Behavioral disordersa
Cognitive disordersa
Indicated by reliable informant using validated inventory
Shown by validated performance index on normalized tests
Significant changes compared to premorbid behavior
Not attributable to developmental disorder
Significant changes in activities of daily living, social life, or work
Highly suggestive
Global hypoactivity with apathy and/or abulia
Response inhibition (e.g., Stroop test)
Global hyperactivity with distractibility and/or psychomotor instability and/or disinhibition
Rule deduction and generation (e.g., Card sorting test)
Shifting of sets (e.g., perseveration)
Stereotyped and perseverative behavior
Information generation and strategic search (e.g., fluency tasks)
Supportive deficits and developing areas
Disorders of emotional control (euphoria, emotional lability)
Planning (e.g., Tower of London test)
Disorders of social behavior including loss of empathy
Coordination of dual tasks (e.g., dual task)
Disorders of sexual, eating, and urinary behavior
Action initiation and sustained alertness (e.g., Simple Reaction Time test)
Spontaneous confabulations, reduplicative paramnesia
Episodic memory strategic processes (retrieval and memory selection)
Anosognosia, anosodiaphoria
Theory of mind and metacognitive processes
Initiation has long been regarded as the rapidity to initiate action and is frequently confounded with action speed. Impairment of action speed is a leading deficit in stroke patients [11–13] and may be observed in the long term [14, 15]. It is frequently labeled “psychomotor slowing.” However, the term “action slowing” appears to be more appropriate, as it has been shown to involve, to varying degrees, perceptual, motor, and cognitive aspects of action speed [16–18]. In addition, these results indicate that action slowing cannot be attributed solely to an initiation deficit, as it may also be due to perceptual or motor impairment. Initiation of action is especially involved in situations requiring repeated activation of a sequence of simple actions. This function is typically assessed by Reaction Time tests. Following experimental validation, Reaction Time [19] and benefits of a warning cue [20, 21] are now used as an index of initiation processes. This method shows that initiation depends on the activation of a thalamo-parieto-prefronto-cingulate network predominantly in the right hemisphere. Lesion studies indicate that the mediofrontal region is especially critical for initiation [17, 20]. These converging results indicate that initiation can be differentiated from action speed and other executive processes [20, 22]. In clinical practice, action speed is usually assessed by the simple condition of chronometric tests (such as part A of the Trail Making Test and naming and reading Stroop subtests) although this does not provide a pure index of initiation (Table 8.1).
The use of a comprehensive cognitive battery and behavioral inventory shows that impairment (usually mild) may be observed in one test or one behavioral domain in normal subjects. For this reason, it is essential to adjust criteria of impairment according to the number of performance indices. In the GREFEX study, this adjustment was performed by differentiating dysexecutive disorder (i.e., impairment in one test or one behavioral domain) from dysexecutive syndrome, which requires the association of several impairments on the behavioral inventory (behavioral dysexecutive syndrome) or tests (cognitive dysexecutive syndrome). The usefulness of this approach is supported by the demonstration of a relationship between dysexecutive impairment and loss of autonomy in dysexecutive syndrome [5].
Disorders of Executive Functions in Stroke
The present review will focus on studies of consecutive patients (hospital- or population-based studies) using a validated battery of tests assessing executive functions and reporting data with sufficient details to determine the profile of cognitive impairment. Published studies provide a consistent picture of the high frequency of executive deficit across various types of stroke with about 35 % of patients suffering from action slowing and about 20 % of patients suffering from a deficit of rule deduction, abstract reasoning, or set shifting. Action slowing is assessed by various tests, such as the Digit-Symbol substitution subtest, Trail Making Test, naming and reading Stroop subtests, and more rarely, Reaction Time tests. Executive deficits are reported with frequencies ranging from approximately 10 to 50 % [11, 23–30]. On tests assessing abstract reasoning, such as the similarities subtest of the revised Wechsler Adult Intelligence Scale or Modified Card Sorting Test, a deficit was observed in 15–30 % of patients [11, 23, 27]. Verbal fluency tests are reported to be impaired in 15–40 % of patients [11, 24, 25]. A study that used a global score of executive functions summarizing performance on verbal fluency, Wisconsin Card Sorting, Stroop interference tests, and Part B of the Trail Making Test reported a deficit in 41 % of patients [31]. These high prevalences of dysfunction tend to decrease on retesting performed 6 months to 4 years post-stroke, suggesting that the deficits improve in about 25–30 % of patients [25, 27]. However, a deficit in executive functions, motor speed, and episodic memory is still frequently observed in studies with long-term stroke survivors [14, 15], indicating that a large percentage of patients still suffer from attentional and executive disorders in the long term. The presence of dysexecutive disorders was associated, in several studies, with a poor functional outcome [13, 15, 27, 31, 32]. This has been shown for both behavioral [33] and cognitive disorders [5] (Table 8.2).
