Tectonic plates move in relation to one another in three specific patterns described as strike-slip, dip-slip, and oblique-slip (Figure 38.2). Strike-slip faults occur when plates slide horizontally past one another. Dip-slip faults occur when the plates slide over or under each other.6 These are further characterized as normal faults (where the underlying segment moves upward) and reverse faults (where the underlying segment moves downward). Oblique-slip faults exhibit both types of motion when they rupture. Dip-slip faults are most frequently associated with the development of tsunamis.

Types of Fault Motion Characterizing Strike-Slip and Dip-Slip Faults.
The location where the fault rupture begins is known as the hypocenter (or focus) and is located below the earth’s surface. The point on the earth’s surface directly above the hypocenter is called the epicenter (Figure 38.3). When an earthquake occurs, stress is relieved along the fault lines as the land masses shift and release energy in the form of seismic waves. Temblors produce three types of seismic waves: primary (P), secondary/shear (S), and surface (L) waves (Figure 38.4). P and S waves are referred to as body waves, meaning that they develop at the hypocenter and radiate in all directions through the earth’s interior. L waves are surface waves and can only move through the earth’s crust.

Relationship between Hypocenter (Focus) and Epicenter. Modified from http://teampride.yolasite.com/resources/8.1.pdf.

Earthquake Shock Waves. Modified from http://home.hiroshima-u.ac.jp/er/Resources/Image195.gif.
P waves are the fastest of the seismic waves, traveling at 4.8 km/s in a longitudinal direction. S waves travel at 3.2 km/s and cause the earth to move at right angles from the direction of the P waves. The difference in velocity between the P and S waves allows determination of the epicenter. Different rates of travel between the P and S waves also produce two separate perceptions by individuals. The P wave generates an acoustic signal that sounds like an approaching train whereas the S wave produces a sharp jolt. The L wave is a slow surface disturbance that causes swaying of tall buildings and large swells in bodies of water. This seismic wave is the major cause of damage and injury from earthquakes.
The frequency and amplitude of the vibrations produced at the surface and the subsequent earthquake severity depend on the amount of mechanical energy released, the distance/depth of the focus, and the structural properties of the soil near the surface.6,8 Distance from the epicenter is a poorer predictor of severity because the transmission strength of earthquake shock waves is influenced by ground composition, liquefaction, and landslide susceptibility. Soils with high water content transmit energy waves that cause significant mortality, morbidity, and structural damage even at great distances from the epicenter. In contrast, solid rock transmits earthquake energy with a minimum of vibration. This explains why areas in a liquefaction zone situated relatively far from the epicenter can be more severely impacted by seismic intensities than locations nearer the epicenter on bedrock.6
Earthquakes are characterized by intensity and magnitude. Magnitude is the total energy generated by the temblor. This energy is measured with a seismograph and then converted using the Richter scale (Table 38.2). The Richter magnitude scale is a logarithmic scale that estimates total energy released by an earthquake. A change of 1 unit in the Richter scale corresponds to a 10-fold change in ground motion and a 32-fold change in radiated energy.8 Measurements on the Richter scale below 2.0 are not usually felt and measurements on the Richter scale above 5.0 can cause damage. A major earthquake consists of a magnitude 7.0 or greater. A major earthquake may be preceded by preliminary tremors known as foreshocks. There can also be lower magnitude events after the main earthquake known as aftershocks, which can produce significant additional damage and may necessitate additional local or regional evacuations.
Richter Scale
Description | Magnitude | Number per year | Approximate energy (J) |
---|---|---|---|
Great Earthquake | >8.0 | 1–2 | >6.3 × 1016 |
Major Earthquake | 7.0–7.9 | 15 | >2 × 1015–6.3 × 1016 |
Destructive Earthquake | 6.0–6.9 | 134 | >6.3 × 1013–2 × 1015 |
Damaging Earthquake | 5.0–5.9 | 1319 | >2 × 1012–6.3 × 1013 |
Minor Earthquake | 4.0–4.9 | 13,000 (estimated) | >6.3 × 1010–2 × 1012 |
Smallest Usually Felt | 3.0–3.9 | 130,000 (estimated) | >2 × 109–6.3 × 1010 |
Detected but Not Felt | 2.0–2.9 | 1,300,000 (estimated) | 6.3 × 107–2 × 109 |
The earthquake’s intensity is determined by the degree of ground shaking in a particular location. Intensity is calculated using two separate methods: an objective approach using instrumentation and a subjective evaluation based on human observations and perceptions. The first method uses motion detectors to record peak ground velocity (PGV) and peak ground acceleration (PGA). The greater the degree of ground motion, the higher the recorded velocity and acceleration. This information is referred to as the instrumental intensity. PGV and PGA determinations are based on the degree of ground movement measured at localized points obtained by ground sensors placed in earthquake-prone areas. When available, they provide precise estimates of intensity not influenced by the subjective perception of motion or damaged structures. Instrumental intensities recorded during an earthquake are better predictors of injury and lethal outcomes than the rate of building collapse.9,10,11 However, since sensors are placed in only a few locations, the use of instrumental intensities as a worldwide measure is limited.
