Diseases involving the aorta can present a challenge to both surgeons and anesthesiologists. Aortic dissection and rupture are life threatening, require rapid and accurate diagnosis, and need definitive medical and/or surgical management due to their high risk of morbidity and mortality.1,2 A key ingredient in the efficient management of these patients is imaging of the thoracic aorta. Transesophageal echocardiography (TEE) has become an essential noninvasive diagnostic modality for acute thoracic aortic pathologies, and is a standard part of the echocardiographer’s armamentarium in the operating room.3–6 It is important for the echocardiographer to quickly and accurately verify the diagnosis, distinguish true pathology from the many common confounding artifacts, and clearly communicate precise echocardiographic findings of the aorta and related cardiac anatomy to the surgeon in order to guide intervention. The following text reviews aortic anatomy and pathology and associated echocardiographic features that assist with imaging during aortic surgery.
In order to truly appreciate the invaluable role that TEE plays in the assessment for diseases of the aorta, a detailed understanding of the aorta and surrounding anatomic structures is crucial. The thoracic aorta can be divided into three anatomic segments: ascending thoracic aorta, aortic arch, and descending thoracic aorta (Figure 16–1). The ascending thoracic aorta originates at the level of the aortic valve annulus. As previously described in Chapter 9, the aortic valve comprises three crescent-shaped leaflets that coapt to form three commissures. Immediately distal to the aortic valve apparatus is a short and dilated aortic segment—the sinus of Valsalva—which is subdivided into the noncoronary, left coronary, and right coronary sinuses. As the nomenclature suggests, the left and right coronary arteries each originate from their respectively named sinus. Distal to the sinus of Valsalva, the aorta slightly narrows, forming the sinotubular junction (STJ). From this point, the ascending aorta crosses beneath the main pulmonary artery, then courses in an anterior, cranial, and rightward direction over the origin of the right pulmonary artery.
Figure 16-1.

Anatomic course of the thoracic aorta. The relationship with the esophagus is particularly important with regard to orientation of the probe and the aorta in each of its thoracic sections: the ascending aorta, aortic arch, and descending aorta. The interposition of the trachea makes portions of the ascending aorta and arch either completely invisible or partially visible.
The ascending aorta terminates and continues as the aortic arch at the origin of the brachiocephalic (innominate) artery. The aortic arch then proceeds to curve in a posterior and leftward direction with cranial convexity. Three arteries arise from the aortic arch: the brachiocephalic, left common carotid, and left subclavian arteries. It is often difficult to visualize the distal ascending thoracic aorta and proximal aortic arch with TEE because the trachea is positioned between the esophagus and aorta, effectively preventing ultrasound transmission. Immediately beyond the origin of the left subclavian artery, at the point of attachment of ligamentum arteriosum (remnant of the fetal ductus arteriosus), is a second narrowing called the aortic isthmus. Unlike the heart and proximal part of the aorta, the aortic isthmus and descending thoracic aorta are relatively fixed. Consequently, deceleration injury secondary to trauma is most often confined to this level. Distal to the aortic isthmus, the descending aorta follows a caudal, slightly anterior, and rightward trajectory towards the aortic diaphragmatic hiatus. Along its intrathoracic course, the descending thoracic aorta and the esophagus are in close proximity. While the esophagus courses almost straight downward, anterior to the midline of the vertebral bodies, the aorta travels in a smooth, curved direction from the anterolateral side of the 4th thoracic vertebral body to the anterior side of the 11th vertebral body.
During its thoracic descent, multiple intercostal arteries branch off the aorta and may occasionally be imaged with TEE using color-flow Doppler (CFD). Spinal branches of these intercostal arteries supply blood to the spinal cord through radicular arteries. The radicular artery anatomy in this area is quite variable, with 4 to 10 radicular branches typically contributing to the thoracic spinal cord. The anterior spinal cord blood supply is tenuous in the thoracic region, thus it is at great risk for cord ischemia. Frequently, one radicular artery—the arteria radicularis magna, or the artery of Adamkiewicz—is very developed and is responsible for the majority of anterior spinal cord blood supply, and it is typically found between T9 and T12.
Below the diaphragm, the abdominal aorta lies posterior to the stomach. Because the stomach is a large cavity that is highly deformable, the position of the abdominal aorta in relation to the intragastric TEE probe is somewhat variable. The celiac artery and mesenteric arteries originate from the anterior side of the abdominal aorta. The renal arteries arise from the left and right sides of the aorta, slightly below the mesenteric vessels.
