Drugs Used in Renal Disease
DRUG CONSIDERATIONS IN PATIENTS WITH RENAL DYSFUNCTION
Influence of Drugs on Renal Function
All anaesthetic agents may cause a generalized depression of renal function which is transient and clinically insignificant. However, nephrotoxic drugs can impair renal function permanently. For example, they may lead to severe sodium and water depletion, reduction in renal blood supply, direct renal damage or renal obstruction (Table 10.1). Some drugs impair renal function by more than one mechanism.
TABLE 10.1
Mechanisms of Drug-Induced Renal Damage
Sodium and water depletion
Reduced renal perfusion
Direct renal toxicity
Urinary obstruction
VASOACTIVE DRUGS USED IN RENAL DYSFUNCTION
Side-Effects
A decrease in splanchnic oxygen consumption in septic patients despite an increase in splanchnic blood flow
A possible decrease in gastric motility compromising gastric feeding and absorption
Impairment of regional ventilation-perfusion matching of the lung and the ventilatory drive in response to hypoxaemia and hypercapnia
Reduced output of anterior pituitary hormones including prolactin, growth hormone, TSH and thyroid hormones
Ideally, dopamine should be administered through a central venous catheter because extravasation may cause sloughing and necrosis of local surrounding tissues.
Adenosine
Adenosine also slows the heart rate and impairs atrioventricular conduction and is used in the treatment of supraventricular tachycardias (Ch 47).
Calcium Channel Blockers
Calcium channel blockers (CCBs) (Ch 8) are predominantly used in the treatment of hypertension. They act selectively on calcium channels in the cellular membrane of cardiac and vascular smooth muscle (VSM) cells. Free calcium within the VSM enhances vascular tone and contributes to vasoconstriction. CCBs reduce the transmembrane calcium influx in VSM cells, causing vasodilatation. In addition, CCBs have a direct diuretic effect that contributes to their long-term antihypertensive action. Nifedipine increases the urine volume and sodium excretion, and may inhibit aldosterone release. This diuretic action is independent of any change in renal blood flow or GFR.
Noradrenaline/Adrenaline/Phenylephrine
Although noradrenaline and adrenaline also have β-receptor effects, all three of these drugs are very potent vasoconstrictors acting on the vascular α-receptors (Ch 8). Their use is often accompanied by fear of inducing decreases in renal blood flow, GFR and renal function.
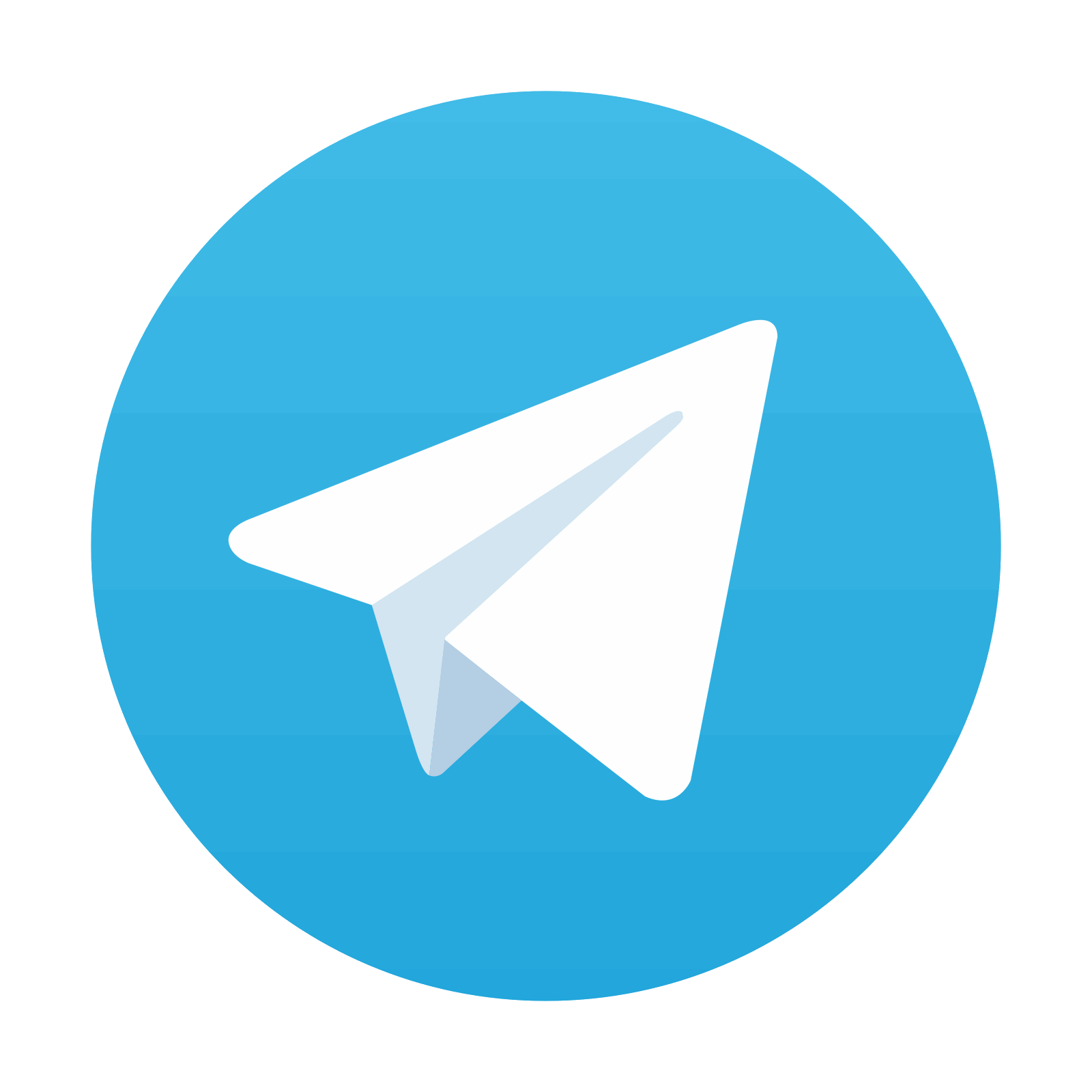
Stay updated, free articles. Join our Telegram channel

Full access? Get Clinical Tree
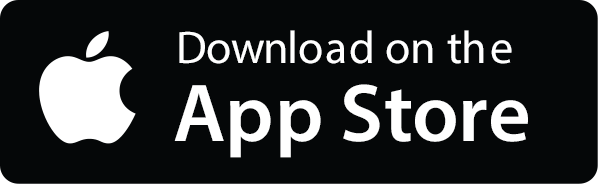
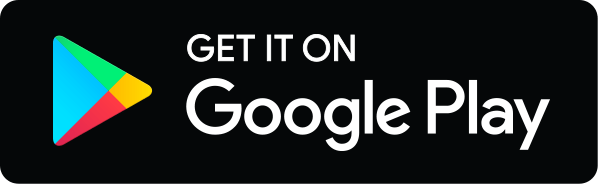