The second method of measuring intensity, the Modified Mercalli Intensity (MMI) scale, relies on the extent of observed property damage and the perceived degree of shaking reported by people experiencing the earthquake.12 Intensity determinations are made using this subjective 12-point scale after an earthquake when U.S. Postal Service employees are interviewed regarding their experiences and the visible structural damage they observe (Table 38.3). Different governmental workers may perform this task in other countries. Using one of the twelve categories on the MMI scale, a value is selected that most accurately represents the degree of shaking and damage. This value is then assigned to the pertinent postal code. Recording the MMI values for all postal codes in the earthquake zone yields a representation of the overall intensity. Although MMI scale measurements are subjective, they are generally valid. Researchers have found that MMI values correlate well with structural damage, deaths, and traumatic injuries.6,10 Electronic resources and social media have increased the potential for data collection in MMI scales. The United States Geological Survey now invites individuals to report their experience of temblors via an online adapted MMI questionnaire (http://earthquake.usgs.gov/earthquakes/dyfi) that contributes to shake maps for seismic events around the globe. Comparisons between the MMI scale, Richter scale, and Instrumental Intensity scale are depicted in Table 38.4.
The Modified Mercalli Intensity Scale
INTENSITY | I | II–III | IV | V | VI | VII | VIII | IX | X+ |
---|---|---|---|---|---|---|---|---|---|
SHAKING | not felt | weak | light | moderate | strong | very strong | severe | violent | extreme |
DAMAGE | none | none | none | very light | light | moderate | moderate/heavy | heavy | very heavy |
Comparison between the Modified Mercalli Scale, the Richter Scale, and the Instrumental Intensity Scale
Modified Mercalli Scale | Richter Scale | Instrumental Intensity Scale | ||||||
---|---|---|---|---|---|---|---|---|
Perceived Shaking | Potential Damage | Peak Acceleration (%g) | Peak Velocity (cm/s) | Instrumental Intensity | ||||
I | Felt by almost no one | Generally not felt, but recorded on seismometers | Not felt | None | <0.17 | <0.1 | I | |
II | Felt by very few people | 2.5 | ||||||
III | Tremor noticed by many, but they often do not realize it is an earthquake | Weak | None | 0.17–1.4 | 0.1–1.1 | II–III | ||
IV | Felt indoors by many; feels like a truck has struck the building | 3.5 | Felt by many people | Light | None | 1.4–3.9 | 1.1–3.4 | IV |
V | Felt by nearly everyone; many people awakened; swaying trees and poles may be observed | Moderate | Very light | 3.9–9.2 | 3.4–8.1 | V | ||
VI | Felt by all; many people run outdoors; furniture moved, slight damage occurs | Strong | Light | 9.2–18 | 8.1–16 | VI | ||
VII | Everyone runs outdoors; poorly built structures considerably damaged; slight damage elsewhere | 4.5 | Some local damage may occur | Very strong | Moderate | 18–34 | 16–34 | VII |
VIII | Specially designed structures damaged slightly, others collapse | Severe | Moderate/Heavy | 34–65 | 31–60 | VIII | ||
IX | All buildings considerably damaged, many shift off foundations; noticeable cracks in ground | 6.0 | A destructive earthquake | Violent | Heavy | 65–124 | 60–116 | IX |
X | Many structures destroyed; ground is badly cracked | 7.0 | A major earthquake | |||||
XI | Almost all structures fall; very wide cracks in ground | Extreme | Very heavy | >124 | >116 | X+ | ||
XII | Total destruction; waves seen on ground surfaces, objects are tumbled and tossed | 8.0+ | Great earthquake |
Seismology, the study of earthquakes and the propagation of seismic waves, has made progress in estimating the probability of a strong earthquake occurring during any 24-hour period in certain parts of the United States, such as California. These predictions are based on evaluations of previous events and analysis of potential relationships between events. Seismologists cannot currently predict with certainty when or where the next earthquake will occur or its intensity level.13
Management Issues
The persistent threat of seismic events and the difficulties involved with mitigating their effects highlight the importance of disaster preparedness for such events. Planning efforts should emphasize the following: 1) protocols outlining predicted modifications of prehospital and hospital medical care during the initial response; 2) efficient use of community resources; 3) awareness of common clinical conditions seen after earthquakes and related treatment options; and 4) recognition that arrival of outside help will not be immediate and likely require more than 48 hours.
Incident Command
In the initial period after an earthquake, a degree of uncertainty arises for individuals in a community and for the local and regional healthcare system. Once it is clear that resource demands in the post-earthquake period exceed what is available under standard operating procedures, establishing a system of command and control, known as an Incident Command System (ICS), becomes essential for effective management. Based on a concept initially used by California firefighters in the 1970s for effective coordination and resource control when battling wildfires, the ICS directs response activities through decisions made by a single individual known as the incident commander and implemented by a formal chain of command. By providing a standardized system of command and control, complex situations can be managed more efficiently during a disaster. In cases in which multiple jurisdictions have a role in management, the ICS becomes a Unified Command System and may include representatives from state and federal levels in addition to local entities.7,14 A detailed explanation of ICS can be found in Chapter 11.