The wall of the aorta is composed of three tunicae: the intima, media, and adventia. The inner layer, the intima, consists of simple squamous epithelium and underlying connective tissue. The tunica media consists of circularly arranged smooth muscle and elastic tissue. The outer adventitial layer is mainly a loose layer of connective tissue, lymphatics, and vasa vasorum (ie, “vessels of the vessels”). TEE provides the ability to assess the aortic wall for many pathologies including thickening of the tunica intima due to arteriosclerosis and/or atherosclerosis, intimal tears/dissections, and aneurysmal dilatation.
As described in Chapter 5, insertion of the TEE probe must be performed gently and should never be forced through areas of resistance. This is especially important in patients with suspicion of major aortic pathology. First, intubation of the esophagus with the TEE probe can be very stimulating and may result in hypertensive episodes, increasing the risk of further tearing or rupture of a dissection or aneurysm. Second, resistance encountered during advancement of the probe may represent esophageal compression by a large aneurysm, and if so, consideration should be given to abandon the examination. Finally, in aortic dissection, because the adventitia is the sole layer of the wall of the false lumen, aortic rupture may occur if the TEE probe is not manipulated cautiously.
As with any TEE examination, a systematic approach is required to thoroughly evaluate the thoracic aorta. As per the SCA/ASE guidelines, there are six short-axis and two long-axis imaging planes that enable imaging of most of the thoracic aorta.7 Although many sequences are possible, the authors recommend the following order: Begin with the midesophageal (ME) aortic valve (AV) short-axis (SAX), “Mercedes-Benz” view, which is obtained at the midesophageal level with the scan angle rotated forward to 30° to 60° (see Figure 5–19B). From here, the angle can be rotated by another 90° to about 120° to 150° to identify the ME AV long-axis (LAX) view (see Figure 5–20B). The long-axis view is particularly important because it allows evaluation of the aortic valve and proximal ascending aorta. Measurements can be made of the left ventricular outflow tract (LVOT), aortic valve annulus, sinuses of Valsalva, STJ, and ascending aorta if aortic valve repair and/or root reconstruction are planned (Figure 16–2). In order to visualize the ascending aorta in short axis, rotate back to a scan angle of 0° and slowly withdraw from the level of the aortic valve (ie, ME ascending aorta SAX view; see Figure 5–30B). By rotating forward to a 120° scan angle, a ME ascending aorta LAX view is obtained (see Figure 5–32B). It is crucial in these two views to carefully examine the aorta for dissections. Artifacts are frequently encountered within the ascending aorta, and it is important to distinguish artifacts from true pathology as discussed in Chapter 3.
Following examination of the ascending aorta, the TEE probe should be advanced to the level of the ME four-chamber view and rotated towards the patient’s left. This should result in the descending aorta SAX view in which the aorta appears as a circular image at the top of the screen (Figure 16–3). As the descending aorta is about 3 to 4 cm in diameter at this level, reducing the scan depth to 6 to 8 cm and selecting a high transducer frequency improves both the spatial and temporal resolutions of the image. Almost the entire descending thoracic aorta may be visualized in short axis by advancing and withdrawing the TEE probe. By rotating the scan angle forward to 90°, the descending aorta can be seen longitudinally (see Figure 16–3). Alternating between short- and long-axis views may help demonstrate aortic pathology more comprehensively. While withdrawing the TEE probe and maintaining the descending aorta in the short-axis view (at 0°), the aorta will change in appearance from circular to longitudinal at the level of the aortic arch (upper esophageal [UE] aortic arch LAX; see Figure 5–34). Frequently, the origins of the left subclavian and carotid arteries can be seen. Adding CFD with the Nyquist limit set at 50 cm/s may aid in visualizing these vessels. Finally, by rotating forward to 90°, the UE aortic arch SAX will be obtained (see Figure 5–31B). Most aortic pathologies can be identified by adding pulsed-wave Doppler (PWD) and continuous-wave Doppler (CWD), as well as gray-scale and color M-mode to the two-dimensional (2D) examination above.
An aortic aneurysm is a localized or diffuse dilation of the aorta to twice its diameter involving all three layers of the vessel wall. The estimated annual incidence is six cases per 100,000 persons.8 TEE is useful for the diagnosis and classification of thoracic and upper abdominal aortic aneurysms. Thoracoadominal aneurysms (TAAs) are categorized into four types based on the Crawford classification system (Figure 16–4).9 Type I involves the entire descending thoracic aorta to the abdominal aorta above the renal arteries. Type II originates in the proximal descending thoracic aorta and terminates distal to the renal arteries. Type III affects the distal half of the thoracic aorta and the abdominal aorta to the bifurcation. Type IV is limited to the distal portion of the descending thoracic aorta and the abdominal aorta to the bifurcation.
