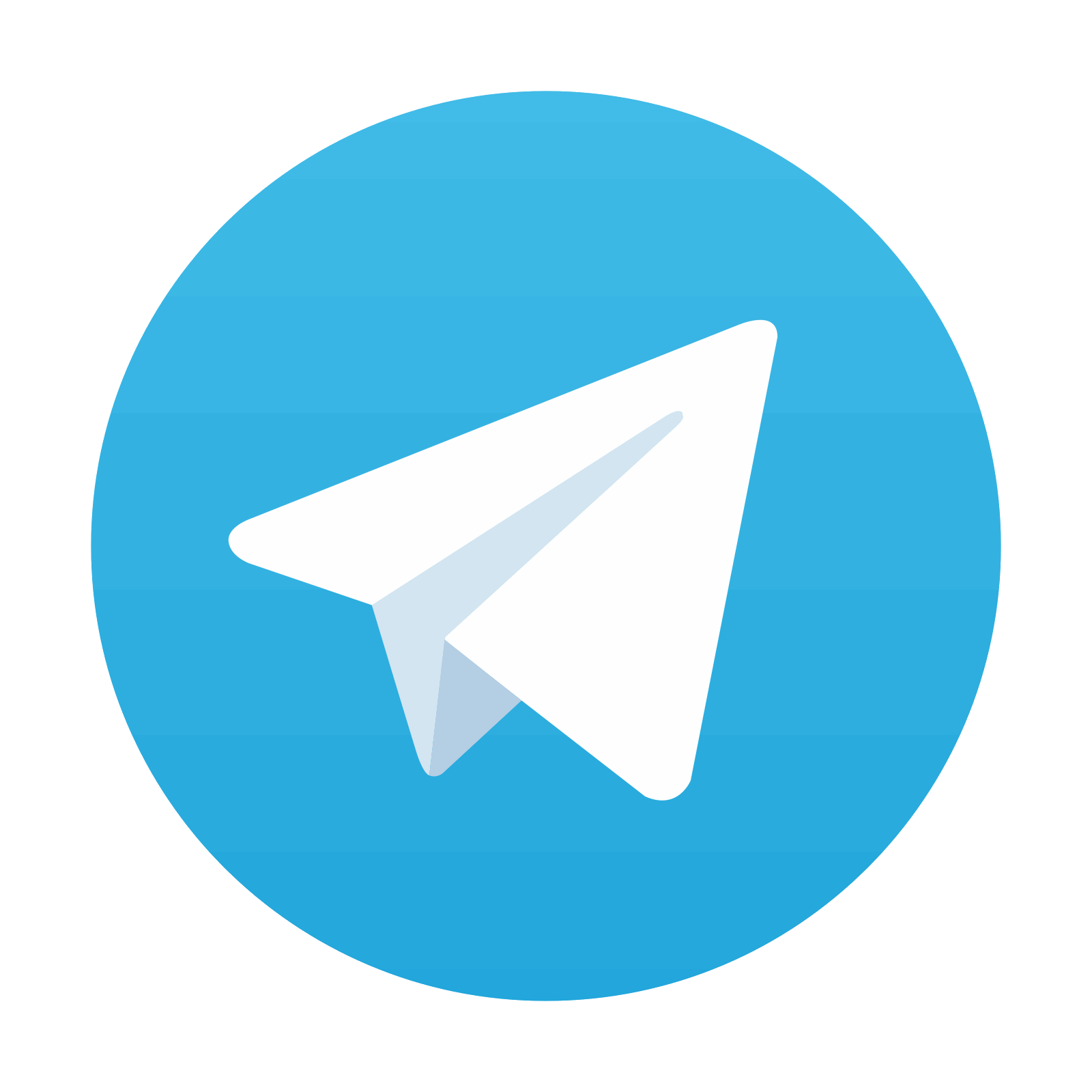
Stay updated, free articles. Join our Telegram channel

Full access? Get Clinical Tree
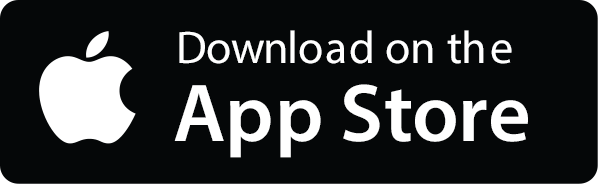
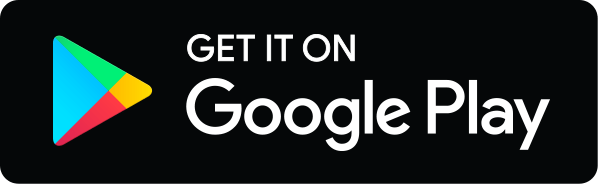