An incident management system is used at the Emergency Operations Center (EOC) for each responding organization. This center is the location where personnel representing various entities from the public and private sector meet during an emergency event to: 1) coordinate response and recovery actions; 2) conduct strategic decision-making; and 3) manage resource allocation.7,14
Prehospital Response
After an earthquake, victims’ lives may depend on how rapidly they are extricated from collapsed buildings and how expeditiously they receive medical treatment. In urban areas of developed countries, it is common that paramedics perform initial prehospital care; however, in a large-scale earthquake they may be unavailable for this activity, particularly in systems in which they have primary responsibilities as firefighters (since fires are common after earthquakes). In systems not using paramedics, first responders may be police, firefighters, other ambulance personnel, or even physicians.
Complicating the threat to the population, secondary events that increase the lethality of the initial earthquake can occur after seismic activity. Such events include fires, landslides, floods, and tsunamis, among others.2,6 The 2004 Indian Ocean (Sumatra-Adanam) Earthquake and Tsunami was one of the most destructive seismic events of the past century. The vast majority of deaths were the result of tsunami activity, rather than the initial earthquake, whose epicenter was over deep water off the coast of Sumatra. During the 1994 Northridge, California, earthquake, fires and burns accounted for 6.1% of the fatalities and 7.3% of the hospitalized injuries respectively, even though nonresidential buildings were the most affected and the fires were quickly controlled.2
When fires occur immediately after an earthquake, such as in the 1989 Loma Prieta temblor in northern California and the 1995 Great Hanshin earthquake in Japan, the priority is to minimize the amount of damage resulting from these blazes. Much the same as medical emergencies, fires may quickly outstrip the resources available for conflagration control. Therefore human resources in places where firefighters also share responsibility for medical response or search and rescue will be initially directed to fire suppression.7,15 This can leave the affected area without paramedic support. Although it was only a moderate-sized earthquake, sections of San Francisco went without paramedic support in the critical early hours following the Loma Prieta temblor because of the prioritization of fire suppression over other aid efforts.
Another problem faced by industrialized populations after an earthquake is exposure to released toxic materials stored in such facilities as power stations and chemical plants. Following the 1989 Loma Prieta earthquake, approximately 20% of the post-earthquake injuries were caused by exposure to toxic chemicals.12 The 2011 Tōhoku Earthquake and Tsunami resulted in the second worst nuclear accident in history to that date. Under these circumstances, decontamination and evacuation must be addressed to limit the number of exposed victims, and to improve community safety. When managing the threat of toxic exposures, it is reasonable to consider directing human resources to assist with decontamination and evacuation instead of the rescue effort.
Communication between paramedics in the field, base stations providing medical direction for prehospital personnel, and receiving hospitals can be disrupted after a seismic event. Notifying hospitals of victims’ impending arrivals becomes difficult, increasing the risk that healthcare resources cannot be effectively managed. Many individuals will not wait for the arrival of paramedics or other prehospital providers. Family and friends will frequently bring victims to the nearest, most familiar, or most accessible hospital, overwhelming the facility’s capacity. During the Puerto Limon, Costa Rica, earthquake in 1999 most victims were transported by survivors.16 Following the 2001 Gujarat, India, earthquake, most of the victims used private transportation to reach hospitals.17 Major trauma victims will be brought to non-trauma centers and many patients without life-threatening injuries will arrive at trauma centers. This mismatch of medical needs and available resources can potentially overwhelm capacities and result in inefficient utilization, potentially leading to increased morbidity and mortality. While this phenomenon is undesirable, it is difficult to avoid during the first hours after an earthquake.
Breakdown of communication systems makes coordination of field care and disposition difficult, jeopardizing the direction and integration of all prehospital activities. Prehospital provider radios frequently rely on repeaters that can fail, making transmission of radio signals to dispatchers or base station hospitals difficult. Ambulances from different jurisdictions tend to use different radio frequencies, making communication between field units and the central coordinating body problematic even when repeaters function. Complicating the situation is the arrival of unsolicited ambulances from outside regions to areas most impacted by the earthquake. Without functioning communications, individuals responsible for coordinating field activity may remain unaware when this outside assistance arrives. Satellite or cellular (wireless) telephones are also of limited usefulness as circuits quickly become overloaded with a large increase in official and non-official communication. The creation of a globally recognized disaster frequency, or even of nationally recognized disaster frequencies, would make a significant contribution to the improvement of communication under these circumstances.