Table 8.2
Summary of findings concerning cognitive impairment after stroke
References | #Pts | Type of stroke | Time of assessment post-admission | Impairment in | % Impaired |
---|---|---|---|---|---|
[11] | 227 | Ischemic | 3 Months | Abstract reasoning | 16–20 |
Attention | 20–39 | ||||
Language | 13–33 | ||||
Memory | 10–25 | ||||
Visuospatial | 17–25 | ||||
[15] | 307 | Ischemic and hemorrhagic | 5 Years | Executive function | 33 |
Information processing | 33 | ||||
Language | 24 | ||||
Verbal memory | 8 | ||||
Visual memory | 9 | ||||
Visuospatial | 3 | ||||
[23] | 37 | Hemorrhagic | 6 Months | Executive function | 19 |
Memory | 14 | ||||
[24] | 150 | Unspecified | >3 Months | Memory | 26 |
Numerical working memory | 43–47 | ||||
Spatial working memory | 14–21 | ||||
[25] | 196 | Ischemic and hemorrhagic | 1 Month | Attention | 32 |
Executive function | 32 | ||||
Information processing | 60 | ||||
Language | 35 | ||||
Memory | 24 | ||||
Orientation | 10 | ||||
Visuospatial | 37 | ||||
[26] | 47 | Unspecified | <10 Days | Executive function | 61 |
[27] | 111 | Ischemic and hemorrhagic | <3 Weeks | Abstract reasoning | 24 |
Executive function | 30 | ||||
Language | 21 | ||||
Verbal memory | 21 | ||||
Visual memory | 16 | ||||
Visuospatial | 31 | ||||
[28] | 74 | Hemorrhagic | 20 Months | Episodic memory | 42 |
Executive function | 14 | ||||
Language | 5 | ||||
Verbal memory | 36 | ||||
Visual memory | 19 | ||||
Visuospatial | 5 | ||||
Working memory | 5 | ||||
[29] | 55 | Ischemic and hemorrhagic | <3 Weeks | Executive function | 46 |
Language | 18 | ||||
Long-term memory | 26 | ||||
Visuospatial | 27 | ||||
[30] | 48 | Hemorrhagic | 40 Months | Episodic memory | 52 |
Executive function | 37 | ||||
Language | 35 | ||||
Visuospatial | 19 | ||||
[31] | 256 | Ischemic | 3 Months | Executive function | 41 |
Profile of Executive Deficits
Studies including consecutive patients usually have different test batteries and usually do not assess behavioral changes, and thus preclude a precise description for the profile of dysexecutive disorders. In addition, most studies report crude test performance results that fail to distinguish between deficits due to true executive disorders or to associated cognitive impairment (e.g., fluency test impairment may be due to language or executive disorders). The GREFEX study assessed 152 stroke patients (infarct: n = 47; complicated ruptured intracranial aneurysm: n = 76; cerebral venous thrombosis: n = 11) referred for cognitive assessment. This study design is not appropriate to determine the prevalence of a deficit, but is useful to assess the relative frequencies of certain disorders. Behavioral and cognitive dysexecutive syndromes were observed in 25 % and 29 % of patients, respectively [5], indicating that the failure to systematically assess behavioral changes results in an underestimation of dysexecutive disorders. The prominent behavioral changes were hypoactivity with apathy (observed in 20 % of patients) and anosognosia. Apathy without depression has been observed with a frequency ranging between 20 and 30 % in stroke patients [33, 34]. The prominent cognitive disorders involved information generation (i.e., fluency tests), rule deduction (i.e., number of categories achieved on the Modified Card Sorting Test and correct responses on the Brixton Test), and shifting (i.e., perseveration on Trail Making Test B and the Modified Card Sorting Test) [5]. The specific dysexecutive impairment profile has not yet been defined according to stroke type.
