The graph above depicts the relationship between current, frequency and biological effect on sensation and muscle contraction. At high frequencies, greater current flow is required to cause muscle contraction and sensation than at 60–100 Hz. This is because at high frequencies, polarity reverses too rapidly to allow time for ion movement. This principle explains the relative safety of diathermy, as it uses high-frequency current to minimise the electric shock risk. However, high-frequency AC is still capable of producing significant heating effects leading to thermal injuries.
Shock hazards can occur via resistive or capacitative coupling. Resistive coupling is the process where an earthed individual completes a circuit, by making direct contact with a live object, such as the casing of faulty equipment. Capacitative coupling occurs when an object is close to a strong AC source, such as an operating light. The AC source and the object form two plates of a capacitor, separated by air (the dielectric). This allows alternating current to flow, resulting in shock and thermal hazards.
Macroshock occurs when a person forms part of an electrical circuit, resulting in current flow through them. This can cause arrhythmias, involuntary muscle contraction (including respiratory muscles), and altered nervous system function (confusion and coma). The severity of macroshock depends on the strength and type of current, the path of current flow through the body, the duration of exposure and the timing of shock in relation to the cardiac cycle.
Current strength is determined by potential difference divided by impedance. Skin and clothing are the main sources of impedance. For a given potential difference, the amount of current flow will be greater if the impedance is low rather than high. Wet skin has lower impedance than dry skin, because water usually contains free ions within it, which improves conduction. Increased contact area and intravenous lines (breaching the skin) also lower impedance.
Low-frequency AC (around 60 Hz) is more likely to cause ventricular fibrillation (VF) than higher frequencies or DC. High-frequency AC causes fewer ion shifts, while with DC a unidirectional ion shift across the myocytes is produced that most often results in ventricular standstill. Complete recovery from DC-induced ventricular standstill is possible if the exposure is brief.
Alternating current flowing across the chest can trigger VF, particularly if it reaches the heart during the repolarisation phase. If the current is sufficient to disrupt the normal conduction pathway in part of the heart, that area will no longer depolarise and repolarise via the normal conduction pathway. Instead, electrical activity triggered aberrantly will result in depolarisation of adjacent segments of the ventricles in a random manner, causing VF.
Microshock occurs when electrical current is transmitted directly to the myocardium. This may occur via a central venous catheter, pacemaker lead, or a temperature probe in the oesophagus. The direct electrical connection to the heart means small currents in the region of 50–100 μA are sufficient to induce VF. Microshock usually arises from leakage currents in line-operated equipment, particularly if there is a fault present in the ground system.
The following table describes the potential physiological effects of electric shock produced by increasing current levels at 60 Hz.
Mean current (mA) | Physiological effect |
---|---|
1 | Threshold of perception |
5 | Pain |
8 | Burns |
15 | Muscle contraction |
50 | Respiratory arrest and severe burns |
100 | Ventricular fibrillation |
0.05 (50 μA) | Microshock (if cardiac connection exists) |
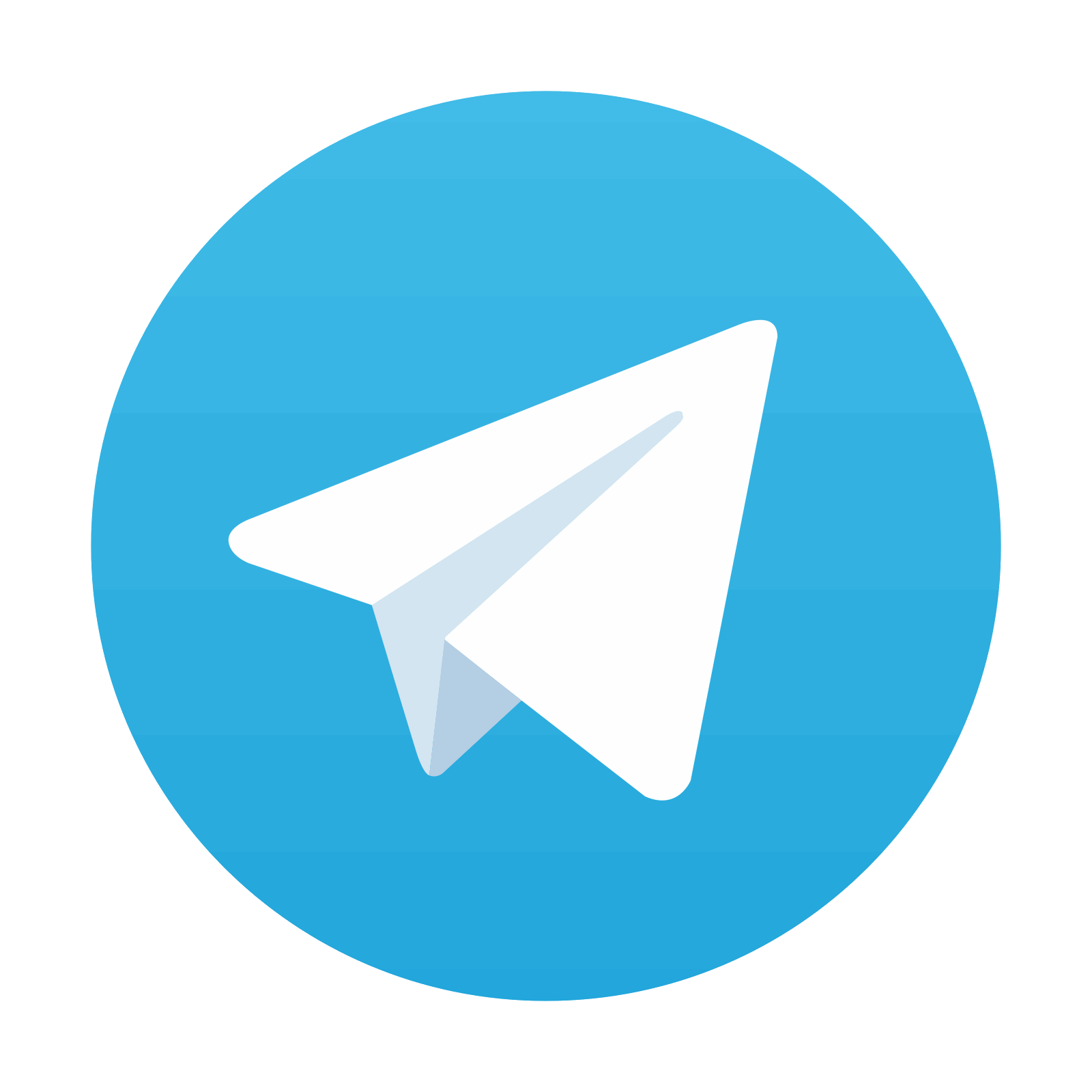
Stay updated, free articles. Join our Telegram channel

Full access? Get Clinical Tree
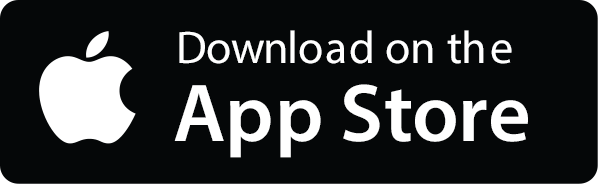
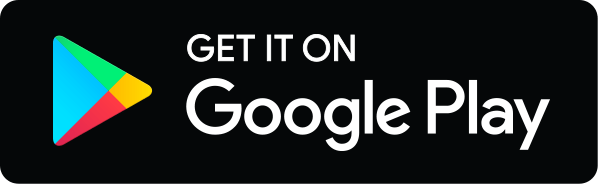