Many alternate communication systems for response coordination and patient care have been developed to address the challenges arising after disaster events, especially in Europe and in the United States. A wireless transmission system for disaster patient care (WISTA) was developed to assist emergency personnel in treating disaster victims and coordinating medical resources.18 The Wireless Internet Information System for Medical Response in Disasters (WIISARD) is a project by the University of California, San Diego, designed to support disaster relief operations in the field.18 The Trauma Patient Tracking System is another initiative that allows integration of information and patient tracking over a wireless network from initial field triage or hospital presentation throughout the disaster event and follow-up. Information is linked to the individual via a barcoded wristband containing a Global Positioning System (GPS) or Radio Frequency Identification (RFID) tracking device.19 Although these systems show promise, at the time of this writing none has been implemented after an earthquake. Given the additional likelihood of electricity and other infrastructural failures, concern exists that technology-based systems will not be applicable during the initial response period.
Movement into and out of a disaster zone is difficult following an earthquake. Significant damage occurs to transportation infrastructure such as roads, bridges, traffic signals, and road lighting. Following the 2010 Chilean earthquake, nearly 300 roadway bridges in the country were destroyed or damaged, including 20 with collapsed spans.20 During the 1994 Northridge earthquake, 15% of the fatal injuries were motor vehicle related, primarily as a result of the disruptions in traffic control devices.10 Forty-three of the sixty-three total deaths following the Loma Prieta event were associated with the collapse of road structures.10 Individuals involved in earthquake-related motor vehicle crashes are 5.23 times more likely to die at the scene than survive to hospitalization.2 Landslides, soil settlement, and slope failures, among other events, increase damage to roadways. Ill or injured survivors who require transportation to medical centers will experience delays until safe or passable routes are identified. The early use of law enforcement personnel (including National Guard assets in the United States) to control important transportation areas can improve this situation.7
The initial prehospital response should be directed toward the provision of emergency medical assistance, followed by search and rescue activities. Approximately 90% of all earthquake deaths are the result of structural collapse.6 In some studies, death and injury rates were 67- and 11-fold higher, respectively, for trapped victims compared to those who did not require extrication.12,21 Injuries caused by collapsing structures or falling building components were 8.36 times more likely to result in fatalities than in hospitalizations.2 The consequences of constructing buildings from substandard material and using seismically unsafe designs are clear.
There is a well-documented decrease in survival for victims trapped longer than 24–48 hours after an earthquake, as seen in the Campania-Irpinia earthquake (1980) in Italy and the Tangshan earthquake (1976) in China.3,8 In Italy, a survey of 3,169 survivors showed that 93% of those who were trapped and survived were extricated within the first 24 hours and 95% of those who died expired before extrication.21 Estimates of survivability among entrapped victims in Turkey and China indicate that within 2–6 hours, fewer than 50% of those buried were still alive.3,11,21 In studies of the 1980 Italian and 1988 Armenian earthquakes, investigators concluded that 25–50% of the victims who were injured and died slowly could have been saved if they had received initial life-saving treatment within 6 hours of the event.3,11,12,21
Urban Search and Rescue (USAR) teams are among the first types of assistance deployed to earthquake zones. These teams theoretically increase survival by facilitating prompt extrication of trapped victims. In principle, USAR teams can help local leaders with structural assessment, advanced search and rescue techniques, and specialized medical training to limit the period of entrapment and mitigate some of its adverse medical effects.22 The utility of USAR teams has, however, been questioned. In the United States, for example, it typically requires nearly 24 hours for teams to deploy and begin search and rescue activities in country and even more time to provide international assistance, thereby limiting their usefulness for saving lives after an earthquake.22 In the Northridge earthquake, 19 hours passed before a USAR team originating in a city only 160 km (100 miles) from the affected area began operations at the collapsed Northridge Meadows Apartments.7 After the 2011 Tōhoku Earthquake and Tsunami, a major Japanese USAR team had to be called back from its deployment in New Zealand to perform rescue activities in Japan.23 The first U.S. USAR team sent to Turkey after the 1999 earthquake required 48 hours to begin operations.7 After the 2010 earthquake in Haiti, airport damage and other access problems delayed arrival of some USAR teams by more than 4 days.24 Reports show that more than 90% of survivors are rescued by local first responders and civilian volunteers within the first 24 hours after an earthquake event.21,24
USAR teams represent an expensive component of the earthquake response, with a heavy equipment team costing an estimated $900,000 USD to deploy for 10 to 14 days (average length of assignment).24 In addition, the consumption of severely limited resources in the earthquake zone by USAR teams to support the transportation and housing of their equipment and personnel may prevent or delay the deployment of other critically necessary medical aid services to the same area. Increasingly there are questions as to the appropriateness of large funding commitments and diversion of other resources to support USAR teams. Some have suggested that funding mobile field hospitals and other interventions might be more effective.24 One option is to train local inhabitants and first responders such as law enforcement personnel in basic search and rescue techniques. This would provide survivors who have the desire to assist their families, friends, and neighbors by engaging in search and rescue activity with the appropriate tools and techniques to do so. Reports on previous search and rescue events have noted that civilian participation in these efforts, specifically in providing knowledge of victims’ whereabouts to searchers, may greatly enhance opportunities for timely extrication and survival.25
After an earthquake, an increased requirement for surgical services is expected during the first 72 hours.6 Early rapid assessment of the extent of damage and injuries is necessary to direct resources where they are most needed.10 Rescue workers frequently perform triage, using a system similar to Simple Triage and Rapid Treatment (START). This methodology can theoretically sort patients into groups of increasing acuity; however, controversy exists regarding the efficacy of such systems and data supporting their use are limited. Two studies evaluating START suggest it may be useful.26,27 A third study evaluating meaningful performance metrics for START in an actual disaster concluded the triage algorithm had an acceptable level of undertriage and was successful in prioritizing transport of the sickest patients to area hospitals.28 The START system emphasizes basic lifesaving measures such as opening an airway and applying direct pressure for external bleeding control, but rescuers are trained not to provide definitive care procedures at the scene. Chapter 14 contains additional information about the START system.
