Echocardiography in the Intensive Care Unit
Achikam Oren-Grinberg
Sajid Shahul
Adam B. Lerner
Introduction
Echocardiography was introduced to the operating suite in the 1970s, with epicardial echocardiography as its initial application. Transesophageal echocardiography (TEE) during surgery was first described in 1980 but did not become commonplace until the mid-1980s. Since then, TEE has evolved to become a widely used and versatile modality for diagnosis and monitoring of critically ill patients. As such, its use has expanded into the perioperative period and the intensive care unit (ICU). Echocardiography provides both anatomic and functional information about the heart; systolic and diastolic function, cavity size, and valvular function [1].
Ease of use, availability of diagnostic information within 10 to 15 minutes from the start of examination, high-quality imaging in most patients, and low complication rates have all led to the pervasive use of echocardiography in the perioperative environment and increasing use in the ICU [2,3,4,5,6,7,8]. However, patient safety and optimal outcome depend heavily on a thorough understanding of both the strengths and limitations of the available technologies and their applications.
Basic Terminology of Echocardiography Techniques
A sonographer must use different echocardiographic imaging techniques and hemodynamic modalities to achieve a diagnosis or management plan. The following is a list of the basic techniques used during an echocardiographic study.
Two-Dimensional Echocardiography
Two-dimensional (2D) echocardiography is the backbone of the echocardiographic examination [9]. Using 2D, a complete visualization of the beating heart is achieved by displaying anatomic structures in real-time tomographic images. By aiming the ultrasound probe at the heart, exactly oriented anatomic “slices” are obtained. Information acquired includes cardiac chamber sizes, global and regional systolic function, and valvular anatomy.
M-Mode Echocardiography
M-mode or motion-mode images are a continuous 1D graphic display that can be derived by selecting any of the individual sector lines from which a 2D image is constructed [9]. It is useful for quantification of myocardial wall and chambers sizes, which in turn can be used to estimate left ventricle (LV) mass and chamber volumes, respectively. Though very limited, M-mode can also be used to determine fractional shortening, a rough estimate of left ventricular systolic function. In addition, since it has high temporal resolution, M-mode is helpful in assessing the motion of rapidly moving cardiac structures such as cardiac valves.
Doppler Echocardiography
Doppler echocardiography is used to supplement 2D and M-mode echocardiography. It can provide functional information regarding intracardiac hemodynamics; systolic and diastolic flows, blood velocities and volumes, severity of valvular lesions, location and severity of intracardiac shunts, and assessment of diastolic function. The four types of Doppler modalities used include continuous-wave, pulsed-wave, color flow mapping, and tissue Doppler [9]. Continuous-wave Doppler is used for measuring high-pressure gradient/high-velocity flows such as seen in aortic stenosis. When using continuous wave Doppler, the ultrasound probe continuously transmits and receives sound waves. This increases the maximum limit of blood velocity that can be evaluated before exceeding the Nyquist limit. The Nyquist limit represents the maximum flow velocity that can be evaluated by Doppler and is dependent on both equipment and imaging variables. Continuous wave Doppler can evaluate higher flows but does so at the expense of spatial specificity. This is referred to as “range ambiguity.” Pulsed-wave Doppler is used for measuring lower-pressure gradient/lower-velocity flows such as in mitral stenosis. In this mode, the ultrasound probe sends out a pulse of sound and then waits to receive reflected waves. This lowers the Nyquist limit and the maximum velocities that can be interrogated but allows for precise spatial resolution. Color flow mapping is useful for screening valves for stenosis or regurgitation, quantifying the degree of valvular regurgitation, imaging systolic and diastolic flow, and detection of intracardiac shunts. Doppler tissue imaging has been introduced as a new method of quantifying segmental and global left ventricular function. It records systolic and diastolic velocities within the myocardium and at the corners of the mitral annulus and is useful for studying diastolic function and contractile asynchrony of the LV [10].
Contrast Echocardiography
Contrast echocardiography is used to enhance the diagnostic quality of the echocardiogram [11]. It may be used to improve assessment of global function and regional wall motion abnormalities by 2D echocardiography. Although approved only for LV opacification, recent clinical studies suggest a potential use in assessing myocardial perfusion [12,13].
