KEY POINTS
Aggressive and prolonged life support maneuvers should be performed as necessary on all electrical injury patients in the first few hours.
All patients are to be considered to have multisystem injuries, including cervical spine fracture, until such injuries are diagnostically eliminated.
Intravenous fluid resuscitation should not be underestimated.
Most patients should be monitored for cardiac dysrhythmias for 24 to 48 hours after injury, particularly if electrocardiographic abnormalities were present or persist.
The preservation of renal function depends largely on adequate volume resuscitation. If urine is visibly discolored by myoglobin, then renal function may depend on supplemental therapies.
The neurologic examination should be carefully monitored for seizure activity, which should be treated if it develops.
Early recognition and decompression of compartment syndromes are critical for maximizing extremity salvage and long-term function.
Adequate wound care necessitates complete debridement of nonviable tissue followed by wound closure as expeditiously as possible.
Electric shock is one of the leading causes of work-related injury, comprising 7% of all workplace fatalities. The typical victim of high-voltage electrical injury is a young industrial worker or lineman usually between the ages of 20 and 34, with 4 to 8 years experience on the job. Immediate death can result from cardiac dysrhythmia, central respiratory arrest, or asphyxia due to tetanic contraction of the muscles of respiration. If the victim survives the initial cardiopulmonary or central nervous system (CNS) insult, he then may face potential limb- and life-threatening sequelae from cutaneous injury, internal tissue destruction, and organ system dysfunction, requiring multidisciplinary intensive care at a specialized burn center. The distribution of the tissues and organs damaged depends on the path of the injury current. Frequently the injury is complicated by associated blunt trauma when the patient falls from a height or is thrown by the force of the electric current.
When the voltage is less than 1000 volts (V), direct mechanical contact is usually required for electrical contact. For high voltages (>1000 V), arcing usually initiates the electrical contact. Most electrical injuries are due to low-voltage (<1000 V) electrical shock. Whereas low-voltage shocks carry a significant risk of electrocution-induced cardiac arrest, high-voltage shock injury is characterized by extensive tissue damage, rather than electrocution. Approximately 1% to 4% of all US hospital burn unit admissions are for electrical injury, mostly a result of high-voltage (>1000 V) shocks.
The duration of contact with the high-voltage power source and the distribution of electrical current are important factors in the magnitude of the injury. If the contact is brief (ie, less than 0.5 seconds), cell damage can occur through nonthermal component of electrical injury, called electroporation.1 If the contact is longer, both heating caused by electrical conduction (joule heating) and electroporation play important roles. Prolonged contact can lead to thermal burning of tissues in the current’s path. The electrical current distribution across the tissues between the surface contact points depends on the electrical conductivity of the various tissues and on the variation in electric field intensity. Usually, current density is greatest at the contact points. Once the current travels away from the contact points into the subcutaneous tissues, the tissues with the least electrical resistance, that is, muscle, nerve, and blood vessels, will have the largest current densities and will experience the most rapid heating.2 As current tries to pass through bone, which has a high resistance, surrounding (deep) muscles are thermally injured.
Many cells, such as muscle and nerve cells, use electrical signals to control their function. The application of weak electric fields from a nonphysiologic source can interfere with cell function and, if the field is strong enough, cause direct cell damage. Electricity at frequencies below one megahertz may produce tissue injury primarily by permeabilization of cell membranes, electroconformational denaturation of cell membrane proteins, and thermal denaturation of tissue proteins. Factors that determine the anatomic pattern, extent of tissue injury, and the relative contribution of heat versus direct electrical damage include the amount of current, anatomic location, and duration of contact. The type of clothing, use of protective gear, and the power capability of the electrical source also contribute to the wide range in clinical manifestations observed in electrical shock victims.