Hospital and Community Response
Hospitals are the traditional source of medical care after seismic events. However, hospitals can also be impacted by earthquakes, aggravating the initial imbalance between demand for care and response capacity. Healthcare facility closure and evacuation are mandated if management staff identify environmental or structural factors that put patients’ safety at risk. An early structural assessment performed by expert engineers or, if not available, by on-site personnel is critical. Institutional disaster plans must include a hospital functional status evaluation.29 The Applied Technology Council (ATC) provides guidance for structural assessments through documents such as the ATC-20 and ATC-20–2, and via a downloadable smartphone or tablet application ROVER (Rapid Observation of Vulnerability and Estimation of Risk, downloadable at www.atc-rover.org) which were developed with support of the U.S. Federal Emergency Management Agency (FEMA) and other entities.3 Structure-dependent collapse patterns are documented in the FEMA Structural Engineer Training Manual.22
After the 2005 Pakistan earthquake, 65% of hospitals in the affected area were destroyed or badly damaged.30 All hospitals in the Port au Prince region of Haiti were severely damaged or destroyed in the 2010 earthquake, possibly related to the lack of building codes in the country. Even facilities in areas with strict earthquake building codes may prove vulnerable to earthquake damage and require evacuation. Following the 2011 Tōhoku Earthquake and Tsunami, nearly 80% of hospitals and 30% of clinics in the affected regions were damaged, with many fully destroyed by the tsunami.31,32 During the Northridge, California, earthquake, eight (9%) of ninety-one acute care hospitals were evacuated. Six of these institutions evacuated patients within the first 24 hours after the temblor, including several constructed under the most updated building codes. Two additional hospitals whose initial inspections revealed no critical damage and therefore continued providing patient care (including elective surgeries) were subsequently evacuated in the following weeks and were eventually demolished.33 Thus hospitals reported to be secure may later prove vulnerable.
Hospitals that cannot continue providing safe inpatient care need to implement policies and procedures to facilitate the transfer and evacuation of their patients. Additionally, hospitals with patients requiring long-term care may choose to evacuate these patients to more distant facilities or to those without emergency or trauma services, making those beds available for potential disaster victims. Ideally, evacuation should be coordinated by the regional EOC if one exists to ensure effective resource utilization; however, if time is critical, hospitals can successfully evacuate patients independently without assistance from an EOC. Such activity is facilitated if mutual aid agreements with other hospitals are already in place or if the hospital is part of a larger system, such as the Department of Veterans Affairs in the United States. In the 2011 Christchurch, New Zealand, earthquake, a national teleconference was held to coordinate transfer of intensive care unit (ICU) patients from the only hospital with an emergency department in the city to hospitals in other areas of the country.34 Evacuation transport problems can often be solved by collaboration between military and civilian groups. In the 1999 Marmara earthquake in Turkey, military boats and helicopters were used to transfer patients to remote major cities.29
Determining which patients to evacuate first is not always straightforward. Individuals hospitalized in ICUs require extensive resources, which are limited after a seismic event. Evacuating the sickest patients first from hospitals often works best, lessening the burden on healthcare facilities while improving the chances for better care of remaining patients and arriving victims.7,32 When time is critical and structural collapse is imminent, evacuating the healthiest patients first permits movement of the greatest number of patients in the least amount of time.7 During the Northridge earthquake, one evacuating institution believed that patients were in immediate danger and chose to evacuate the healthiest patients first, successfully evacuating all patients (334 total) to nearby open areas in 2 hours.32 Methods to evacuate patients from a hospital may vary. In the Northridge evacuation, supervisors transported patients using available equipment such as backboards, wheelchairs, and blankets.32 Vertical evacuation of patients (moving patients from one floor of the hospital to another) should use stairwells and not involve elevators until these have been inspected and deemed safe.
Without a thorough understanding of the science, planners might falsely believe that hospitals located near the earthquake epicenter will have a higher probability of structural damage. As such, incident managers may incorrectly assume that hospitals located farther away will remain functional and will preferentially direct patients to these facilities. Although this is true for institutions located at large distances from the epicenter, it does not appear these assumptions are correct for facilities located close to the epicenter. A study examining the association between distance from the epicenter and hospital evacuation found no relationship between these two variables (Figure 38.5).35 All facilities studied were located within approximately 32 km (20 miles) of the epicenter. In contrast, a strong association existed between PGA and the need for hospital evacuation, regardless of the institution’s location. It may be appropriate for incident managers to consult earthquake shake maps before making patient transport decisions during hospital evacuations. In regions with ground motion sensors, computers can generate shake maps identifying the areas of greatest shaking within minutes after a seismic event occurs.