Transesophageal Versus Transthoracic Echocardiography
Although transthoracic echocardiography (TTE) is a less invasive way to image cardiac structures, suboptimal acoustic windows lead to low-quality images in many critically ill patients. These suboptimal acoustic windows are due to obesity, pulmonary disease, the presence of chest tubes, drains and wound dressings, and limitations on patient positioning. Using TTE in the ICU can be challenging; one study found the echocardiographic examination to be inadequate in approximately 50% of patients on mechanical ventilation and 60% of all ICU patients [8]. The relatively low percentage of adequate imaging improves when TTE is used as a monitoring tool, which does not require the same quality of images, and not as a diagnostic tool. In a report of more than 200 ICU patients, TTE used as a monitoring tool provided 2D images of acceptable quality in 97% of patients [14].
In contrast to TTE, TEE is more invasive but consistently provides images of better quality. In up to 40% of patients, TEE may provide additional unexpected diagnoses that are missed by TTE [4,15]. Recent advances in ultrasound imaging, which include harmonic imaging, digital acquisition, and contrast endocardial enhancement, have improved the diagnostic yield of TEE [16,17].
Contraindications to Performing Tee
Although TEE is safe [18,19], there are several contraindications to probe insertion. These include significant esophageal or gastric pathology; mass or tumors, strictures, diverticulum, Mallory-Weiss tears, recent esophageal or gastric surgery, upper gastrointestinal bleeding, and dysphagia or odynophagia not previously evaluated. Esophageal varices are not an absolute contraindication, and a risk–benefit analysis of each case must be carried out before performing TEE in any individual patient [20]. Practitioners must be aware of the potential for severe bleeding, in particular when a coagulation abnormality exists. Cervical spinal injury is another relative contraindication requiring careful risk–benefit analysis.
Complications and Safety of Tee
TEE is considered a moderately invasive procedure and complications are rare. In one study of ICU patients, complication rates reached 1.6% and included hypotension following sedation for probe insertion, oropharyngeal bleeding in a coagulopathic patient, and aspiration during tracheal intubation performed prior to TEE [19]. Another study in 2,508 ICU patients reported a complication rate of 2.6%. In this study, there was no examination-related mortality. Complications included
transient hypotension or hypertension, circulatory deterioration, hypoxemia, arrhythmias, vomiting, coughing, superficial mucous membrane lesions, displacement of a tracheostomy tube and accidental removal of a duodenal feeding tube [18]. A large European multicenter study of 10,419 examinations reported a complication rate of 2.5% with one (0.01%) case of fatal hematemesis due to a malignant tumor [2]. In addition, in 0.88% of the reported cases, the TEE exam had to be prematurely terminated due to either patient intolerance or because of cardiac, pulmonary, or bleeding events [2].
transient hypotension or hypertension, circulatory deterioration, hypoxemia, arrhythmias, vomiting, coughing, superficial mucous membrane lesions, displacement of a tracheostomy tube and accidental removal of a duodenal feeding tube [18]. A large European multicenter study of 10,419 examinations reported a complication rate of 2.5% with one (0.01%) case of fatal hematemesis due to a malignant tumor [2]. In addition, in 0.88% of the reported cases, the TEE exam had to be prematurely terminated due to either patient intolerance or because of cardiac, pulmonary, or bleeding events [2].
Common Indications for TEE in the ICU
In 1996, a task force created by the American Society of Anesthesiologists and the Society of Cardiovascular Anesthesiologists published guidelines regarding the indications for TEE [21]. Three categories of evidence-based clinical indications were identified. For indications grouped into category I, TEE was judged to be frequently useful in improving clinical outcomes. To date, there is only a single category I indication for TEE in the ICU. That indication is for “unstable patients with unexplained hemodynamic disturbance, suspected valve disease, or thromboembolic problems (if other tests or monitoring techniques have not confirmed the diagnosis or patients are too unstable to undergo other tests)” [21]. This indication, however, encompasses a significant proportion of ICU patients and in practice, clinicians use echocardiography in the ICU for many other indications. These are summarized in Table 29.1.
Echocardiographic Evaluation of Hemodynamic Instability
Hemodynamic instability is an extremely common event in every ICU. Determining the cause of such can sometimes be more challenging than one would expect. Echocardiography can be used successfully in the diagnosis, monitoring, and management of the unstable patient in the ICU. Using echocardiography to determine the etiology of hemodynamic instability requires assessment of cardiac function, volume status, valvular function, and extracardiac processes.