Aneurysms are generally thought to be a disease of aging and a consequence of degeneration and atherosclerosis. Aging results in a pathological process that involves the development of eccentric fibrous intimal thickening, lipid deposition, and calcification, leading to weakening of the aortic wall and dilation.10 According to Laplace law (Tension = Pressure × Radius), as the diameter of the lumen increases, the wall tension increases resulting in progressive dilation. Other causes of TAAs include connective tissue diseases (ie, Marfan’s, type IV Ehlers-Danlos and Loeys-Dietz syndromes), infections (ie, bacteria, mycotic, or syphilitic), trauma, and increased wall tension secondary to hypertension or a high-velocity jet originating from aortic stenosis.
The decision to surgically repair a TAA is based upon the size and etiology of the aneurysm. According to recommendations by the Society of Thoracic Surgeons, a thoracic fusiform aneurysm should be surgically repaired if it is greater than 5.5 cm in diameter or twice the diameter of the normal contiguous aorta.11 Indications for saccular aneurysm have not been determined, but it is considered reasonable to intervene if the width is greater than 2 cm. Patients with connective tissue diseases, such as Marfan’s syndrome, may be considered for early operative repair because of their increased risk of dissection or rupture. A strong family history of aortic aneuryms may also prompt early intervention. Finally, symptomatic patients should be considered for operative treatment regardless of the size of the aneurysm. Symptoms include persistent pain, malperfusion, and compression of nearby structures leading to dysphagia, cough, hoarseness, or Horner’s syndrome. Descending TAAs can also be treated by endovascular stent grafting. There are no established guidelines regarding which patients should be managed with endovascular aortic repair (EVAR). In general, patients at high risk for complications from either conventional open repair or medical management may benefit from this relatively less invasive approach. Another emerging alternative for complex aortic pathology is the “hybrid” approach in which an open surgical technique is combined with an EVAR. This approach is thought to maximize the benefit of complete repair of complex lesions while minimizing the risk of a total open technique.
TEE may be used to detect the patency of aortic side branches and to evaluate for the presence of organ malperfusion. In the thoracic region, the identification of the left subclavian artery and its patency may be particularly important in EVAR and hybrid approaches. Intraoperative TEE is also an excellent monitoring tool, especially if aortic cross-clamping is performed, and may be helpful during cannulation if total or partial extracorporeal circulatory support is required. Monitoring of cardiac function is an added benefit of TEE during aortic aneurysm surgery. While the aorta remains the focus of intraoperative imaging, the effects of aortic manipulation on cardiac function can also be evaluated. This enables clinicians to make informed decisions on pharmacological support, should it be required.
An aortic dissection is a separation in the aortic wall that allows blood flow within the tunica media. Currently, there are two proposed etiologies for aortic dissections.12 In the first hypothesis, the intima is ruptured along the edge of an atheromatous plaque or at a penetrating ulcer. The high pressure in the aorta forces blood through the intimal tear into the tunica media, creating a false lumen. The intimal layer that separates the false lumen from the true lumen (normal conduit of blood in the aorta) is termed the intimal flap. While intimal injury per se does not lead to dissection, it is a common precipitating factor, especially when the aortic medial layer is diseased. In the second hypothesis, the dissection is attributed to spontaneous rupture of the vasa vasorum or degeneration of the collagen and elastin that make up the tunica media. This medial layer can be affected by poor structural integrity as seen in old age or with primary connective tissue diseases such as Marfan’s syndrome. Apart from medial integrity, the time required for extension of an intimal tear and development of a dissection depends on the rate of rise of systolic pressure, pulsatile pressure, diastolic recoil, and mean arterial pressure.
Aortic dissection is the most common cause of death among all conditions involving the aorta. The incidence of thoracic aortic dissection in North America is about 5 to 10 cases per million people per year.13 The mortality associated with acute aortic dissection is extremely high, with 21% of patients dying before hospital admission.14 The mortality rate from acute aortic dissection has been shown to be 1% to 3% per hour for the first 24 to 48 hours, and as high as 80% by 2 weeks.15 Due to this high mortality, early diagnosis is considered crucial for appropriate management to be initiated.