Epicenter and hospital locations, Los Angeles County, CA. This map shows the geographic locations of the study and control hospitals, as well as the epicenter of the 1994 Northridge earthquake. From Schultz CH, Koenig KL, Lewis RJ. Decisionmaking in hospital earthquake evacuation: does distance from the epicenter matter? Ann Emerg Med; 50(3) 2007: 320–326.
Information about the status of surrounding hospitals is valuable. Some will sustain damage and be unable to accept victims from the field. Others will be in the process of evacuation. Remaining functional hospitals can expect an increase in emergency department volume as well as requests to accept patient transfers from damaged institutions. Therefore, it would be extremely useful to establish a hospital communication system that could provide a rapid estimate of status of all regional facilities. Implementation of such a system would permit undamaged hospitals to estimate potential demand for their services in the immediate post-disaster period. Healthcare institutions could then decide whether to cancel elective surgeries, discharge stable patients earlier than planned, or implement other components of their surge plans.34 Several communications systems currently exist but have not always performed optimally after seismic events.
Functioning hospitals must activate their disaster plans to prepare for the influx of victims. Effective command and control of the facility’s response requires implementation of a system that is not dependent on the presence of any specific individual and will function at all time and on all days. A proposed model used by many hospitals is known as the Hospital Incident Command System (HICS) and is based on ICS principles. HICS has been effectively used during previous earthquakes.7 More information on HICS is available in Chapter 22.
After establishing a command post and implementing an incident management system such as HICS, hospital administration should focus on evaluating personnel availability, communications, and resources. Hospital staff, like the general population, use telephones as a major method of communication. Because standard telephones frequently fail, secondary communication methods should be available. Examples of such methods include: alphanumeric pagers, priority phones, fax machines, Internet/email/instant messaging, cellular phones, wireless systems, ham radio systems, portable two-way radios, satellite telephones, and runners. Use of overhead speakers in patient care areas may be the simplest method of achieving broad communication for updates to in-house staff.
Hospital personnel who are not in the facility after a disaster can be difficult to contact. Therefore, implementation of a disaster callback policy is necessary. Such a policy could state that personnel should report to work in a major disaster unless they are notified to stay at home. Disaster planners can expect that most hospital staff will remain at work and not abandon their responsibilities. After the Northridge earthquake, as in other earthquakes, the majority of staff remained on duty. Communication and transportation disruptions were the main barriers for workers who did not immediately report to the hospital.7 Notifying suppliers of an abrupt increase in demand for certain products may be difficult.
Patient tracking and the creation of medical records can be problematic after earthquakes. Many patients will not have access to items that document personal information and identity (e.g., medical insurance cards, state-issued identification cards) or will have a health condition that makes gathering information from them impossible (e.g., altered mental status). Therefore, creating a medical record and tracking a patient’s movement through the healthcare system can be challenging. Potential solutions include documenting descriptive information such as sex, estimated age, height, color of skin, unique skin marking (mole, tattoo, or scar), eye color, and possibly fingerprints in the medical record.6 Using records created with generic fictitious names before an event can also solve registration issues. Many trauma centers with electronic tracking systems maintain a large number of these fictitious registration names for quick assignment to unidentified patients. In settings in which patient volume overwhelms hospitals, documenting patient care may not be possible. After the 1991 Costa Rica earthquake, hundreds of patients received medical care even though documentation of these interactions was absent in many instances.16 No widely accepted solution to the patient-tracking problem has yet been found, although some have suggested using bar codes or RFID devices (see Chapter 28).
In actuality, it is difficult for hospitals to meet the entire demand for medical assistance because they typically operate at a high census with little capacity for accepting additional patients. The solution to providing an effective medical response is optimizing use of all available resources. This requires hospitals to establish plans for maximizing surge capacity after an earthquake, even if hospital structures are damaged or fully occupied.12 Typical plans include use of additional space not traditionally designated for patient care, such as cafeterias, auditoriums, and parking lots. After a temblor in Puerto Limon, Costa Rica (1999), the only treatment facility was declared structurally unsafe. This resulted in patient evacuations and establishment of treatment areas in the parking lot. After the Northridge and Loma Prieta earthquakes in the United States, treatment areas were established outside several hospitals.16 Augmenting surge capacity also requires an increase in treatment supplies. To maintain the flow of medical goods, an agreement requiring suppliers to automatically deliver a set amount of material after a seismic event or other disaster should be established as part of a hospital’s disaster plan.