Assessment of Cardiac Function
Systolic dysfunction of either ventricular chamber must be considered in every unstable patient. The etiology of dysfunction may often times be discerned from the echocardiographic evaluation allowing for appropriate therapy to be initiated.
Table 29.1 Common Indications for Performing Tee in the ICU | ||||||||||
---|---|---|---|---|---|---|---|---|---|---|
|
Assessment of Left Ventricular Systolic Function
Use of several echocardiographic assessment modalities is necessary for evaluation of left ventricular systolic function. These modalities include quantitative as well as qualitative assessments.
Quantitative Assessment of Left Ventricular Systolic Function
Volumetric Method Using Geometric Models
Quantitative assessment of left ventricular systolic function relies on volume assessment using 2D tomographic images. To determine the volume at end diastole (LVEDV) and end systole (LVESV), the endocardial borders in two orthogonal tomographic planes are traced at end diastole and end systole. Several geometric assumptions and formulas have been developed (e.g., truncated ellipse, “bullet” formula, cylinder, and cone) to determine the LVEDV and LVESV based on these 2D images. Once LVEDV and LVESV have been determined, the stoke volume, and thus cardiac output (CO) can be calculated:

In addition, ejection fraction (EF) can be calculated from these volumes using the formula:

These formulas work optimally in a symmetrically contracting ventricle; the presence of regional wall motion abnormalities decreases accuracy. In addition, foreshortening of the LV cavity is a common source of underestimation of LV end-diastolic and end-systolic volumes and can similarly impact the accuracy of systolic function assessment with these formulas [1,22]. Lastly, since the models depend on accurate endocardial border definition, their use requires adequate visualization. Incomplete endocardial definition is described in 10% to 20% of routine echocardiographic studies [23] and may reach 25% in ICU patients [24]. This challenge is even greater in patients requiring mechanical ventilation in which imaging can be particularly challenging. These challenges have limited the use of the geometric models and formulas for assessment of LV systolic function.
Discs Method (Simpson’s Rule)
Another method for volumetric assessment of LV systolic function is the discs method, which may be more accurate than the other volumetric methods described above, particularly in the presence of distorted LV geometry [9]. In this method the ventricle is divided into a series of discs of equal height and each disc volume is calculated as follows:

The ventricular volume can be calculated from the sum of the volumes. This technique requires true apical images, which in clinical practice may be difficult to achieve. Foreshortening of the ventricular apex will result in inaccurate assessment of the left ventricular EF and CO (Fig. 29.1).
Qualitative Assessment of Left Ventricular Systolic Function
2D Evaluation of Ventricular Systolic Function
Using 2D imaging, two of the most important questions regarding hemodynamic stability can be rapidly answered; are the ventricles contracting well and are they adequately filled. Using 2D, an experienced observer can qualitatively evaluate systolic function. This should be assessed from multiple tomographic planes and attention must be given to obtaining adequate endocardial definition. Normal ventricular contraction consists of simultaneous myocardial thickening and endocardial excursion toward the center of the ventricle. It is important to look for this myocardial thickening; infarcted myocardium may be pulled inward by surrounding, normal myocardium. There is some regional heterogeneity of normal wall motion with the proximal lateral and inferolateral (or posterior) walls contracting somewhat later than the septum and inferior wall [25]. For qualitative assessment of overall systolic function, the echocardiographer integrates the degree of wall thickening and endocardial motion in all tomographic views and reaches a conclusion about overall LV systolic function and EF. Although different institutions use different standards, severe LV systolic dysfunction is usually defined as an EF < 30%, moderate dysfunction 30% to 45%, mild depression 45% to 55%, and normal > 55%. This method of EF estimation is of great clinical utility and can be performed with good correlation to quantitative measurements. There are however, a few potential pitfalls to 2D assessment of EF that must be considered:
Accurate assessment requires satisfactory endocardial border definition. Qualitative EF estimation becomes inaccurate when the endocardium is inadequately defined.
Accurate estimation of EF depends on the experience of the echocardiographer.