At the commercial frequency of 60 Hertz (Hz), the threshold for human perception of a current passed hand to hand is approximately 1.0 milliampere (mA). As the current reaches 16 mA, the muscles in the arm develop involuntary spasms. Within 10 to 100 milliseconds (ms) of the onset of the current (the excitation-contraction response time of human skeletal muscles), muscles located in the current’s path will contract. If the hand is holding the conductor at that time, the strong forearm flexor muscles will contract, causing the victim to grasp the conductor strongly, thus maintaining uncontrollable contact with the current source. This is called the “no-let-go” phenomenon. Alternatively, if the victim is close to but not touching the conductor at the time of current passage, the strong muscle contractions generally propel him away from the contact. Judging from eyewitness reports, the latter phenomenon may be more common. In addition, a very high-energy electrical arc can produce a strong thermoacoustic blast force leading to significant barotrauma.
When a current of 60 mA or greater traverses the mediastinum, there is enough induced depolarization of myocardial membranes to cause cardiac arrhythmias, particularly if the induced depolarization occurs during early myocardial repolarization. When the current amplitude reaches 1500 mA through the upper extremity, skeletal muscle and peripheral nerve cells are damaged by electrical forces, independent of heat. Smaller currents, in the range of 200 to 500 mA, can generate enough joule heating to cause tissue damage if the duration of current passage is sufficient. The rise in serum creatine phosphokinase (CPK) levels that results may be a useful prognostic indicator.3
While the spectrum of electrical injury ranges from minor cutaneous trauma to severe multisystem injury, the victims of high-voltage electrical injury are usually the patients who are admitted to a critical care unit (see Tables 122-1 and 122-2).
Criteria for Admission of Electrically Injured Victims
Any of the following qualifies a patient for admission to an intensive care unit: |
|
Criteria for Transfer Out of Intensive Care Unit
All of the following criteria need to be met for safe transfer at 48 h: |
|
INITIAL EVALUATION
Upon arrival at a critical care facility, the ABCs of trauma (airway, breathing, and circulation) are assessed, and appropriate therapeutic maneuvers initiated. Cardiopulmonary resuscitation (CPR) is initiated or continued, as needed, and routine advanced trauma life support (ATLS) procedures and protocols are performed. Life-support activities should be continued for a prolonged period, as complete functional recovery after lengthy resuscitation efforts has been well documented.4 Precise time limits for the continuation of life support have not been elucidated.
After the patient is stabilized, a complete history should be obtained if possible, and a careful physical examination should be performed. Witnesses and family members often give pertinent information regarding the accident as well as significant medical history. On physical examination, particular attention should be paid not only to the sites of electrical contact but also to other areas of significant patient complaint. It is a misnomer to refer to electrical “entry” and “exit” sites. When the electrical source is an alternating current (eg, a home 60 Hz electrical socket), any point of physical contact will carry the current in and out of the body at 120 times per second. The location of surface contact points, which usually are full-thickness burns, allows the physician to establish the most likely pathway of the current and the region(s) of potential tissue damage. Obvious cutaneous injury is usually only the tip of a large soft tissue injury (iceberg theory). During resuscitation, electrical trauma victims frequently require large volumes of isotonic intravenous fluids, in excess of calculated needs. These large fluid requirements are due to considerable third-space losses secondary to deep or occult tissue damage. Unlike purely thermal burn injuries, resuscitation formulas such as the Parkland formula are not helpful guides to fluid management. Isotonic fluids should be given liberally, with the initial goal of resuscitation being a urine output of between 0.5 and 1 mL/kg/h. Any electrolyte abnormalities should be corrected quickly. If serum CPK is greater than 1000 and/or hemochromogens (such as myoglobin or free hemoglobin) are found in the urine, the rate of fluid administration is increased to achieve a goal urine output of 1.5 to 2.0 mL/kg/h. Consideration should also be given to the alkalinization of the urine and administration of mannitol. Alkalinization of the urine (to pH >6.5) may inhibit precipitation of myoglobin and hemoglobin in the renal collecting system.4,5 Mannitol, an osmotic diuretic, is generally used to aid in diuresis, wash out myoglobin in the renal tubules and expand intravascular volume.6,7 The recommended dosage is 12.5 g administered as an intravenous bolus. If hemochromogens persist in the urine after mannitol bolus therapy, then a mannitol infusion should be started at the rate of 12.5 g/h continuously until the urine clears of hemochromogens. Careful observation of electrolytes is required when a patient is treated with continuous mannitol infusion. Loop diuretics should rarely, if ever, be used to improve urine output in electrical injury patients.