Magnetic resonance imaging (MRI) is currently the gold standard test for the detection and assessment of aortic dissections with a sensitivity and specificity of 98% and 98%, respectively.16 However, there are many contraindications to MRI examination including implanted medical devices (ie, pacemaker, orthopedic hardware, etc) and hemodynamic instability. Consequently, TEE is increasingly becoming an important and convenient modality for diagnosis of acute aortic dissection. TEE, similar to MRI, is highly sensitive and specific for the diagnosis of aortic dissection, with a sensitivity of 97% and specificity of 100%.17 TEE is an attractive first-choice diagnostic procedure because of its accuracy, speed, relatively low cost, portability, and noninvasiveness.18 However, a major limitation of TEE in the diagnosis of aortic dissection is the inability to reliably visualize the distal ascending aorta and proximal aortic arch. The frequent presence of artifacts such as mirror images in aortic imaging makes TEE prone to important false-positive diagnoses of dissection (Figure 16–5).
Figure 16-5.

Midesophageal ascending aortic long-axis view with a suspicious shadow (?) in the aortic lumen. The pulmonary artery (PA) catheter may cast a mirror image artifact in the ascending aorta that displays a similar “bounce” to that of an intimal flap, creating an impression of a dissection. Similarly, an actual dissection flap may be erroneously mistaken for an artifact.
There are two main classification systems utilized for thoracic aortic dissections (see Figure 16–4).19 The DeBakey classification system recognizes three types of aortic dissections.19,20 In type I, the entire aorta is dissected; in type II, only the ascending aorta is involved; and in type III, the ascending aorta and arch are spared, while the descending aorta is dissected. Type III is further subclassified into type IIIA, involving the descending thoracic aorta alone, and type IIIB, extending into the abdominal aorta. The Stanford system classifies dissections into two types.20 In Type A the ascending aorta is affected, while in Type B the ascending aorta is spared. A classification system from Europe has also been proposed to replace the DeBakey and Stanford classification systems.21,22 This classification groups dissection into five types based on etiology (Table 16–1).
Class | Description |
---|---|
I | Classic aortic dissection (DeBakey and Stanford) |
II | Intramural hematoma/hemorrhage |
III | Discrete/subtle dissection without hematoma |
IV | Plaque rupture leading to aortic ulceration |
V | Traumatic or iatrogenic |
These classification systems have important prognostic and therapeutic consequences.11,23 Type A aortic dissection is a formal indication for surgical intervention because the reported mortality rate with medical therapy far exceeds that reported for surgical treatment.24–26 Unlike Type A aortic dissections, the correct management for Type B aortic dissections remains controversial.27–29 Medical management is advocated for most Type B dissections as most studies show no clear survival advantage with surgical management. Some indications for surgery in Type B dissection include organ malperfusion, persistent pain, hemodynamic instability, or any signs of impending or ongoing rupture, notably the accumulation of pleural, pericardial, periaortic, or mediastinal fluid; propagation of the dissection; increasing size of hematoma; and development of a saccular aneurysm. In addition, echocardiographic evidence of a wide-open false lumen with communication to the true lumen increases the risk of progression of the dissection, and therefore is considered an indication for surgery.
Though an intimal tear is the classic finding for aortic dissection, it is not always present. The presence of an intimal flap is therefore considered a classical sign of dissection, but not a mandatory one. The TEE examination of a patient with aortic dissection involves several components including characterization of the dissection, assessment of flow in aortic branches, and determination of cardiac complications. The dissection flap is a thin, mobile echogenic membrane found within the aortic lumen; however, to avoid a false-positive diagnosis, the intimal flap must be identified in multiple image planes.18,30,31 Although identification of the site of the intimal tear can be challenging, CFD imaging is useful in the assessment of entry and exit sites. It can sometimes be very difficult to distinguish the true lumen from the false lumen. In contrast to the false lumen, the true lumen tends to be smaller, round in appearance, shows enlargement during systole, and often has normal PWD and CFD profiles. In addition, M-mode imaging can help determine the direction of movement of the flap in systole, and thereby identify the location of the true lumen (Figure 16–6). The false lumen is usually larger and crescent shaped, and often demonstrates spontaneous echo contrast suggesting sluggish blood flow.