In asynchronous contraction (paced-rhythm, conduction defects, etc.), assessment of EF is more difficult.
Despite its limitations, 2D qualitative assessment is the most widely used technique for assessment of LV systolic function due to its ease of application in the clinical setting. In the operating room, after completing the TEE exam, most physicians monitor LV systolic function continuously with 2D imaging using the transgastric (TG) midpapillary short-axis view. This allows for quick assessment of regional wall motion abnormalities in all coronary arterial circulatory beds as well as rudimentary evaluation of volume status [26]. However, it is important to remember that this view alone is never satisfactory for assessing overall systolic function.
Regional Left Ventricular Function
Most commonly, abnormal regional wall motion is the result of coronary artery disease and resultant ischemia/infarction. Abnormal wall motion is a continuum of conditions consisting of hypokinesis, akinesis, and dyskinesis. With dyskinesis, the affected wall segment moves away from the center of the ventricle during systole. To standardize echocardiographic evaluations of wall motion, a 17-segment model of the LV has been defined [25]. These 17 segments are evaluated separately for the presence and degree of regional wall motion abnormality. When the etiology of the wall motion abnormality is CAD, the location of the coronary lesion can be usually predicted from the location of the regional wall motion abnormality.
Contrast Echocardiography
Recent innovations have been made to overcome some of the technical obstacles related to endocardial border detection and image quality. Intravenous echocardiographic contrast agents that opacify the left side of the heart can markedly improve visualization of the LV cavity and enhance endocardial definition. These agents can aid assessment of regional and global LV functions [27,28,29,30]. They also have the potential to “salvage” nondiagnostic TTEs in ICU patients. One study demonstrated a “salvage” rate of 51% [31] and another 77% of nondiagnostic TTEs [32]. In addition to improving visualization and assessment of LV function, assessment of myocardial perfusion defects with intravenous contrast has been reported with various imaging techniques and modalities [33,34,35].
Doppler Assessment of Left Ventricular Systolic Function
Doppler spectral profiles can be used to evaluate left ventricular function quantitatively. This evaluation of left ventricular
systolic function is based on calculation of stroke volume (SV) and CO.
systolic function is based on calculation of stroke volume (SV) and CO.
Stroke Volume—the volume of blood ejected during each cardiac cycle is a key indicator of cardiac performance. SV can be calculated by using pulse wave Doppler (PWD) to measure the instantaneous blood velocity recorded during systole from an area in the heart where a cross-sectional area (CSA) can be easily determined. The left ventricular outflow tract (LVOT) is most commonly used because its cross section is essentially a circle. By measuring the diameter of the LVOT and assuming a circular geometry, the CSA is calculated as π(D/2)2. Any cardiac chamber or structure that has a measurable CSA may be used; mitral valve annulus, right ventricular outflow tract outflow, and tricuspid annulus are some examples. By tracing the outline of the PWD profile, the echocardiographic computer can calculate the integral of velocity by time or the velocity–time integral (VTI). The VTI is the distance (commonly referred as the stroke distance) that the average red cell has traveled during the systolic ejection phase. SV (cm3) is then calculated by multiplying the VTI (stroke distance in cm) by the CSA in cm2of the conduit (i.e., LVOT, aorta, mitral valve annulus, pulmonary artery) through which the blood has traveled [36,37,38,39,40,41,42]; SV = CSA × VTI. CO is then easily derived by multiplying the calculated SV by the heart rate: CO (cm3/min) = SV × HR (Fig. 29.2).
This approach to SV and CO calculations has shown very good correlation with thermodilution-derived CO measurements [43]. There are however, several potential sources of error:
CSA determination often leads to the greatest source of error. When using any diameter for CSA determination, any error in measurement will be squared (CSA = π(D/2)2). This translates to a 20% error in calculation of CO for each 2-mm error when measuring a 2.0-cm diameter LV outflow tract [25]. Studies have shown that while the Doppler velocity curves can be recorded consistently with little interobserver measurement variability (2% to 5%), the variability in 2D LVOT diameter measurements for CSA is significantly greater (8% to 12%) [44].
The Doppler signal is assumed to have been recorded at a parallel or near parallel intercept angle, called θ, to blood flow. The Doppler equation has a cos θ term in its denominator. With an intercept angle of 0 degree, the cos θ term equals 1. Deviations up to 20 degrees in intercept angle are acceptable since only a 6% error in measurement is introduced.