Determinants of Dysexecutive Disorders
Stroke location is usually considered to be the main determinant of the type of cognitive disorder. Executive functions are mainly supported by the frontal lobes, basal ganglia (especially the caudate nucleus, pallidum, anterior and median thalamus), and their connections (especially the anterior centrum semiovale, anterior limb, and genu of the internal capsule). Executive disorders are therefore essentially expected in strokes affecting these structures. This classical view has been challenged by many studies. Firstly, several studies have shown that anterior and posterior strokes result in impaired performance on tests of executive functions [12, 35]. This probably accounts for the contrast between the high prevalence of dysexecutive impairment and the lower frequency of anterior stroke. Secondly, stroke location is not the sole determinant of dysexecutive disorders; white matter abnormalities [36–38], microbleeds [39], medial temporal atrophy [40], and pre-stroke cognitive disorders [41] also contribute to post-stroke executive impairments. White matter abnormalities are frequently correlated with executive test performance, especially on tests with time constraint and chronometric performance [42]. Accordingly, it has been demonstrated that the volume of white matter abnormalities is related to action slowing [43]. Cerebral microbleeds have been associated with deficits on executive tests and action speed [39, 44, 45] independent of white matter abnormalities [39, 44]. Additionally, patients with impaired executive tests had a greater number of microbleeds in frontal and basal ganglia regions [39, 44]. Pre-stroke cognitive deficits are more frequent in patients after the age of 75 [41]. They may be caused by previous strokes or by nonvascular lesions, especially Alzheimer’s disease [41]. Preexisting dementia has been observed in patients with spontaneous cerebral hemorrhage with a prevalence similar to that reported in ischemic stroke patients [46]. It may be caused by previous vascular lesions (especially in subcortical hemorrhages) or by Alzheimer’s disease (especially in lobar hemorrhages) [46].
Dysexecutive Disorders According to Stroke Type and Location
The high frequency of executive disorders relative to other cognitive deficits seems to concern all types of stroke, as it has been reported in ischemic [11, 13, 24, 27, 31], hemorrhagic [30], and mixed ischemic-hemorrhagic strokes [15, 25, 29]. The majority of studies determining the prevalence of dysexecutive disorders were performed by focusing on the most frequent stroke type (cerebral infarction). They showed that action slowing is observed in about one-third of patients, and a cognitive dysexecutive deficit is observed in about one-fifth of patients. The profile of dysexecutive disorders has been assessed in patients with various infarct types and locations.
Infarcts involving the frontal lobes, basal ganglia, and their connections are mainly due to occlusions of the anterior/superior branch of the middle cerebral artery and/or the deep perforating branches (lateral lenticulostriate arteries). Infarcts in the territory of the anterior cerebral artery are rare (accounting for 2–5 % of infarcts), but they can cause frontal (medial part) and deep (ventral part of caudate nucleus) cerebral infarctions. Finally, infarcts of the anterior part of the thalamus are mainly located in the territory of the tuberothalamic artery, a branch of the posterior communicating artery, and the anterior choroidal artery. Infarcts of the middle part of the thalamus are in the territory of the paramedian thalamic-subthalamic arteries, which originate from the first segment of the posterior cerebral artery.
Infarcts in the territory of the anterior/superior branch of the middle cerebral artery usually induce cognitive dysexecutive disorders that are reported to be especially marked on rule deduction, shifting of rules, information generation, and maintenance of information in working memory. Conversely, behavioral dysexecutive changes are usually reported to be moderate and can even be absent. Global hypoactivity with abulia-apathy is the most frequent disorder. Dysexecutive disorders are usually associated with other cognitive deficits (aphasia in left-sided infarct and visuo-constructional disorders in right-sided infarct—see Chaps. 5 and 6 for more information). Pure unilateral lenticulostriate infarcts may induce dysexecutive disorders, although the deficits are usually mild. In the behavioral domain, global hypoactivity with abulia-apathy or hyperactivity with distractibility-impulsivity may be observed [47–49]. Cognitive executive deficits concern tests that assess response activation and suppression, information generation, and rule deduction [49, 50]. They are usually associated with memory disturbances [10, 51, 52] and, in left-sided infarcts, language disorders [10, 50, 53]. Bilateral striatal lesions may be observed in anoxia, which usually also causes lesions outside the striatal region. Such a condition may induce “loss of psychic autoactivation” [54] characterized by severe global hypoactivity with abulia-apathy (usually associated with “empty mind”), which is reversed by heteroactivation (usually following instructions from the examiner or the caregiver). This disorder has also been reported after bilateral thalamic infarction [55].
In anterior cerebral artery infarcts, the profile of dysexecutive disorders has been only partly documented. Akinetic mutism (which is an extreme form of global hypoactivity with apathy) may be as a result of bilateral infarcts followed by severe dysexecutive disorders persisting after the acute phase [56, 57]. Transient akinetic mutism and acute confusional states have been reported in patients following left-sided infarcts [56–58]. Acute action slowing has also been reported in patients after right-sided infarcts [56–58].