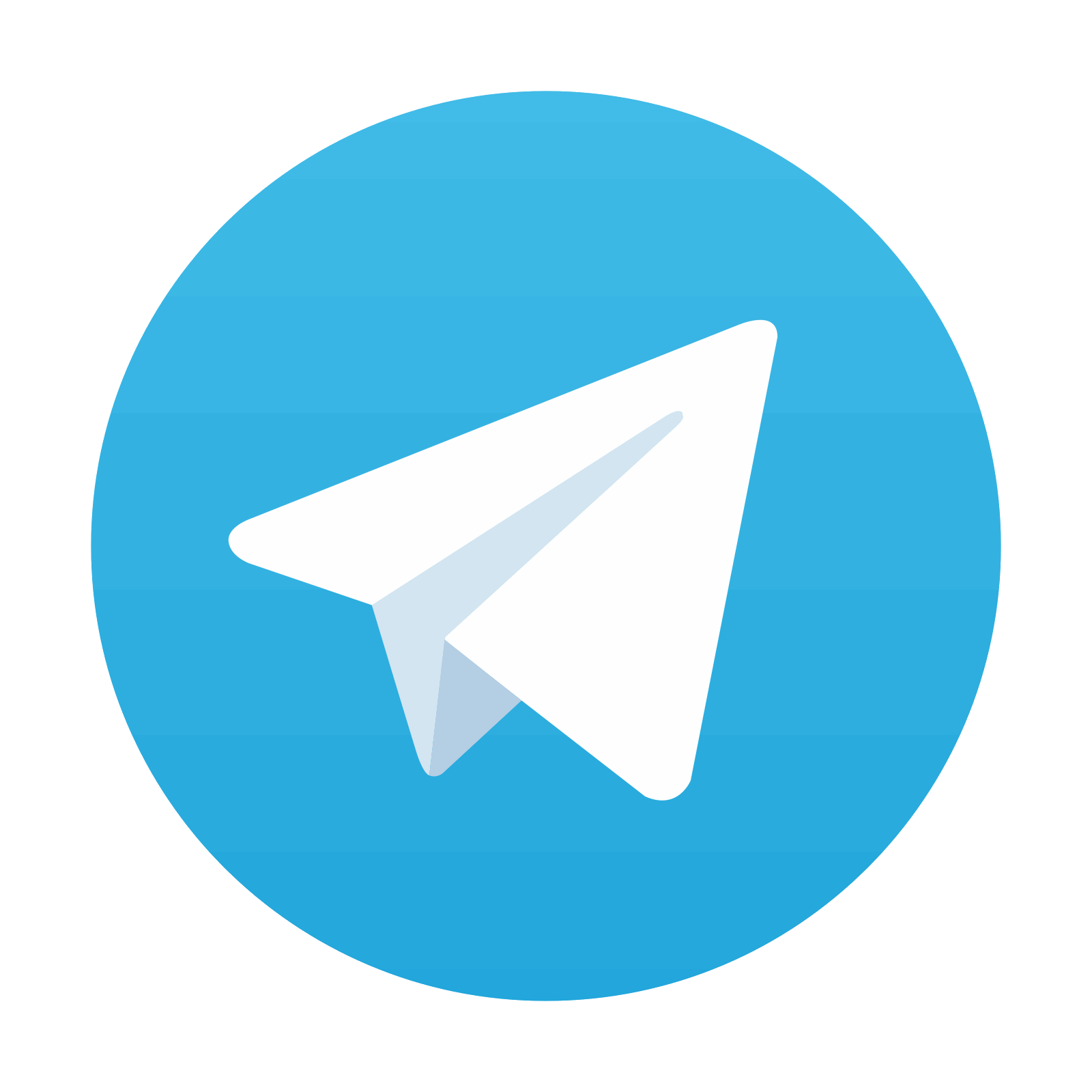
Stay updated, free articles. Join our Telegram channel

Full access? Get Clinical Tree
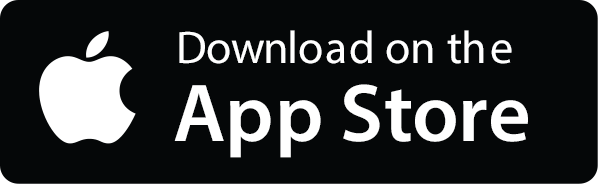
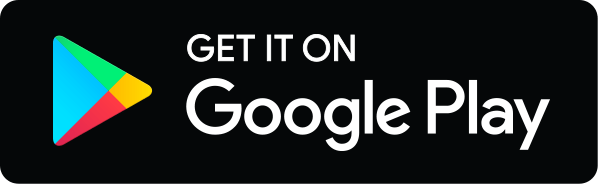