Velocity and diameter measurements should be made at the same anatomic site. When the two are measured at different places, the accuracy of SV and CO calculations is decreased.
Although the pattern of flow is assumed to be laminar, in reality the flow profile is parabolic. This does have some impact on velocity-based calculations [25]. However, in routine clinical practice this factor is of little significance and can be essentially ignored.
Determination of Left Ventricular dP/dt
The changing rate of left ventricular pressure (dP/dt) is an important parameter in the assessment of myocardial systolic function. Traditionally, dP/dt was derived from the left ventricular pressure curve acquired at cardiac catheterization using a micromanometer catheter recording. It has been shown that echocardiography can be used accurately and reliably to assess dP/dt by performing Doppler assessment of mitral regurgitant jet [45,46]. Using continuous wave Doppler, a spectral display of the mitral regurgitation (MR) jet is obtained. From the spectral display, information about the rate of pressure development within the LV can be derived using measurements undertaken in the early phase of systole (the upstroke of the velocity curve is used for calculations). Determination of dP/dt using the MR spectral jet is done by calculation of the time required for the MR jet velocity to go from 1 m per second to 3 m per second. The time between these two points represents the time that it takes for a 32 mm Hg pressure change to occur in the left ventricular cavity. This is based on the modified Bernoulli equation (P = 4v2), which relates pressure to velocity. Thus, in going from 1 m per second to 3 m per second:

where vB is velocity of 3 m/s.
dP/dt is then is calculated using the formula:

A depressed ventricle will take a longer time to develop this pressure gradient—a lower dP/dt. Normal dP/dt value is > 1,200 mm Hg per second (or time ≤ 27 milliseconds),
moderately depressed systolic function value range between 1,000 and 1,200 mm Hg per second, and when dP/dt is decreased bellow 1,000 mm Hg per second, left ventricular systolic function is severely depressed [47,48].
moderately depressed systolic function value range between 1,000 and 1,200 mm Hg per second, and when dP/dt is decreased bellow 1,000 mm Hg per second, left ventricular systolic function is severely depressed [47,48].
Assessment of Patient Volume Status
One of the most challenging and crucial tasks in the management of a hemodynamically unstable patient is to predict accurately whether the patient would benefit from fluid therapy. Overhydration may lead to pulmonary edema, hypoxia, and worsening outcome and therefore should be avoided. A novel and effective method for determining this “fluid responsiveness” is through the assessment of “dynamic parameters.” Examples of such dynamic parameters are stroke volume variation (SVV) and pulse pressure variation (PPV). These parameters can be readily assessed with echocardiography.
SVV and PPV are caused by the interaction of the cardiac and respiratory systems; that is, the changes in intrathoracic pressure during controlled ventilation have an impact on SV and therefore, arterial pressure. The increase in intrathoracic pressure during the inspiratory phase of positive pressure ventilation leads to simultaneous but different physiologic effects on the left and right sides of the heart. In the left side, SV increases as blood is pushed forward out of the pulmonary veins into the LV. In addition, the increased intrathoracic pressure leads to improved “afterload matching” for the LV, which increases SV by functionally decreasing afterload. The interventricular septum is shifted toward the right ventricle (RV) also increasing LV SV. On the right side, right ventricular inflow decreases secondary to compression of the inferior vena cava (IVC). The rightward shift of the septum also decreases RV SV. At the beginning of the exhalation phase, SV decreases since both the pulmonary veins and the RV are relatively “empty.” In hypovolemic patients, the magnitude of these cyclical or dynamic changes is increased and this serves as the basis for the accurate assessment of fluid responsiveness using these parameters [49,50,51,52,53,54].