In situations in which receiving hospitals become nonfunctional or remain intact but are unable to meet increased service demands, community-based solutions to augmenting surge capacity may be used. One such model is the Medical Disaster Response program. The main advantage of the Medical Disaster Response program is that victims receive rapid advanced medical care even if hospitals are damaged or destroyed. This project, developed by emergency physicians in southern California, trains healthcare personnel in the management of medical problems commonly encountered after earthquakes. It utilizes supplies stored in the community before an earthquake occurs, similar to the concept behind the Strategic National Stockpile, but at the local level. Under austere conditions, the Medical Disaster Response model directs the initial management of casualties by specially trained local personnel who access medical supplies at designated sites within the community.3 Using this system, local healthcare providers in or near the disaster zone can respond immediately and deliver advanced patient care. Detailed discussion of the Medical Disaster Response project is available in the literature.3,34,36
Surge capacity is critical for a health system’s disaster preparedness. Deficient capacity limits the ability of healthcare systems to respond to disasters.37 Surge capacity plans require multidisciplinary collaboration to be successful. With regards to the personnel component of surge capacity, Schultz and Stratton proposed the creation of a database to provide rapid emergency credentialing of volunteers. If implemented, this strategy would offer an accurate, inexpensive, efficient, sustainable, U.S.-based, Joint Commission–compliant tool for rapidly expanding healthcare personnel support for hospital-based patient care. This database is created from a list provided by each hospital of personnel and physicians currently credentialed at their facility with unrestricted privileges. It would be shared with all participating institutions and a county healthcare agency. Volunteers listed in the database could obtain hospital privileges at any hospital within the county for the first 72 hours after a disaster event.36 Should the database be shared between counties, such individuals could receive emergency privileges in other jurisdictions as well.
The first influx of patients is expected to arrive 30–60 minutes after the temblor, largely by self-transport. These patients will likely present with minor injuries such as uncomplicated lacerations, contusions, or fractures. No consistent relationship between injuries and demographic factors has been reported in the literature; neither age nor sex is considered a risk factor for injury. Because supplies may be limited in a disaster situation, victims with uncomplicated conditions should be quickly evaluated and directed to an observation area, with treatment postponed until more detailed information regarding the overall demand for medical care is available. Many victims suffering from minor injuries never require hospital care. After the 1989 Loma Prieta earthquake, as many as 60% of those with earthquake-related injuries either treated themselves or received treatment in nonhospital settings.12 When treating uncomplicated lacerations during a disaster, providers should be aware of the risks of infection and missed foreign bodies. To decrease these risks, some experts recommend allowing uncomplicated lacerations to close by second intention or to use delayed primarily closure. There is insufficient evidence to justify any particular treatment recommendation at this time.
The second group of patients to arrive to hospitals is composed of victims with more serious conditions such as crush injuries. In developed nations, these victims are more typically transported via ambulance because they were more difficult to extricate or transport by lay individuals. They may also suffer from other medical conditions, making their initial treatment more challenging. Depending on the scenario, the number and acuity of patients needing acute care at local emergency facilities may overwhelm available resources. Therefore, implementation of a triage process is necessary. Hospital triage decisions must focus on which individuals should be prioritized for medical or surgical treatment. START does not discriminate between those who will consume large amounts of limited resources or whose prognosis will remain poor despite aggressive treatment. To allocate resources more appropriately, a proposed algorithm called the Secondary Assessment of Victim Endpoint (SAVE) was created. The goal of this algorithm is to reduce victim mortality and morbidity by allocating treatment resources only to those who will benefit. This means that not all victims will receive the same degree of care they would under normal circumstances. This treatment approach contradicts the usual medical philosophy of providing potentially unlimited care to each patient.35
The SAVE triage algorithm designed for mass casualty events is driven by estimated patient prognosis. It is based on outcomes data from trauma patients and those with other medical conditions who receive standard treatment. It recommends withholding aggressive medical care from those with a less than 50% chance of survival or from those who will essentially deplete all available resources.35 Implementation of SAVE triage should only occur in situations in which the time for return to standard operations is unknown and rationing of limited resources is necessary. A detailed discussion of SAVE triage is available in the literature.35
The need for tools to rapidly prioritize patient care in disasters has received considerable attention. Ultrasonography holds promise as a technology for evaluating mass casualty victims and has many benefits. It is: 1) noninvasive; 2) portable; 3) easily repeatable; and 4) highly sensitive for intraperitoneal blood and many other conditions. Current indications for ultrasound in the evaluation of trauma patients include detection of pleural and pericardial effusions, increased intracranial pressure, pneumothoraces and hemothoraces, and fractures, and for placement of ultrasound-guided nerve blocks for anesthesia. The practical use of sonography has been studied in disaster settings (e.g., following Hurricane Katrina in the United States in 2005) and in the prehospital setting. During the 1988 Armenian earthquake, physicians with two ultrasound machines were able to triage 400 trauma patients over a period of 48 hours in a setting with limited availability of computed tomography.38 Ultrasound has also been used in Kosovo, Afghanistan, and Iraq by deployed military forces.39 Concerns regarding use of ultrasound in disaster situations relate to its dependence on functioning electricity and on the operator-dependent nature of its sensitivity. However, newer portable ultrasound devices can be battery-powered over the short-term, and increasing availability of bedside ultrasound in emergency departments, as well as training in their use, will decrease the variability in operator performance and interpretation of scans. It is likely that ultrasound will be increasingly implemented in disaster settings for patient screening and guiding medical care.