Reliable, large-bore intravenous access is essential, as well as arterial blood pressure monitoring and a urinary catheter. There is no evidence to support the routine use of pulmonary artery catheterization. It may be helpful to view all victims of electrical trauma as potentially having multiple injuries. These patients should be evaluated as multisystem trauma victims. A large percentage of high-voltage electrical trauma patients have either fallen from a height or been thrown by the force of the electric current. Cervical spine as well as other orthopedic injuries should be suspected and sought, and therapy initiated as appropriate. A falling hematocrit or hemodynamic instability must be thoroughly investigated. Changes found by the mental status examination must be explained. An unstable level of consciousness cannot be attributed to changes secondary to electricity until any systemic hypoperfusion or surgically correctable head trauma has been eliminated. The initial physical examination should also include a careful evaluation and documentation of both the central and peripheral nervous systems. A paralyzed ventilated patient may need EEG monitoring to assess for seizure activity. The manifestation of neurologic deficits may be delayed, so these evaluations should be repeated daily.
Appropriate tetanus prophylaxis is provided as delineated by the American College of Surgeons Committee on Trauma guidelines. Appropriate evaluation and management of corneal injury and tympanic membrane rupture should be instituted.
CARDIAC
Lethal ventricular dysrhythmias are a major cause of immediate mortality from electrical injury. If an initial dysrhythmia is corrected and the patient is hemodynamically stabilized, recurrence of a potentially fatal dysrhythmia is unusual unless a cardiac pathology exists. As stated earlier, it is important to remember that electrical injury victims who require CPR because of a dysrhythmia should be given prolonged ACLS, as reports of complete functional survival after significant periods of CPR do exist.8
Close to 50% of patients exhibit electrocardiographic (ECG) changes or rhythm disturbances after injury. The most common ECG alterations are nonspecific ST-T wave changes and sinus tachycardia, which usually revert with time. Most dysrhythmias are transient, and therapeutic intervention is rarely needed. The difficulty lies in identifying the existence of new myocardial damage and determining its physiologic significance. Some patients may suffer long-term damage to the conductive system.9
The usual clinical diagnostic criteria for myocardial infarction include ECG changes and elevation of cardiac isoenzyme levels in a setting compatible with myocardial ischemia. These pieces of evidence are not reliable, however, in the circumstance of electrical injury. ECG abnormalities after electrical trauma are common, temporary, and usually physiologically insignificant. The levels of the creatine phosphokinase (CPK) MB isoenzymes may be elevated owing to large-scale muscle damage and may give a false impression of myocardial damage.10 Troponin levels may be more helpful to help delineate myocardial injury.11
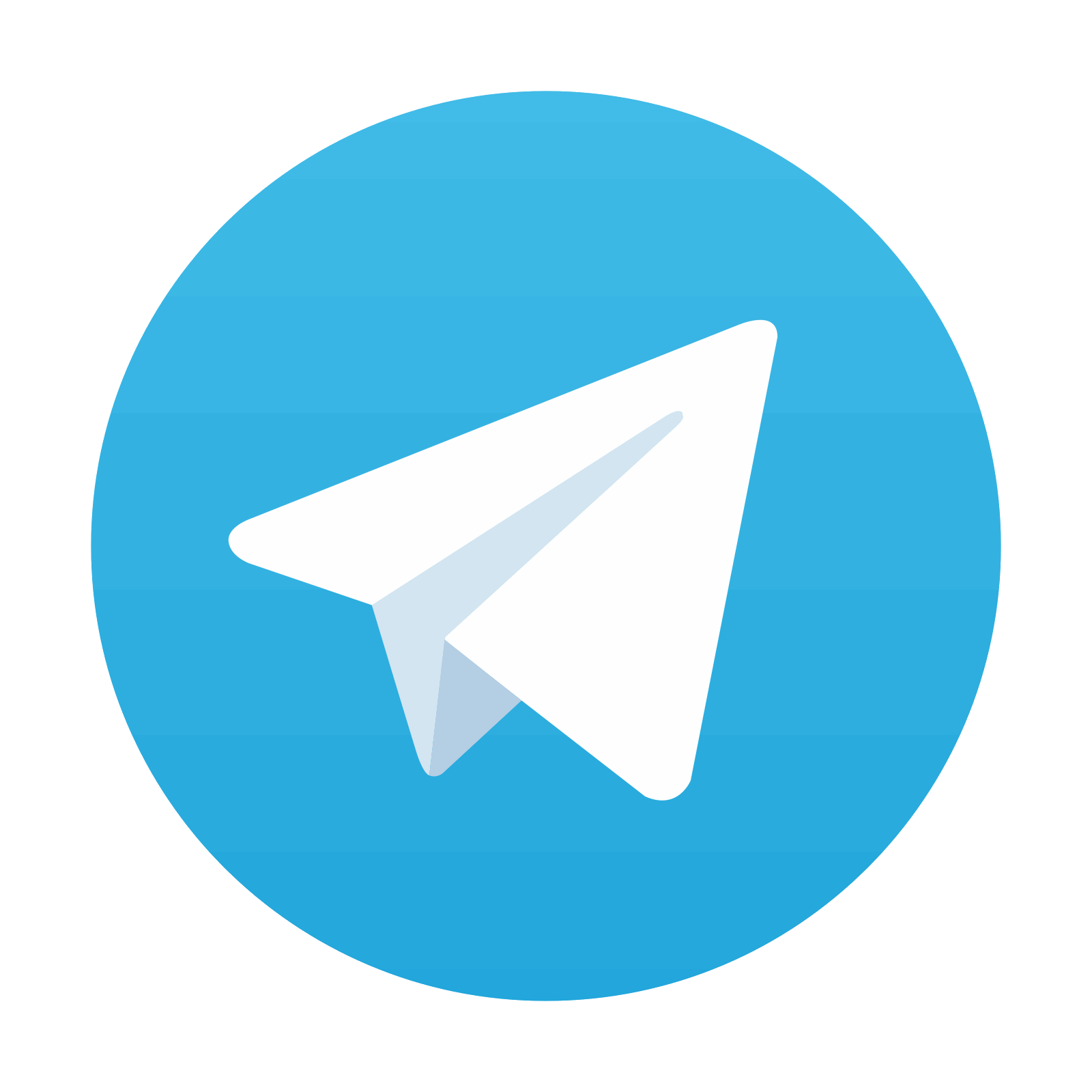
Stay updated, free articles. Join our Telegram channel

Full access? Get Clinical Tree
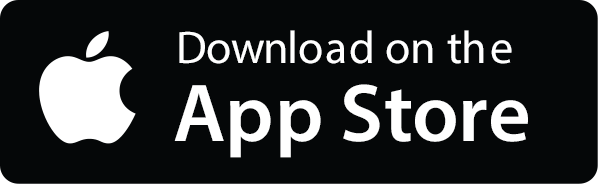
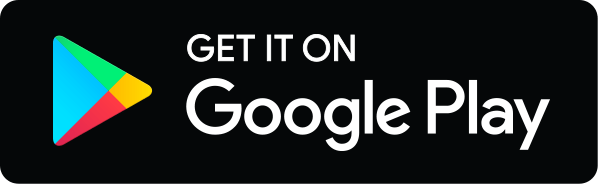