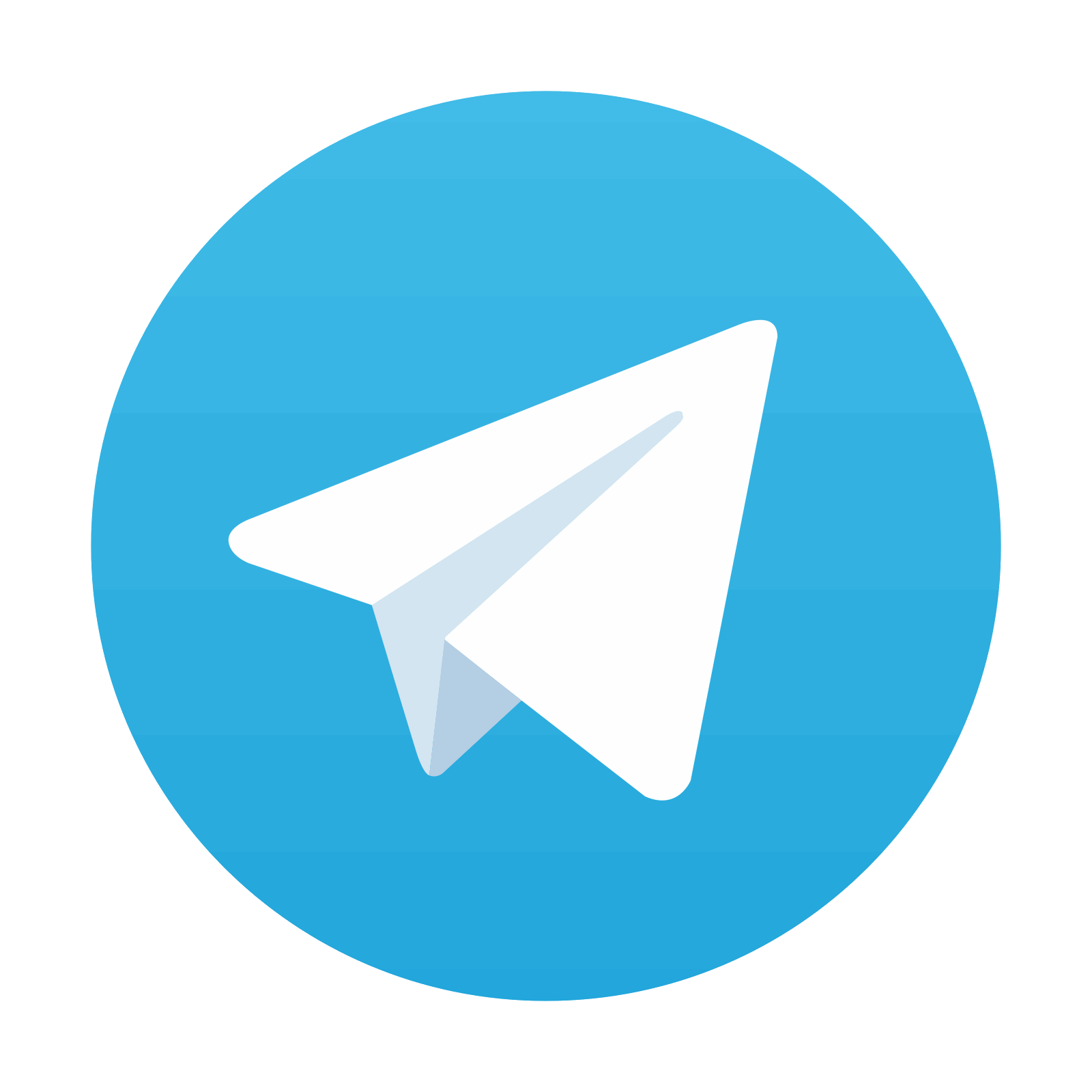
Stay updated, free articles. Join our Telegram channel

Full access? Get Clinical Tree
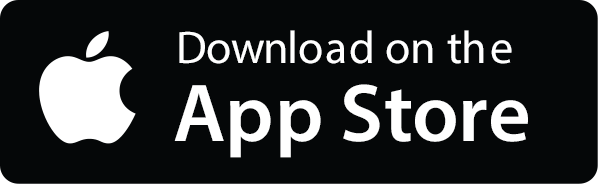
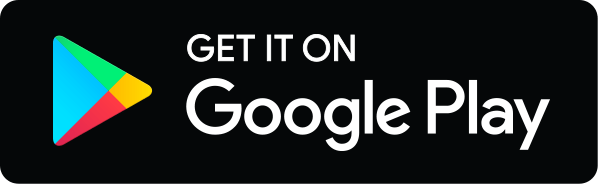