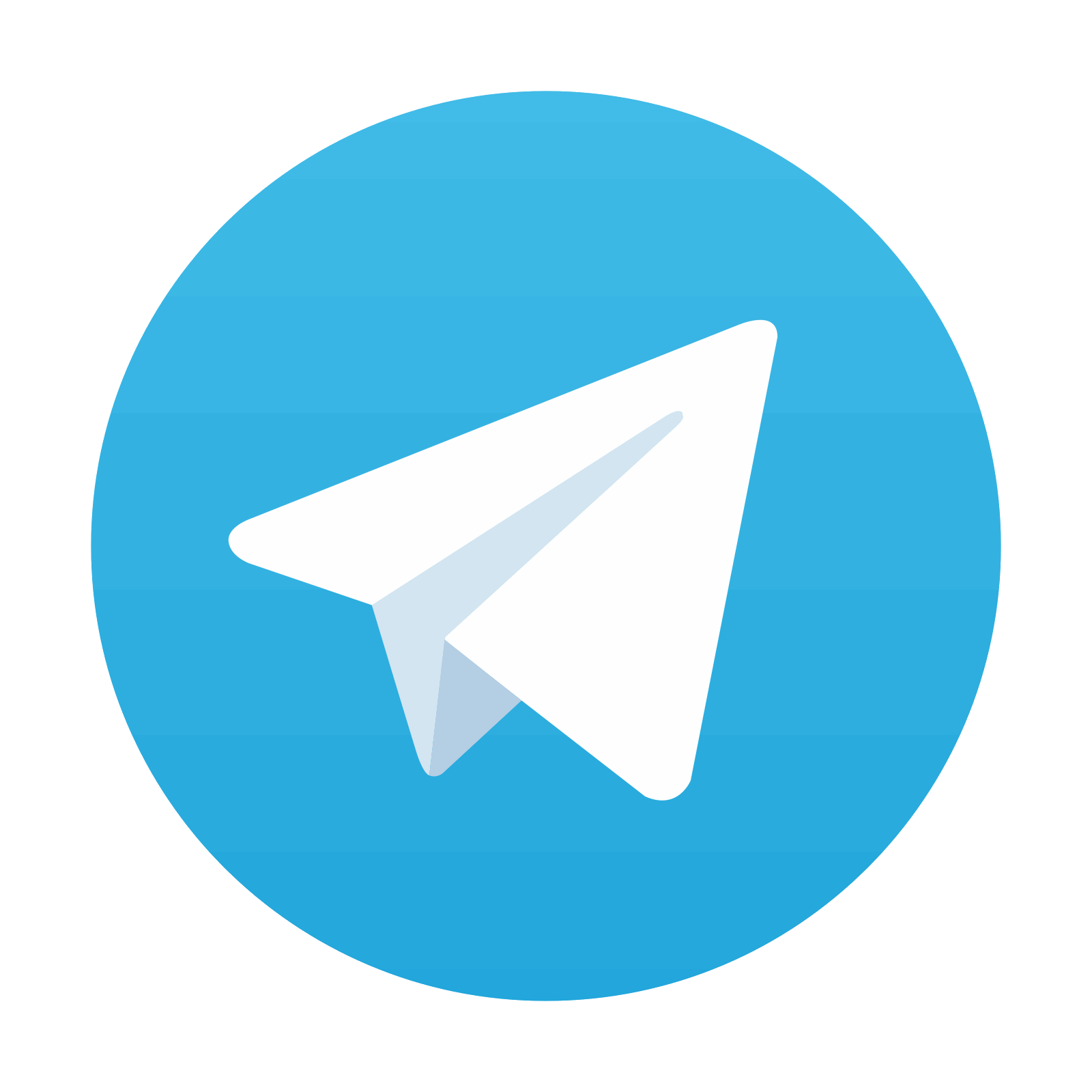
Stay updated, free articles. Join our Telegram channel

Full access? Get Clinical Tree
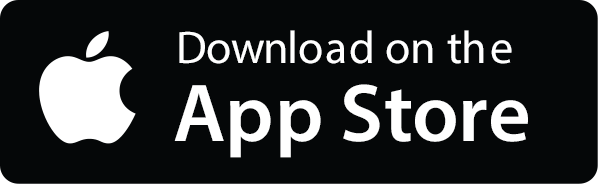
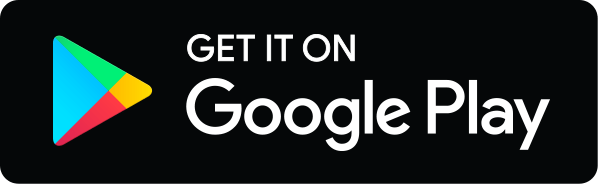