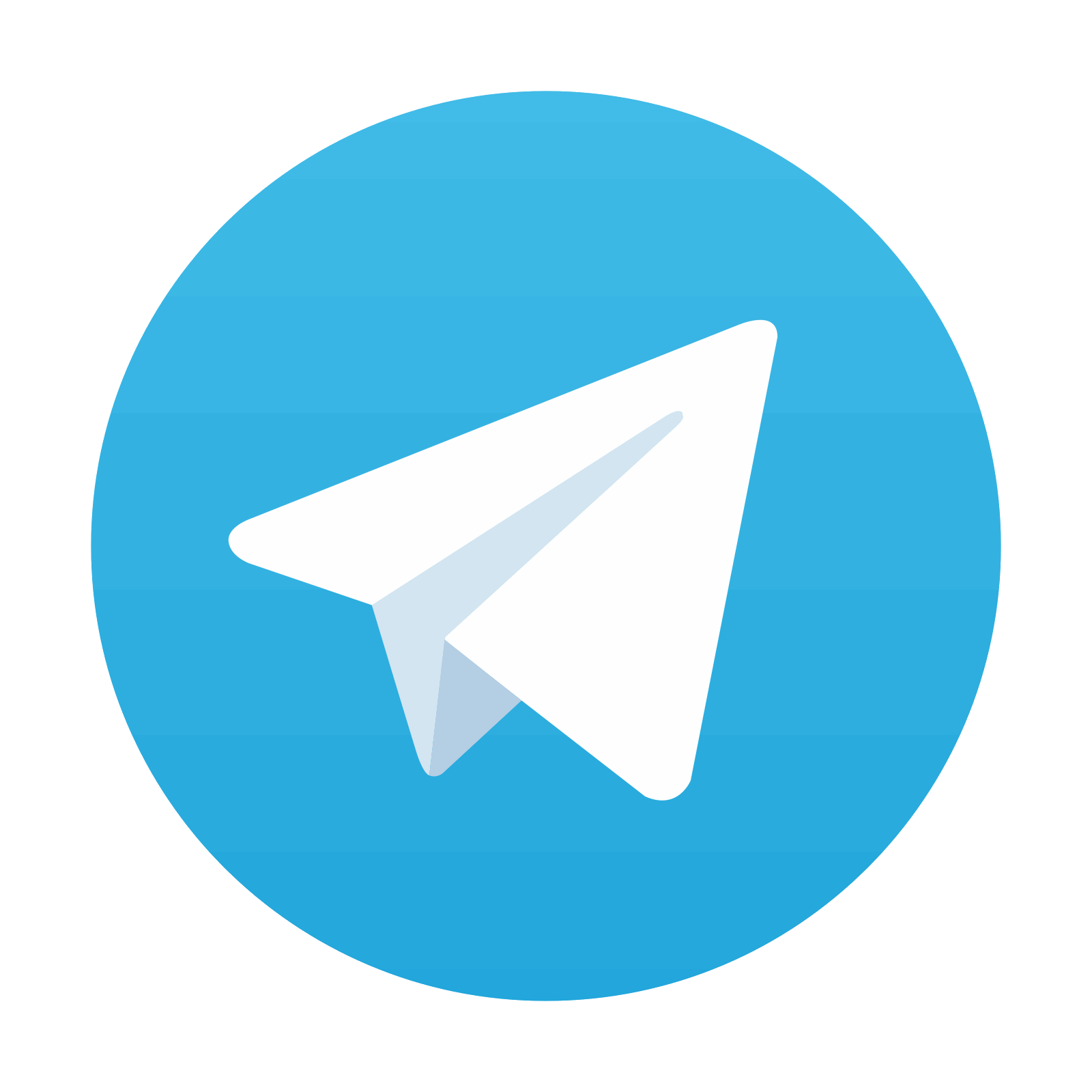
Stay updated, free articles. Join our Telegram channel

Full access? Get Clinical Tree
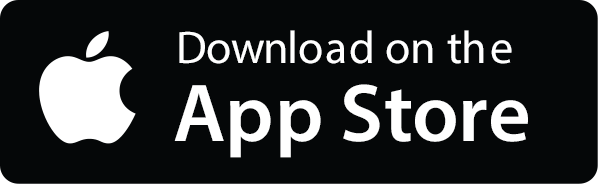
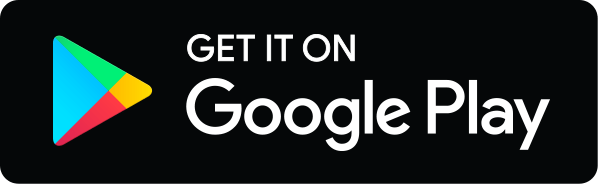